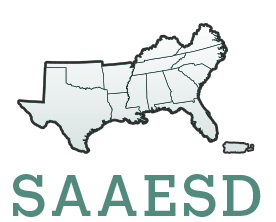
S1023: Enhancing production and reproductive performance of heat-stressed dairy cattle.
(Multistate Research Project)
Status: Inactive/Terminating
S1023: Enhancing production and reproductive performance of heat-stressed dairy cattle.
Duration: 10/01/2005 to 09/30/2008
Administrative Advisor(s):
NIFA Reps:
Non-Technical Summary
Statement of Issues and Justification
Project's Primary Website is at http://rps.uvi.edu/AES/S299/S299_home_page.html (direct link can be found under LINKS)
Throughout the Southeast United States and in many subtropical and tropical regions dairy cattle are subject to elevated ambient temperature and/or high relative humidity for extended periods. In the Southeast US, ambient conditions frequently exceed the thermoneutral range and cause heat stress for 4 to 6 months of each year. In lactating dairy cows, heat stress reduces feed intake and elevates body temperatures, which are associated with reduced milk yield and reproductive performance. Moreover, genetic selection for milk yield has reduced the dairy cows' ability to regulate body temperature, which exacerbates the effects of heat stress. Environmental modifications to mitigate the impact of heat stress have been developed, but a recent analysis of Dairy Herd Improvement (DHI) records suggest that further research is needed; dairy herds in the Southeast US have lower milk yields, greater days open and higher somatic cell counts than those further north. To minimize the impact of heat stress on southeastern dairy producers, a thorough understanding of the effects of environmental, dietary and genetic modifications is necessary. In addition, a more complete understanding of the cellular and physiological mechanisms by which cows regulate body temperature will allow the development of new strategies for improving milk production,reproduction and embryonic survival during periods of heat stress.
Dairy cattle in the Southern region are annually exposed to prolonged periods of elevated humidity and heat which reduce feed intake, milk production and reproductive performance. Development and testing of effective management strategies to mitigate the negative effects of heat stress requires a coordinated research effort, which includes fundamental and applied research in
nutrition and reproduction. This is best accomplished through a regional research project that includes shared resources and scientific expertise. There are RRF projects in the Southern region focused in whole or in part on enhancing production and reproductive performance of swine (S-288), poultry (S-1020; S-292) and beef cattle (S-284), but no similar regional effort focused on
dairy cattle. Two related regional projects in the Western region focus on heat stress: W-112, Reproductive Performance in Domestic Ruminants; and W-173, Stress Factors of Farm Animals and Their Effects on Performance. One potential outcome of W-173 is to develop more cost effective techniques for reducing heat stress. Yet, neither of these projects focus on nutrition and reproduction interactions in heat-stressed dairy cattle. The intent of the current proposal is to
complement, not duplicate, objectives of the two existing projects. Methodologies effective in reducing heat stress will be incorporated into the project and expanded to examine the potential of nutritional modification and hormonal therapy on subsequent production and reproduction. This project proposal fits within the region's priorities as described in the Southern Strategic Research Plan. The emphasis is on inter-disciplinary research to enhance efficiency and sustainability of an animal system in our region's unique climate.
Related, Current and Previous Work
Introduction
Elevated ambient temperature, humidity and radiant energy compromise the ability of the lactating
dairy cow to dissipate heat, and coupled with metabolic heat, make it difficult for lactating dairy
cows to maintain thermal balance. Elevated body temperatures initiate adaptive/compensatory
responses to reestablish thermal balance. Stott (1981) stated "these re-adjustments to maintain
homeostasis by homeostatic mechanisms are referred to as adaptations and may be favorable or
unfavorable to the economic interests of humans. Nevertheless, they are essential for survival of the
animal."
The impact of heat stress on livestock is broad in geographic terms. Dairy cattle across the
Southeastern United States and in many subtropical and tropical regions are subject to high ambient
temperature and/or high relative humidity for extended periods. In the Southeast, conditions of heat
stress persist for 4 to 6 mo of each year. Several trends will make heat stress an even greater
dilemma in the future. Heat stress itself may increase in magnitude if continued global warming
occurs (Hulme, 1997). Secondly, the world's population is growing faster in tropical and subtropical
regions of the world than in temperate regions (Roush, 1994) and it is reasonable to assume that a
greater proportion of food animals will live in hot, humid climates than at present. Finally, changes
in the genetics and physiology of food animals for increased production are making these animals
less able to regulate body temperature (i.e., less adapted to warm environments). This is especially
true for dairy cattle, where selection for milk yield reduces thermoregulatory ability in the face of
heat stress (Berman et al., 1985; Ravagnolo & Misztal, 2000) and magnifies the seasonal depression
in fertility caused by heat stress (Al- Katanani et al., 1998). Moreover, adaptation is reduced when
native, genetically-adapted dairy cattle in tropical and semitropical regions are replaced by higher
producing, non-adapted breeds. Also, the use of bovine somatotropin (GH) can exacerbate heat
stress (West et al., 1991; Elvinger et al., 1992; Cole and Hansen, 1993).
Beede and Collier (1986) identified three strategies to minimize the effects of heat stress in
lactating dairy cows: modification of the environment, genetic development of heat tolerant breeds
and improved nutritional management. Nutritional modifications that minimize hyperthermia during
heat stress have been developed, but the response to dietary changes may be improved by
environmental modifications. Nutrient excesses also contribute to inefficient energy and protein
utilization, and further exacerbate thermal stress. Thus, a thorough understanding of dietary
modifications on ruminal fermentation and cellular metabolism is necessary to minimize heat stress.
Reproductive performance of dairy cattle can be estimated as the herd pregnancy rate (Hansen
and Aréchiga, 1999), which is a product of the estrus detection rate and the pregnancy rate per
insemination. Heat stress is a major limitation to reproductive function of dairy cows because it can
have deleterious effects on both components of the herd pregnancy rate. Historically, environmental
modification has been used to mitigate the effects of heat stress. Further research in this area is
critical because existing housing systems cannot eliminate the seasonal depression in fertility
(Hansen and Aréchiga, 1999). In addition, it may be possible to develop new strategies for
improving reproduction during heat stress by regulating the physiology of the cow or the cell
biology of tissues involved in reproduction so as to prevent or counteract actions of heat stress.
These approaches will depend upon greater knowledge of the cellular and physiological mechanisms
by which heat stress leads to decreased estrous activity and embryonic survival as well as a better
understanding of the processes by which cows regulate body temperature.
Influence of Heat Stress on the Physiological Responses of the Cow
Reactions of cattle and other homeotherms to moderate climatic changes are compensatory and
are directed at maintaining or restoring thermal balance (Kibler and Brody, 1953). Numerous
physiologic mechanisms for coping with heat stress have been described, including sweating,
panting and vasodilation with increased blood flow to the skin surface (Blackshaw and Blackshaw,
1994). In addition, metabolic rate is reduced, dry matter intake (DMI) and nutrient intake are
reduced, and water metabolism is altered in response to heat stress. Unfortunately, responses to heat
stress often have negative effects on the physiology of the cow. One example is that while DMI
declines as temperatures exceed 25 oC, the maintenance energy expenditure actually increase.
The term heat stress is often used loosely in reference to stressful climatic conditions, yet the
contribution of individual environmental parameters to the severity of heat stress are poorly defined.
Kaguga and Sarpong (1991) reported mean daily temperature had the greatest effect on milk yield
and rectal temperature. Moore et al. (1992) reported nighttime minimum temperature was a critical
factor in maintaining milk production. Others have reported a lag effect, in which the influence of
elevated temperatures has a greater impact 2-3 days later. Yet, it is clear that elevated humidity
exacerbates conditions of heat stress and the temperature-humidity index (THI) may describe more
precisely the effects of the environment upon the cow's ability to dissipate heat (Ravagnolo et al.
2000). Milk yield and TDN intake declined slightly when the THI exceeded 72 and then sharply
above an index of 76 (Johnson et al., 1963). For each unit of increase in THI above 72, West et al
(2000) observed a 0.32 kg drop in DMI and a 0.91 kg drop in milk yield. Holter et al. (1996)
reported that the minimum daily THI was more closely correlated with DMI than maximum THI in
Jerseys. Reductions in DMI of up to 4.4 kg/d or 22%, began when the minimum THI exceeded 56-
57 and continued until THI reached 72. Similarly, correlation of DMI with minimum and maximum
THI for Holsteins was -0.63 and -0.62 (Holter et al, 1997).
Effects of Cooling on Intake and Milk Yield
Environmental modification is necessary to maintain productivity of lactating dairy cows.
Maintenance of DMI is critical to production and minimizing the increase in body temperature
during hot conditions translates directly into greater feed consumption. Shade is the most obvious
environmental modification. In a hot, humid climate shade alone can drop rectal temperatures 2 to
4.1%, respiratory rates by 29 to 60%, improve DMI by 6.8 to 23.2%, and improve milk yield by 9.4
to 22.7% (Mallonee et al., 1985; Schneider et al., 1986; Schneider et al., 1984). Cows shaded
during the dry period yielded 4.5 and 13.6% more milk at 100 and 305 d postpartum and delivered
calves weighing 3.1 kg more compared with cows receiving no shade, even though cows were
handled similarly postpartum (Collier et al., 1982a; Wolfenson et al., 1988b). Additional benefits
from cooling cattle with fans and sprinklers can be derived in hot or hot and humid climates.
Reports as early as the 1940's (Seath and Miller, 1948) demonstrated that fans and sprinklers work
synergistically to reduce rectal temperatures and respiratory rates. Combining sprinklers and fans
also reduces the diurnal variation of body temperature. Williams et al. (2004) observed reduced
rectal temperatures and respiration rates in response to tunnel ventilation cooling in the southeast.
Much of the response to cooling results directly from increased DMI (Bucklin et al. 1991), yet
the physiologic benefits of cooling included greater circulating GH and lower prolactin
concentrations (Igono et al., 1987). With these physiologic changes were a 2.3 and 2.0 kg/d increase
in DMI and milk yield. Similar to shade research with dry cows (Collier et al., 1982b), cooling with
fans and sprinklers during the dry period increased mean 150 d milk yield by 3.5 kg/d, and the effect
was greater in older cows (Wolfenson et al., 1988b). Milk yield was 0.9, 2.5, and 7.3 kg per day
greater in lactation 2, 3, and 4+ for cows which were cooled during the dry period. It is apparent
that with increasing production, body size and maturity, cows become more sensitive to the effects
of heat stress. Cooling of dry cows increases milk yield through 120 d of lactation and the increase
was greater as daily temperatures rose (Armstrong, 1994).
Nutrition Modifications to Mitigate the Impact of Heat Stress
The six-week period around parturition (transition) is a time of stress for dairy cows. Feed
intake declines by 25 to 30% during the final week prepartum (Bertics et al., 1992). Unfortunately,
nutrient demands for the placenta and fetus are greatest during this period (Bell, 1995) and the
current nutritional guidelines do not address this decline (NRC, 2001). Reasons for reduced
prepartum DMI include hormonal (progesterone and estrogen) fluctuations (Grummer, 1995),
repositioning of the fetus and increasing non-esterified fatty acids (NEFA) in blood. In addition, the
number of cows experiencing postpartum metabolic disorders often exceeds 50% and in these cows
the drop in DMI can be even more greater (Zamet et al., 1979). Heat stress exacerbates the stress of
transition, further depressing intake and reducing the probability of a smooth transition. Frequently
dry cows receive little shade and no supplemental cooling, yet, it is becoming increasingly apparent
that cooling both pre- and post-partum may return large benefits in the form of healthier cows with
less body condition loss due to the greater DMI.
Extensive research in heat abatement, nutritional management and their interaction has been
conducted in lactating dairy cows. To compensate for the lower DMI and maintain milk yield in
heat-stressed cows, producers often increase the dietary nutrient density, but the results are not
always favorable. The challenge for producers is to increase ration density, while meeting the
minimum fiber needed to maintain rumination and rumen health. West (1999) recently reviewed
studies on fiber feeding during hot weather and concluded that, low fiber diets may reduce the heat
load, but the level of DMI has a much greater role in determining metabolic heat production.
Further studies on the role of fiber quality, heat increment and production during hot weather are
required. Feeding protein, especially rumen-degradable protein, in excess of NRC recommendations
can decrease DMI and milk yield in heat-stressed cows (Huber et al., 1994; Arieli et al., 2004). The
drop in milk yield is greater than the reduction in energy intake because of the energy required to
convert the excess protein into urea for excretion. Improving protein quality supports higher milk
yields in heat-stressed cows (Huber et al., 1994). Cows fed diets containing higher concentrations of
lysine (from soybean, fish or blood meals) produced 11% more milk under heat stress conditions
(Chen et al. 1993).
Because fats have a high energy density and a low heat increment, their addition to rations
should benefit heat-stressed cows. Huber et al. (1994) found adding fats to ruminant diets reduced
heat production (above maintenance) up to 9.7% under thermoneutral conditions. Yet, in under
conditions of heat stress, rectal temperatures are generally unaltered by added fats. Furthermore,
while milk fat is often increased in response to added fats, the production response is variable; milk
yield increased (Chan et al., 1992; Huber et al., 1993) or remained constant (Chan et al., 1993;
Chang et al., 1977), suggesting the response to supplemental fat is diminished in heat stress cows.
The variable results may originate with differences in rumen available carbohydrates and fiber in
rations with added fats and some fats can depress DMI (Palmquist, 1984). Additional studies are
needed to define the potential of added fats for heat-stress cows and the conditions that maximize
their benefit. Addition of fungal cultures has been reported to increase milk yield by approximately
4% and decrease rectal temperatures and respiratory rates (Huber, 1994), but the results have not
been consistent.
Influence of Heat Stress on the Estrous Cycle and Endocrine Regulation of Reproduction
Heat stress in dairy cattle reduces estrus length (Monty and Wolff, 1974; Abilay et al., 1975) and
intensity (Gangwar et al., 1965; Nebel et al., 1977). Heat stress-induced changes in estrus activity
reduce the likelihood that estrus will be detected by dairy personnel (Thatcher and Collier, 1986).
The causes of reduced expression during heat stress have not been completely defined, although may
involve some aspects of the hypothalamic-pituitary- adrenal axis (i.e., the CRH-ACTH- cortisol
cascade interacting with the reproductive system). Heat stress can increase cortisol secretion
(Roman-Ponce et al., 1981; Wise et al., 1988a; Elvinger et al., 1992) and ACTH has been reported
to block estradiol-induced sexual behavior (Hein and Allrich, 1992). However, in some studies
heat-stress induced elevations in circulating cortisol were transitory (Christison and Johnson, 1972;
Miller and Alliston, 1974; Elvinger et al., 1992), non-existent (Wise et al., 1988b, West et al., 1991)
or heat stress depressed cortisol concentrations (Abilay et al., 1975). Accordingly, the effects of heat
stress on estrus behavior likely include other actions independent of the pituitary-adrenal axis.
Some reports indicate that heat stress causes a reduction in peripheral concentrations of
estradiol-17B (E2) at estrus (Gwazdauskas et al., 1981; Wilson et al., 1998a) although this effect has
not always been observed (Rosenberg et al., 1982). It is possible that the major reason that heat
stress reduces the expression of estrus is because of the physical lethargy produced by heat stress.
Direct action of elevated maternal temperature on the function of reproductive tract tissues
remains a possibility although exposure of cultured endometrial explants to 43 oC caused only small
changes in protein and DNA synthesis (Putney et al., 1988b; Malayer et al., 1988; Malayer and
Hansen, 1990). However, heat shock of 42 and 43 oC increased output of prostaglandins by cultured
endometrium collected at day 17 of the estrous cycle (Putney et al., 1988b; Malayer et al., 1990) and
exposure of cultured day 17 conceptuses to 43 oC reduced secretion of interferon-t (Putney et al.,
1988b). Furthermore, heat stress on day 17 of pregnancy increased uterine production of PG-F2alpha
in response to oxytocin (Wolfenson et al., 1993). Heat stress can also redistribute blood flow from
the visceral organs to the periphery; the decreased perfusion could compromise endometrial and
oviductal function. Roman-Ponce et al. (1978) observed that the increase in uterine blood flow
caused by injection of E2 was greater in cows receiving summer shade than in those without.
Increased synthesis of heat shock proteins might also alter assembly, transport or binding activity of
steroid receptors. Indeed, heat shock blocked E2 induced vitellogenin transcription in Xenopus
hepatocytes (Wolffe et al., 1984). Recent reports suggest that heat stress can cause an increase
peripheral E2 levels between days 1-4 of the estrous cycle (Wolfenson et al., 1995) and a reduction
from days 4-8 (Wolfenson et al., 1995) and 11-21 (Wilson et al., 1998ab); but, earlier reports
suggested heat stress had no effect on circulating estrogens (Roman-Ponce et al., 1981; Wise et al.,
1988ab). Similarly, heat stress has been reported to increase (Abilay et al., 1975; Roman-Ponce et
al., 1981; Trout et al., 1998), decrease (Younas et al., 1993; Howell et al., 1993; Ronchi et al., 2001;
Wolfenson et al., 2002) or have no effect (Wise et al., 1988a; Wolfenson et al., 1995; Wilson et al.,
1998ab) on peripheral progesterone (P4) concentrations during the luteal phase. Some of the
variation in hormonal responses to heat stress probably reflects the fact that ovarian steroid
concentrations depend not only on rate of secretion from ovarian tissue, but also on rate of vascular
perfusion of the ovary, possible adrenal release (at least for P4), metabolic degradation, and the
degree of hemodilution or hemoconcentration (Richards, 1985; McGuire et al., 1989; Johnson et al.,
1991; Elvinger et al., 1992). In addition to steroid hormones, heat stress has also been shown to
decrease concentrations of LH (Gilad et al., 1993) and inhibin (Roth et al., 2000).
Heat-Stress Induced Alterations in Ovarian Follicular Dynamics
The ovulating follicle emerges as an antral follicle about 40 d before ovulation (Lussier et al.,
1987). Thus, heat stress during follicular growth has the potential to compromise the oocyte, either
by the direct actions on the oocyte or by altering the follicular function. Badinga et al. (1993)
observed that heat stress beginning on the day of ovulation reduced the diameter and volume of the
dominant follicle on day 8 of the estrous cycle. Heat stress from day 3-5 of the estrous cycle
increased androstenedione and reduced E2 levels in follicular fluid of the dominant follicle at day 7
(Wolfenson et al., 1997). In another study (Wolfenson et al., 1995), heat stress at day 1 of the
estrous cycle caused an increase in number of follicles > 10 mm in diameter, earlier emergence of
the dominant follicle in the second follicular wave and tended to reduce plasma inhibin levels.
Initiation of heat stress at day 11 of the estrous cycle caused more estrous cycles characterized by
three follicular waves versus two, reduced E2 levels in blood and an extended estrous cycle (Wilson
et al., 1998ab). The increase in estrous cycle length was ascribed to reduced estrogenic support for
the uterine luteolytic mechanism. Yet, Trout et al. (1989) found no extension of estrous cycle length
by heat stress from day 11. Changes in follicular function in response to heat stress could result
from endocrine changes in LH secretion (Wise et al., 1988a; Gilad et al., 1993) or changes in
metabolic hormones that affect ovarian function. Heat stress tended to reduce concentrations of GH
(Igono et al., 1987; McGuire et al., 1991), but not IGF-1 (McGuire et al., 1991). Elevated body
temperature may also directly affect follicular function. Culture of thecal cells at 40.5 oC reduced
androstenedione production but had no effect on E2 production from cultured granulosa cells
(Wolfenson et al., 1997). Likewise, heat shock reduced LH-stimulated P4 secretion from rat luteal
cells in a process that could be partially blocked by antisense oligonucleotide to heat shock protein
70 (HSP70; Khanna et al., 1995). A latent effect of heat stress on follicular function has also been
demonstrated, with decreased steroid production and altered follicular characteristics observed 20 to
26 days after the heat stress exposure which may affect reproduction well into the cooler season
(Roth et al., 2001).
Heat-Stress Induced Embryonic Mortality
Eliminating the problems of estrous detection caused by heat stress is not sufficient to restore
herd pregnancy rates to cool season levels because of the impact of heat on embryonic development.
Depression in pregnancy rate per insemination in warm or hot periods has been well-documented in
many regions (Stott et al., 1962; Ulberg and Burfening, 1967; Rosenberg et al., 1977; Cavestany et
al., 1985; King et al., 1988; Du Preez et al., 1991). There are two types of evidence that this
depression is caused primarily by heat stress and not other environmental variables. Specifically,
experimental application of heat stress can reduce fertility and embryonic survival (Dunlap and
Vincent, 1971; Putney et al., 1988a, 1989b; Ealy et al., 1993) and cooling cows during the summer
can improve pregnancy rate per insemination (Stott et al., 1972; Thatcher et al., 1974; Roman-Ponce
et al., 1977).
Disruption of early embryonic development results from actions on the embryo itself (Ealy et al.,
1995; Edwards and Hansen, 1997; Sugiyama, 1998; Rivera and Hansen, 2001) or on the oviductal or
uterine environment. The seasonal nature of heat stress on oocyte competence has been observed,
with fewer oocytes collected during summer months developing to blastocysts (Al-Katanani et al.,
2002). In addition, heat shock of maturing oocytes in culture can lead to reduced protein synthesis,
fertilization rate and subsequent developmental competence (Lenz et al., 1983; Edwards and
Hansen, 1996; Sugiyama, 1998). However, the deleterious effects of maternal heat stress decline as
pregnancy proceeds (Ealy et al., 1993) which may reflect acquisition of thermal resistance by the
embryo as it progresses to blastocyst stage (Edwards and Hansen, 1997). Heat shock can induce
synthesis of protective molecules, such as HSP70, as early as the 2-cell stage (Edwards and Hansen,
1996), which may contribute to a protective effect by stabilizing protein structure (Georgopoulos
and Welch, 1993), inhibiting translation (Thulasiraman et al., 1998) and preventing apoptosis (Li et
al., 1996). Though embryos are most sensitive to elevated temperature early in development, heat
stress at more advanced times can reduce development as well (Biggers et al., 1987; Ryan et al.,
1993; Sugiyama, 1998). For example, embryos > 16 cells in number at day 5 after fertilization
exposed to heat shock have an increase in the number of cells undergoing apoptosis (Paula-Lopes
and Hansen, 2000). Finally, recent evidence suggests that (at least for some breeds) the oocyte may
play a more significant role in the resistance of embryos to the deleterious effects of heat shock than
the contributions of spermatozoa to this response (Block et al., 2002).
Strategies to alleviate the effects of heat stress on reproduction / fertility
The effects of heat stress on reproduction in dairy cattle and the current status of strategies to
alleviate these effects have been recently reviewed (Jordan, 2003). These include: (1)
environmental modifications (cooling via shades, fans, sprinklers, ponds, tunnel ventilation, etc.);
(2) the use of timed-AI programs to circumvent the need for estrus detection during summer when
estrus activity is less frequent and intense; (3) using embryo transfer to eliminate the need for estrus
detection and overcome the deleterious effects of heat stress on the oocyte and early embryo; (4)
induction of accessory corpora lutea or administration of supplemental P4 post-breeding to augment
steroid hormone production; and (5) cross-breeding of dairy cattle for heat tolerance through the
infusion of Bos indicus genetics or heat tolerant genotypes-phenotypes within the Holstein breed
(e.g., the slick-haired gene; Olson et al., 2003). While all of these strategies have merit, further
research is needed to determine whether one or a combination of these strategies might be employed
to improve fertility during the summer months, particularly in the southern U.S.
Objectives
-
Defining the physiological characteristics of heat-stressed dairy cattle
-
Improving fertility of heat-stressed dairy cows without decreasing milk production
-
Improving milk production of heat-stressed dairy cows without decreasing fertility
Methods
Objective 1A. Quantifying heat stress in dairy cattle. In discussions with S-299 project participants during the past 5 years of this project, it became increasingly clear that significant differences existed among investigators in the method for quantifying heat stress at the cow level. Out of these discussions has evolved the need for comparative studies of different measures of heat stress within and among study locations, following which, a standardization of measures of heat stress (i.e., methods of measurement and reporting) could be employed. Participant locations (GA, NC, MS, PR, USVI & TN) will utilize a combination of measures for quantifying core body temperature that may include one or more of the following techniques for comparison within and across locations: rectal thermometers; tympanic probes; or rumen, implanted and/or intravaginal temperature recording devices. Data from each location will be compiled for analysis by GA and MS. Correlations of temperature readings among measurement methods and with ambient temperature / THI will be conducted. Participant locations will share measurement devices, techniques and data to compare heat stress quantitation methods for standardizing future studies of heat stress in relation to sampling methodology (device, technique, time of day, etc.). Additional studies will also be conducted to quantify heat stress in dairy cattle using novel techniques including measurements of cow coat surface measures using digital infrared thermography (MS, USVI). These studies will utilize thermography to quantify heat load as measured at the skin interface, rates of cooling at the skin surface using fan and sprinkler modifications and quantify any breed (Holstein, Jersey, Gir x Holstein and Senepol) skin thermal signature differences resulting from environmental changes (temperature, THI, time of day, shade vs. no shade, etc.). Data from each location will be compiled for analysis by MS. Objective 1B. The effect of various summer cooling strategies on symptoms of heat stress,endocrine status and lactation performance. Numerous strategies for environmental modification exist to reduce the impact of heat stress on lactation performance, however these systems can be overwhelmed during periods of severe heat stress. Thus, the cooling ability and economic feasability of novel cool systems (tunnel ventilation, targeted cooling, etc.) will be evaluated as they are developed. In each case, lactation trials will be performed to compare the performance of the novel systems with that of traditional cooling techniques (fans alone or fans plus sprinklers) using standardized research protocols. In addition to the environmental variables, body temperature, respiration rate, milk production and feed intake will be measured daily. Body weight, body condition score and concentrations of critical blood metabolites (glucose, NEFA, BUN, and minerals) will also be measured (GA, MS, NC). Consumption of electricity and water will be monitored and the time required for maintenance/ management of each system will be logged. Cow health incidence (mastitis, feet and leg problems) and breeding records will be recorded and evaluated. Because cows studied will be selected from the same herd and exposed to the same ambient conditions, comparing measures collected from control and treated cows will provide a accurate indication of the relative function of the novel cooling strategies. Data from each location will be compiled for analysis by NC. Objective 1C. Characterize the impact of prepartum cooling and identifying prepartum metabolic and endocrine markers as indicators of postpartum performance. Supplemental cooling can alleviate the effects of heat stress on intake, performance and health of lactating cows, but few data are available to characterize the effects of prepartum heat abatement. The negative effects of heat stress and the potential of supplemental cooling to increase feed intake and subsequent performance of transition dairy cows will be determined in replicated studies (GA, NC, MS) coordinated by GA. Starting at 60 days prepartum, cows treated with shade alone or shade plus supplemental cooling will be studied at each location using standardized research protocols, which will allow data to be compiled for analysis. Body temperature and climatic variables will be collected together with feed intake and body weight, body condition score and concentrations of critical blood metabolites (NEFA, BUN, and minerals) during the pre- and post- partum periods. At calving, all cows will be handled similarly and in addition to the previously listed measurements, milk yield and composition will be measured and the incidence of metabolic disorders will be recorded. Effects of climatic variables on prepartum intake and the effects of prepartum heat abatement procedures will be determined. Other studies will be conduced to characterize the effects of environment on transition cows calving in spring, summer and fall (GA, NC, FL). Nutrient intake, body weight and condition score, body temperature, and blood metabolites (insulin, NEFA, beta-hydroxybutyrate, BUN, and P4) will be determined prepartum along with milk yield and composition, health data, select endocrine concentrations and reproductive performance postpartum using a shared research protocol. Data from each location will be compiled for analysis by GA. There are several ways heat stress can impact reproduction in lactating dairy cows, with latent effects associated with smaller progeny and lower milk production resulting form heat stress events that may occur during the dry period. Therefore, studies will address whether cooling during the dry (transition) period benefits subsequent lactation and breeding (GA, MS, NC). To this end, dry cows and first calf heifers will be sorted into two groups at dry off or 60 days prepartum, respectively. One group will be cooled with shade alone during the dry period, while the other will have fans and sprinklers. Feeding will be managed similarly, and at calving all cows will be managed similarly, including environmental cooling. Data to be collected include environmental conditions (ambient temperature, relative humidity, THI), and rectal temperature. Milk yield and milk composition will be recorded following calving. Cows will be weighed at the beginning of the dry period, at about 3 weeks prepartum, immediately following calving, and twice monthly after calving. Calf body weight and indicators of calf vigor will also be recorded (aggressiveness in feeding, quantity of milk and dry feed consumed, calf serum protein, etc.). Colostrum quality will be measured using commercial kits to quantify immunoglobulin levels. In conjunction with these studies, differences in endocrine and metabolite patterns in heat stressed and non-heat stressed dairy cows during the transition period will be studied among the participants (NC, MS, GA). This will be conducted using subsets of cows calving in different seasons at the different locations, or within the same season but under different cooling regimens (no shade vs shade vs fans and sprinklers, etc.) as stated previously. Measurements will include thyroid hormones, insulin, cortisol, P4, glucose, NEFA, and PUN in postpartum cows calving in summer or cool seasons. Protocols for the timing of sampling prepartum and for standardization of the transition period studies will be coordinated by NC and GA. Postpartum milk production and other production performance characteristics will be compiled and correlated back to prepartum endocrine and metabolite patterns. Objective 1D. Characterize the effect of genetic selection in heat tolerance. Potential genetic factors related to heat stress tolerance will be studied (GA, MS). The relationship between test day yield and black globe and temperature-humidity index (BGTHI, THI) will be established for several climatological regions in the South and Midwest using records from the Dairy Records Management Service. Subsequently, genetic parameters of heat stress for production and reproduction traits will be calculated for each of the regions separately. Predicted transmitting abilities (PTA) of heat stress will be calculated for active sires available from commercial studs. Sires will be ranked based on best profitability under different levels of heat stress. Researchers will collaborate to implement shared research protocols. Data from each location will be compiled for analysis by GA. Objective 1E. Determining the relationships between coat color and cow body size on production performance in heat-stressed dairy cattle. The effects of coat color (black vs. white percentage) and cow body size (linear measures) on fertility and lactation performance of dairy cows will be evaluated by project participants as part of all trials to be conducted in this proposal (All Stations). Specifically, digital photos will be taken of each animal on studies to be conducted by project participants and percentage of black and white hair coat will be determined using image analysis software or manually (Godfrey and Hansen, 1996). This analysis will be conducted by the USVI and results returned to investigators to include in their experimental analysis. In addition, USVI will amass all the collected data into a meta-analysis to determine if there is a relationship overall between coat color, fertility and production performance in dairy cattle. Cow body size measures will also be taken at the beginning of each trial to include body weight, body condition score, heart girth, body length, hip height, and wither height. These parameters will be used to analyze the relationships between cow body size, effects of heat stress on physiological parameters (e.g., rectal temperatures and respiration rates) and production performance (e.g., milk production). In addition, MS will amass all the collected data into a meta-analysis to determine if there is a relationship overall between cow body size, the ability of cows to tolerate heat loads and production performance. Objective 2A. Use of supplemental hormone administration pre- and post-breeding to improve fertility of heat-stressed dairy cattle. Numerous studies have demonstrated that heat stress decreases serum P4 levels in cattle (reviewed by Wolfenson et al., 2000). Several studies have suggested that lower concentrations of P4 after insemination are related to reduced fertility, and some hormonal manipulations (GnRH and hCG) for increasing serum P4 post-breeding have resulted in higher pregnancy rates under some conditions (Thatcher et al., 2001; Ullah et al., 1996; Willard et al., 2003). Joint studies conducted as part of this S-299 project have (MS, NC, USVI) demonstrated that treatment with GnRH or supplemental CIDR after insemination increased pregnancy rates in heat stressed dairy cows (Willard et al., 2003; Denson et al., 2005). Continuing studies will address the timing of CIDR insertion post-breeding in relation to pregnancy rates in heat-stressed (summer) and non-heat stressed (summer cooled and/or autumn-winter) dairy cattle (NC, MS, PR, USVI) as a source of supplemental P4. Future studies will address other strategies to enhance the endogenous hormone environment and pregnancy rates in heat-stressed dairy cows using protocols for resynchronization (e.g., overlapping OvSynch or CIDR systems) to accelerate rebreeding and improve conception rates of cows returning to estrus. In vitro data from Edwards' laboratory have shown that heat-stressed oocytes mature faster than controls. Moreover, fertilization of oocytes earlier than usual obviated the effects of heat stress to reduce development. Therefore, methodologies will be explored to hasten ovulation the cow such that fertilization of heat-stressed oocytes will occur during their fertile period. Data from each location will be compiled for analysis by NC. Objective 2B. Establishing hormone markers pre-breeding as predictors of reproductive success in heat-stressed and non-heat-stressed dairy cows. There is evidence that the P4 concentration in the luteal phase prior to breeding can affect fertility (Fonseca et al., 1983; Meisterling et al., 1987). Cows with P4 levels in the luteal phase prior to AI > 11 ng/ml had pregnancy rates of 50% compared with 15% for cows with levels of < 11 ng/ml (Meisterling et al., 1987). Helmer and Britt (1986) also reported that treatment with hCG prior to insemination, which increased serum P4, increased pregnancy rates in repeat breeder cows. The use of P4 and/or other hormone concentrations pre-breeding as predictors of reproductive success (measured as an established conception) have not been evaluated in relation to heat-stressed animals or the economics of using a hormone concentration determination a determination as to whether the animal should be bred or resynchronized without breeding in relation to projected chances of a conception. NC and MS will establish protocols for pre-breeding sampling that can be employed at all participating stations as part of routine management/breeding systems at the station dairy units. Collected samples will then be analyzed for hormone concentrations (e.g., P4) and correlated to conception rate data in relation to season (i.e., summer, winter, etc.). The costs associated with hormone measurements and decision making (semen use or resynchonization) based on intended and actual outcomes from conception rate data in relation to hormone marker information will be fit to economic models. Data from each location will be compiled for analysis by NC. Objective 2C. Use of interval cooling (i.e., immediately before and after breeding) to improve fertility of problem breeders and heat-stressed dairy cows. Research from FL has shown that uterine temperature on the day of insemination and the day following insemination had the greatest effect on fertility (Gwazdauskas et al., 1973; Thatcher, 1974; Ealy et al., 1993). In most heat stress studies the reduced conception rate was associated with elevated rectal and uterine temperatures (Monty and Wolff, 1974; Stott and Wiersma, 1976; Gwasdauskas et al., 1973). Knowing this, one may cool the cow intensively for a short period around breeding and potentially improve conception rates. Cows cooled with sprinklers and fans for 2 to 3 days before and 5 to 6 days after A.I. had a conception rate of 16.0% compared with 6.2% for cows with access to shade only (Ealy et al., 1994). Cows that were cooled using air conditioning (Thatcher et al., 1974), shade (Roman-Ponce et al., 1977 ), or cooled for short periods near breeding (1 to 6.5 days; Stott and Wiersma, 1976) had significantly higher conception rates than those exposed to ambient conditions. Yet, even if the embryo survives, its development may be inhibited by elevated uterine temperature. Early embryonic deaths were partly responsible for a decrease in conception rate from 44.4 to 25.3% as the environmental temperature increased from 83.1 to 98.1F (Roman-Ponce et al., 1977). To address the effectiveness of interval cooling as a management strategy to improve summer breeding, a small area will be modified at each participating station to double fan and water sprinkling capacity over normal conditions to keep cows exposed to large volumes of air and water. Cows will be staged for breeding using targeted breeding protocols such as OvSynch or CIDR so that the dates cows are to be bred are known. Cows will be held in the intensive cooling area for one week prior to breeding and for one week following breeding to minimize heat stress during the critical breeding time. Control cows will be those exposed to normal herd management, including the 'normal' cooling systems available for the entire cow herd. All other management procedures (milking, feeding) will be the same as for the remaining herd cows. When cows are to begin the breeding protocol they will be designated as first breeding cows or multiple breeding cows to ensure that cows with potential breeding problems unrelated to heat stress are identified. Data to be collected include environmental conditions (ambient temperature, relative humidity, THI), rectal temperature (at least once, preferably twice per day), conception rate, and calving interval. Because most herds breed very few cows during summer heat, it is necessary for multiple locations to participate in this study (GA, NC, MS), and data from two to three summers will likely be necessary to generate sufficient breedings. The economics associated with the increased resources needed for interval cooling and the added inputs (fans, power, water usage, etc.) will be incorporated into the analysis of data. The impact of heat stress on oocyte/ embryonic development and survivability will be characterized (FL, TN) to better understand the effects of heat stress around the time of breeding on subsequent conception and calving rates. Data from each location will be compiled for analysis by MS. Objective 2D. Use of targeted vitamin and mineral supplementation in conjunction with estrous synchronization and timed-AI to improve fertility in heat-stressed dairy cows To address the potential for nutrition x reproduction interactions that may improve fertility post- breeding in heat-stressed dairy cows, nutritional supplementation of selected vitamins and minerals will be used in conjunction with hormonal supplementation and synchronization methodologies. Evidence suggests that one of the negative effects of heat stress on the oocyte and embryo may be an increase in free radicals (Payton et al., 2004), and vitamin A and its precursor ²-carotene could protect against this effect by acting as an antioxidant. Some studies have found improved development of oocytes and embryos (Shaw et al., 1995; Lawrence et al., 2004) and fertility after treatment with vitamin A or ²-carotene (Rakes et al., 1985; Arechiga, 1998a). Moreover, treatment with vitamin E and selenium on day 30 postpartum increased the pregnancy rate at the second AI service and reduced services per conception in cows in a temperate environment (Arechiga et al., 1998b). It would seem warranted to further investigate the effects of these vitamins on fertility of heat stressed cows. Targeted vitamin (e.g., vitamin A or ²-carotene) and/or mineral (e.g., selenium) combinations and forms (inorganic vs organic) will be administered (injections or other routes) in conjunction with OvSynch and/or CIDR protocols to assess the efficacy of these combinations for improving fertility in heat-stressed vs. non-heat-stressed dairy cows (FL, GA, MS, NC). Data from each location will be compiled for analysis by FL and NC. Objective 3A. The effect of heat stress on nutritional requirements of high-producing dairy cows. The effects of environmental stressors on nutrient requirements during will be studied in a series of trials, which will examine the effects of dietary fiber, mineral, and lipid content as well as the dietary cation-anion level (FL, MS, NC). Each location will use similar management practices, heat abatement techniques and research protocols in the replicated trials. Nutrient absorption and excretion patterns will be used to determine the impact of heat stress on nutrient requirements. Ruminally and duodenally cannulated cows will be used to measure changes in ruminal fermentation and nutrient digestibility. Selected metabolites in blood related to nutrient metabolism and stress will be monitored. Intake, milk yield, and milk composition will be evaluated in additional intact cows. Lactation performance will be evaluated using daily milk yield, milk composition analysis, and changes in body weight and condition. Researchers will collaborate to implement shared research protocols and diets to address specific nutrients. Data from each location will be compiled for analysis by MS. Objective 3B. The effects of high protein diets on production performance and summer breeding in dairy cows. The practice of feeding high CP diets during hot weather to compensate for lower DMI should be pursued with caution, and studies are needed to address the impact of these practices on production and reproductive performance. To this end, cows will be fed diets containing adequate CP and RUP for the level of intake and milk yield experienced during summer, or will be fed diets containing excessive dietary CP which are high in soluble protein (GA, MS). Cows will be assigned to targeted breeding protocols and will only be bred at the assigned timed breeding; cows will not be bred to natural heats during the breeding protocol prior to the designated timed breeding. Diets will be implemented immediately after calving and will be continued through the early Fall when temperatures are still relatively high in the southeast. The relationship between high BUN and important reproductive hormones will be evaluated. Data collected will include milk yield and composition, BUN and MUN, conception rate, and P4, prolactin, and cortisol. Environmental conditions (ambient temperature, relative humidity, THI) will also be recorded to assess the degree of heat stress experienced during the study periods. Data from each location will be compiled for analysis by GA. Objective 3C. The effect of dietary supplements (antioxidants, minerals and probiotics) to enhance nutrient intake and digestibility. Dietary ingredients (enzymes, direct fed microbials, yeast cultures, etc.) designed to improve dry matter digestibility have shown some potential for improving lactational performance. Studies will be conducted to determine if these additives will improve performance during periods of heat stress (MS, GA). Effects of these ingredients will be determined by measuring DMI, milk yield, change in body condition score and weight, efficiency of production and nutrient digestibility. Shared protocols will be used to provide replication of treatments and data compiled for analysis. The potential for improving lactational and reproductive performance of dairy cows by adding dietary antioxidants such as vitamin E and selenium will be examined. Blood will be collected from cows for analysis of vitamin E and Se content. The incidence of retained placentas and uterine infections developing after freshening will be recorded. Cows will be bred at estrus after the 90 d voluntary waiting period and pregnancy confirmed by rectal palpation at 45 days after breeding. Data from each location will be compiled for analysis by MS.Measurement of Progress and Results
Outputs
- This project is expected to yield designs and methods to quantify and mitigate the effects of heat stress on the southern dairy industry.
- Information developed will assist in transferring new technology to producers and citizens through web-based and paper publications, spreadsheets, workshops, and field days.
- The effects of heat-stress on the dairy cow will be more clearly characterized and improved techniques to measure the impact will be provided.
- The potential of alternative management strategies to mitigate heat-stress in hot, humid climates will be identified.
Outcomes or Projected Impacts
- Results of this project will provide additional insight into the biological changes induced by heat stress which result in decreased lactational and reproductive performance for lactating dairy cows. With a better understanding of the physiologic and endocrine changes that occur in response to heat stress. One particular focus of this project is to assess the potential for improving production and reproduction in the subsequent lactation through incorporation of effective heat stress abatement techniques in combination with nutritional modifications during the dry period. This, combined with hormone therapy, would greatly improve both lactation and reproductive performance. Identification of genetic lines that are more tolerant of heat stress offers the potential to reduce the trend toward lower reproductive performance associated with selection for improved milk production. Dietary modifications or supplements that would either reduce heat production through improved digestion or production of metabolites that interfere with reproduction would be easy to implement.
- Implementation of these practices at the farm level would improve overall efficiency and profitability. Management practices that improve overall efficiency would also reduce the number of animals sold due to poor reproduction or production, reducing the number of costly replacements required to maintain herd size. Improved lactation performance during late summer and fall would reduce the amount of milk imported into the Southeast US which is of particular interest to the many milk processors. Long term, this could reduce prices consumers pay as well as reduce some of the problems associated with shipping milk into the market.
- Results of this project will be communicated to the end users through a variety of media including scientific journal articles, popular press articles, presentations at regional/state producer conferences, and presentations at regional, state, and local field days. Results of collaborative research projects will be reported in jointly authored manuscripts and submitted for publication in refereed journals selected by the authors. A regional symposium with a published proceedings will be scheduled near the end of the project to present results of the project and provide recommendations for reducing heat stress to enhance production and reproduction. Joint extension bulletins and popular press articles will be prepared to communicate the results of this project. A web site will be established for the project. The site will include a list of project members, annual reports, and publications resulting from the project. As the project progresses, significant outcomes and recommendations will be posted.
Milestones
(0):Projected Participation
View Appendix E: ParticipationOutreach Plan
ÿResults of this project will be made available to the scientific community, Extension Specialists
and County Agents via refereed publication, technical reports, internal university reports, and
research and extension publications. A webpage will be maintained by the University of the Virgin
Islands. Training of graduate and undergraduate students will be involved at all stages of the
project.
Organization/Governance
This committee will consist of an Administrative Advisor appointed by the Southern Association of
Agricultural Experiment Station Directors (SAAESD), a Technical Advisor appointed by USDA/
CSREES, and one or more representatives from each cooperating Agricultural Experiment Station.
Participating SAES with more than one representative will designate one voting member.
Members of the Regional Technical Committee will elect a Chair, a Chair-elect and a Secretary
from the membership to serve as officers for the Regional Technical Committee. The Executive
Committee shall consist of the current officers. A new Secretary shall be elected each year at the
annual meeting by the voting members of the Regional Technical Committee. The previous Secretary
shall become the Chair-elect for one year before ascending to the office of Chair. The Chair, in
consultation with the Administrative Advisor, will notify the Regional Committee Members of the
time and place of the annual meeting, prepare the agenda and preside at the meeting. The Chair-elect
will be responsible for preparing the annual report. The secretary will record and prepare minutes of
the annual meeting for distribution to the membership, to SAAESD and to CSREES. If any member
of the Executive Committee resigns, the remaining members shall, with the advice and consent of the
Administrative Advisor, appoint a member of the Regional Technical Committee to fill the vacancy.
Literature Cited
(*Performed as part of the S-299 project)
Abilay, T. A., H. D. Johnson, and M. Madan. 1975. Influence of environmental heat on peripheral plasma progesterone and cortisol during the bovine estrous cycle. J. Anim. Sci. 58:1836-1840.
Al-Katanani, Y. M., F. F. Paula-Lopes, and P. J. Hansen. 2002. Effect of season and exposure to heat stress on oocyte competence in Holstein cows. J. Dairy Sci. 85:390396.
Al-Katanani, Y.M., D. W. Webb, and P. J. Hansen. 1998. Factors affecting seasonal variation in nonreturn rate of lactating dairy cows. J. Dairy Sci. 81 (Suppl. 1): 217 (Abstr.).
Alliston, C. W., and L. C. Ulberg. 1961. Early pregnancy loss in sheep at ambient temperatures of 70 and 90 F. as determined by embryo transfer. J. Anim. Sci. 20:608-613.
Arechiga, CF, CR Staples, LR McDowell, PJ Hansen. 1998a. Effects of timed insemination and supplemental -carotene on reproduction and milk yield of heat stressed dairy cows. J Dairy Sci 81:390-402.
Arieli, A., I. Bruckental and G. Adin. 2004. The effect of protein intake on performance of cows in hot environmental temperatures. J. Dairy Sci, 87:620-629.
Armstrong, D. V. 1994. Heat stress interaction with shade and cooling. J. Dairy Sc. 77:2044- 2050.
Badinga, L., W. W. Thatcher, T. Diaz, M. Drost, and D. Wolfenson. 1993. Effect of environmental heat stress on follicular development and steroidogenesis in lactating Holstein cows. Theriogenology 39:797-810.
Beede, D. K., and R. J. Collier. 1986. Potential nutritional strategies for intensively managed cattle during thermal stress. J. Anim. Sci. 62:543-554.
Bell, A. W. 1995. Regulation of organic nutrient metabolism during transition from late pregnancy to early lactation. J. Anim. Sci. 73:2804-2819.
Bell, A. W., B. W. McBride, R. Slepetis, R. J. Early, and W. B. Currie. 1989. Chronic heat stress and prenatal development in sheep: I. Conceptus growth and maternal plasma hormones and metabolites. J. Anim. Sci. 67:3289-3299.
Berman, A., Y. Folman, M. Kaim, M. Mamen, Z. Herz, D. Wolfenson, A. Arieli, and Y. Graber. 1985. Upper critical temperatures and forced ventilation effects for high-yielding dairy cows in a subtropical environment. J. Dairy Sci. 68:1488-1495.
Bertics, S. J., R. R. Grummer, C. Cadorniga-Valino, and E. E. Stoddard. 1992. Effect of prepartum dry matter intake on liver triglyceride concentration and early lactation. J. Dairy Sci. 75:1914-1922.
Biggers, B. G., R. D. Geisert, R. P. Wetteman, and D. S. Buchanan. 1987. Effect of heat stress on early embryonic development in the beef cow. J. Anim. Sci. 64:1512-1518.
Blackshaw, J. K., and A. W. Blackshaw. 1994. Heat stress in cattle and the effect of shade on production and behaviour: a review. Aust. J. Exp. Agric. 34:285-295.
Block, J., C. C. Chase, Jr. and P. J. Hansen. 2002. Inheritance of resistance of bovine preimplantation embryos to heat shock: relative importance of the maternal vs paternal contribution. Mol. Reprod. Dev. 63:32-37.
Bucklin, R. A., L. W. Turner, D. K. Beede, D. R. Bray, and R. W. Hemken. 1991. Methods to relieve heat stress for dairy cows in hot, humid climates. App. Eng. In Agric. 7:241-247.
Cavestany D., A. B. El-Wishy, and R. H. Foote. 1985. Effect of season and high environmental temperature on fertility of Holstein cattle. J. Dairy Sci. 68:1471-1478.
Chan, S. C., J. T. Huber, Z. Wu, K. H. Chen, and J. Simas. 1992. Effect of fat supplmentation and protein source on performance of dairy cows in hot environmental temperatures. J. Dairy Sci. 75(Suppl. 1):175. (Abstr.)
Chan, S. H., J. T. Huber, Z. Wu, J. Simas, K. H. Chen, F. Santos, A. Rodrigues, and J. Varela. 1993. Effects of supplementation of fat and evaporating cooling of dairy cows subjected to hot temperatures. J. Dairy Sci. 76(Suppl. 1):184. (Abstr.)
Christison, G. I. and H. D. Johnson, H. D. 1972. Cortisol turnover in heat-stressed cows. J. Anim. Sci. 35:1005-1010.
Chen, K. H., J. T. Huber, C. B. Theurer, D. V. Sullivan. 1993. Effect of supplemental protein quality and evaporative cooling on lactational performance of Holstein cows in hot weather. J. Dairy Sci. 76:819-825.
Cole, J. A. and P. J. Hansen. 1993. Effects of administration of recombinant bovine somatotropin on the responses of lactating and nonlactating cows to heat stress. J. Am. Vet. Med. Assn. 203:113-117.
Collier, R. J., D. K. Beede, W. W. Thatcher, L. A. Israel, and C. J. Wilcox. 1982a. Influences of environment and its modification on dairy animal health and production. J. Dairy Sci. 65:2213-2227.
Collier, R. J. , S. G. Doelger, H. H. Head, W. W. Thatcher, and C. J. Wilcox. 1982b. Effects of heat stress during pregnancy on maternal hormone concentrations, calf birth weight and postpartum milk yield of Holstein cows. J. Anim. Sci. 54:309-319.
Dreiling, C. E., F. S. Carman III, and D. E. Brown. 1991. Maternal endocrine and fetal metabolic responses to heat stress. J. Dairy Sci. 74:312-327.
Dunlap, S. K., and C. K. Vincent. 1971. Influence of postbreeding thermal stress on conception rate in beef cattle. J. Anim. Sci. 32: 1216-1218.
Du Preez, J. H., S. J. Terblanche, W. H. Giesecke, C. Maree, and M. C. Welding. 1991. Effect of heat stress on conception in a dairy herd model under South African conditions. Theriogenology 35:1039-1049.
Ealy, A. D., C. F. Arechiga, D. R. Bray, C. A. Risco, and P. J. Hansen. 1994. Effectiveness of short-term cooling and vitamin E for alleviation of infertility induced by heat stress in dairy cows. J. Dairy Sci. 77:3601-3607.
Ealy, A. D., M. Drost, and P. J. Hansen. 1993. Developmental changes in embryonic resistance to adverseeffects of maternal heat stress in cows. J. Dairy. Sci. 76:2899-2905.
Ealy, A. D., J. L. Howell, V. H. Monterroso, C. F. Aréchiga, and P. J. Hansen. 1995. Developmental changes in sensitivity of bovine embryos to heat shock and use of antioxidants as thermoprotectants. J. Anim. Sci. 73:1401-1407.
Edwards, J. L., and P. J. Hansen. 1996. Elevated temperature increases heat shock protein 70 synthesis in bovine two-cell embryos and compromises function of maturing oocytes. Biol. Reprod. 55:340- 346.
Edwards, J. L., and P. J. Hansen. 1997. Differential responses of bovine oocytes and preimplantation embryos to heat shock. Mol. Reprod. Dev. 46:138-145.
Elvinger, F., R. P. Natzke, and P. J. Hansen. 1992. Interactions of heat stress and bovine somatotropin affecting physiology and immunology of lactating cows. J. Dairy Sci. 75:449-462.
Gangwar P.D., C. Branton and D. L. Evens. 1965. Reproductive and physiological responses in Holstein heifers to controlled and natural climatic conditions. J. Dairy Sci., 48:222-227.
Georgopoulos, C. and W. J. Welch, W.J. 1993. Role of the major heat shock proteins as molecular chaperones. Annu. Rev. Cell Biol., 9, 601-634.
Gilad, E., R. Meidan, A. Berman, Y. Graber, and D. Wolfenson. 1993. Effect of tonic and GnRH-induced gonadotrophin secretion in relation to concentration of oestradiol in plasma of cyclic cows. J. Reprod. Fertil. 99:315-321.
Godfrey, R. W., D. D. Lunstra, T. G. Jenkins, J. G. Berardinelli, M. J. Guthrie, D. A. Neuendorff, C. R. long, and R. D. randel. 1990. Effect of season and location on semen quality and serum concentrations of luteinizing hormone and testosterone in Brahman and Hereford bulls. J. Anim. Sci 68:734-749.
Godfrey, R. W. and P. J. Hansen. 1996. Reproduction and milk yield of Holstein cows in the US Virgin Islands as influenced by time of year and coat color. Archivos Latinoamericanos de Producciòn Animal 4:31-44.
Grummer, R. R. 1995. Impact of changes in organic nutrient metabolism on feeding the transition dairy cow. J. Anim. Sci. 73:2820-2833.
Gwazdauskas, F. C., W. W. Thatcher, C. A. Kiddy, M. J. Paape, and C. J. Wilcox. 1981. Hormonal patterns during heat stress following PGF2a-tham salt induced luteal regression in heifers. Theriogenology 16:271-285.
Gwazdauskas, F. C., W. W. Thatcher, and C. J. Wilcox. 1973. Physiological, environmental, and hormonal factors at insemination which may affect conception. J. Dairy Sci. 57:873.
*Hansen, P.J. and Aréchiga, C.F. 1999. Strategies for managing reproduction in the heat-stressed dairy cow. J. Anim. Sci. 77 (Suppl. 2): 36-50.
Hein K. G. and R. D. Allrich 1992. Influence of exogenous adrenocorticotropic hormone on estrous behavior in cattle J. Anim Sci. 1992: 243-247.
Helmer SD, Britt JH. 1986. Fertility of dairy cattle treated with human chorionic gonadotropin to stimulate progesterone secretion. Theriogenology 26:683-695.
Holter, J. B., J. W. West, and M. L. McGilliard. 1997. Predicting ad libitum dry matter intake and yield of Holstein cows. J. Dairy Sci. 80:2188-2199.
Holter, J. B., J. W. West, M. L. McGilliard, and A. N. Pell. 1996. Predicting ad libitum dry matter intake and yields of Jersey cows. J. Dairy Sci. 79:912-921.
Howell, J. L., A. S. Smith and, J. W. Fuquay. 1994. Corpus luteum growth and function in lactating Holstein cows during spring and summer. J. Dairy Sci. 77:735-739.
Hulme, M. 1997. Global warming. Prog. Phys. Geogr. 21: 446-453.
Huber, J. T., G. Higginbotham, R. A. Gomez-Alarcon, R. B. Taylor, K. H. Chen, S. C. Chan, and Z. Wu. 1994. Heat stress interactions with protein, supplemental fat, and fungal cultures. J. Dairy Sci. 77:2080-2090.
Huber, J. T., Z. Wu, S. C. Chan, and J. Simas. 1993. Feeding of fat during heat stress conditions,and combinations of fat sources. Page 90 in Proc. Southwest Nutr. Manage. Conf., Phoenix, AZ, Dept. Anim. Sci., Univ. of Arizona, Tucson.
Igono, M. O., H. D. Johnson, B. J. Stevens, G. F. Krause, and M. D. Shanklin. 1987. Physiological,productive, and economic benefits of shade, spray, and fan system versus shade for Holstein cows during summer heat. J. Dairy Sci. 70:1069-1079.
Johnson, H. D., R. Li, W. Manalu, and K. J. Spencer-Johnson. 1991. Effects of somatotropin on milk yield and physiological responses during summer farm and hot laboratory conditions. J. Dairy Sci. 74:1250-1262.
Johnson, H. D., A. C. Ragsdale, I. L. Berry, and M. D. Shanklin. 1963. Temperature-humidity effects including influence of acclimation in feed and water consumption of Holstein cattle. Univ. of Missouri Res. Bull. No. 846.
Jordan, E. R. 2003. Effects of Heat Stress on Reproduction. 2003. J. Dairy Sci. 86:(Suppl. E.): E104-E114.
Kabuga, J. D., and K. Sarpong. 1991. Influence of weather conditions on milk production and rectal temperature of Holsteins fed two levels of concentrate. Int. J. Biometeorol. 34:226-230.
Khanna, A., R. F. Aten, and H. R. Behrman. 1995. Heat shock protein-70 induction mediates luteal regression in the rat. Mol. Endocrinol. 9:1431-1440.
Kibler, H. H. and S. Brody. 1950. Influence of temperature, 5 to 95 F, on evaporative cooling from the respiratory and exterior body surfaces in Jersey and Holstein cows. Missouri Agric. Exp. Sta. Bull. No. 461. Columbia.
King, V. L., S. K. Denise, D. V. Armstrong, M. Torabi, and F. Wiersma. 1988. Effects of a hot climate on the performance of first lactation Holstein cows grouped by coat color. J. Dairy Sci. 71:1093- 1096.
Lenz RW, G. D. Ball, M. L. Leibfried, R, L. Ax and N. L. First.1983. In vitro maturation and fertilization of bovine oocytes are temperature-dependent processes. Biol. Reprod. 29:173179.
Li W. X., C. H. Chen, C. C. Ling and G. C. Li. 1996. Apoptosis in heat-induced cell killing: the protective role of hsp-70 and the sensitization effect of the c-myc gene. Radiat. Res.145:324-30.
Magdub, A., H. D. Johnson, and R. L. Belyea. 1982. Effect of environmental heat and dietary fiber on thyroid physiology of lactating cows. J. Dairy Sci. 65:2323-2331.
Malayer, J. R., and P. J. Hansen. 1990. Differences between Brahman and Holstein cows in heat-shock induced alterations of protein secretion by oviducts and uterine endometrium. J. Anim. Sci. 68:266-280.
Malayer, J. R., P. J. Hansen, and W. C. Buhi. 1988. Effect of day of the oestrous cycle, side of the reproductive tract and heat shock on in vitro protein secretion by bovine endometrium. J. Reprod. Fertil. 84:567-578.
Mallonee, P.G., C. J. Wilcox, R. J. Collier and D. K. Beede, D.K. 1985. Production and physiological responses of dairy cows to varying dietary potassium during heat stress. J. Dairy Sci.68 :1479-1487.
McGuire, M. A., D. K. Beede, M. A. DeLorenzo, C. J. Wilcox, G. B. Huntington, C. K. Reynolds,and R. J. Collier. 1989. Effects of thermal stress and level of feed intake on portal plasma flow and net fluxes of metabolites in lactating Holstein cows. J. Anim. Sci. 67:1050-1060.
McGuire, M. A., D. K. Beede, R. J. Collier, F. C. Buonomo, M. A. DeLorenzo, C. J. Wilcox, G. B.Huntington, and C. K. Reynolds. 1991. Effects of acute thermal stress and amount of feed intake on concentrations of somatotropin, insulin-like growth factor (IGF)-I and IGF-II, and thyroid hormones in plasma of lactating Holstein cows. J. Anim. Sci. 69:2050-2056.
Meisterling EM, Dailey RA. 1987. Use of concentrations of progesterone and estradiol 17 beta in milk in monitoring postpartum ovarian function in dairy cows. J Dairy Sci 70:2154-2161.
Miller, H. L. and C. W. Alliston, C.W. 1974. Plasma corticoids of Angus heifers in programmed circadian temperatures of 17 to 21 OC and 21 to 34 OC. J. Anim. Sci. 38:819-822. and L. K. Wolff. 1974. Summer heat stress and reduced fertility in Holstein-Friesian cows in Arizona. Am. J. Vet. Res. 35:1496-1500.
Moody, E. G., P. J. Van Soest, R. E. McDowell, and G. L. Ford. 1967. Effect of high temperature and dietary fat on performance of lactating cows. J. Dairy Sci. 50:1909.
Moore, R. B., J. W. Fuquay, and W. J. Drapala. 1992. Effects of Late Gestation Heat Stress on Postpartum Milk Production and Reproduction in Dairy Cattle. J. Dairy Sci. 75:1877-1882.
National Research Council. 2001. Nutrient Requirements of Dairy Cattle (7th Rev. Ed.) National Academy Press. Washington, D.C.
Nebel, R. L., S. M. Jobst, M. B. G. Dransfield, S. M. Pandolfi, and T. L. Bailey. 1997. Use of radio frequency data communication system, HeatWatch(r), to describe behavioral estrus in dairy cattle. J. Dairy Sci. 80 (Suppl. 1):179 (Abstr.).
Olson, T. A., A. C. Hammond, C. C. Chase, Jr. and C. Lucena. 2003. Evidence of a major gene influencing hair length and heat tolerance in Bos taurus cattle. J. Anim. Sci. 81:80-90.
Palmquist, D. L. 1984. Use of fat in diets for lactating cows. Page 357 in Fats in Animal Nutrition. J. Wiseman, ed. Butterworths, Boston, MA.
Paula-Lopes, F. F. and Hansen, P. J. 2002. Heat-shock induced apoptosis in preimplantation bovine embryos is a developmentally-regulated phenomenon. Biol. Reprod., 66:1169-1177.
*Payton, RR, R Romar, P Coy, AM Saxton, JL Lawrence, JL Edwards. 2004. Susceptibility of bovine germinal vesicle stage oocytes from antral follicles to direct effects of heat stress in vitro. Biol Reprod 71"1303-1308.
Putney, D. J., M. Drost, and W. W. Thatcher. 1988a. Embryonic development in superovulated dairy cattle exposed to elevated ambient temperature between days 1 to 7 post insemination. Theriogenology 30:195-209.
Putney, D. J., J. R. Malayer, T. S. Gross, W. W. Thatcher, P. J. Hansen, and M. Drost. 1988b. Heat-stress induced alterations in the synthesis and secretion of proteins and prostaglandins by cultured bovine conceptuses and uterine endometrium. Biol. Reprod. 39: 717-728.
Putney, D. J., S. Mullins, W. W. Thatcher, M. Drost, and T. S. Gross. 1989. Embryonic development in superovulated dairy cattle exposed to elevated ambient temperatures between the onset of estrus and insemination. Anim. Reprod. Sci. 19:37-51.
*Ravagnolo, O., and I. Misztal. 2000. Genetic component of heat stress in dairy cattle, parameter estimation. J. Dairy Sci. 83:2126-2130.
*Ravagnolo, O., I. Misztal, and G. Hoogenboom. 2000. Genetic component of heat stress in dairy cattle, development of heat index function. J. Dairy Sci. 83:2120-2125.
Richards, J. I. 1985. Effect of high daytime temperature on the intake and utilization of water in lactating Friesian cows. Trop. Anim. Health Prod. 17:209-217.
Rivera, R. M., and P. J. Hansen. 2001. Development of cultured bovine embryos after exposure to increased temperatures in the physiological range. Reproduction 121:107115.
Roman-Ponce, H., W. W. Thatcher, D. E. Buffington, C. L. Wilcox, and H. H. Van Horn. 1977. Physiological and production responses of dairy cattle to a shade structure in a subtropical environment. J. dairy Sci. 60:424.
Roman-Ponce, H., W. W. Thatcher D. Caton, D. H. Barron, and C. J. Wilcox. 1978. Thermal stress effects on uterine blood flow in dairy cows. J. Anim. Sci. 46:175-180.
Roman-Ponce, H., W. W. Thatcher, and C. J. Wilcox. 1981. Hormonal interrelationships and physiological responses of lactating dairy cows to a shade management system in a subtropical environment. Theriogenology 16:139-154.
Ronchi, B., P. A. Accorsi, A. Nardone, E. Seren, N. Lacetera, G. Stradaioli, A. V. Supplizi and U. Bernabucci. 2001. Influence of heat stress or feed restriction on plasma progesterone,oestradiol-17², LH, FSH, prolactin and cortisol in Holstein heifers. Livestock Prod. Sci. 68:231-242.
Rosenberg, M., Z. Herz, M. Davidson, and Y. Folman. 1977. Seasonal variations in post-partum plasma progesterone levels and conception in primiparous and multiparous dairy cows. J. Reprod. Fertil. 51:563-568.
Rosenberg, M., Y. Folman, Z. Herz, I. Flamenbaum, A. Berman, and M. Kaim.1982. Effect of climatic conditions on peripheral concentrations of LH, progesterone and oestradiol-17ß in high milk-yielding cows. J. Reprod. Fertil. 66:139-146.
Roth, Z., R. Braw-Tal, D. Wolfenson, R. Meidan and A. Shaham-Albalancy. 2001. Delayed effect of heat stress on steroid production in medium-sized and preovulatory bovine follicles. Reprod. (Cambridge) 121:745-751.
Roth, Z., D. Wolfenson, R. Braw-Tal and R. Meidan. 2000. Immediate and delayed effects of heat stress on follicular development and its association with plasma FSH and inhibin concentration in cows. J. Reprod. Fertil.120:83-90.
Roush, W. 1994. Population - the view from Cairo. Science 265: 1164-1167.
Ryan, D. P., J. F. Prichard, E. Kopel, and R. A. Godke. 1993. Comparing early embryo mortality in dairy cows during hot and cool seasons of the year. Theriogenology 39:719-737.
Schneider, P. L., D. K. Beede, and C. J. Wilcox. 1986. Responses of lactating cows to dietary sodium source and potassium quantity during heat stress. J. Dairy Sci. 69:99-110.
Schneider, P. L., D. K. Beede, C. J. Wilcox, and R. J. Collier. 1984. Influence of dietary sodium and potassium bicarbonate and total potassium on heat-stressed lactating dairy cows. J. Dairy Sci. 67:2546-2553.
Seath, D. M., and G. D. Miller. 1948. Effect of water sprinkling with and without air movement on cooling dairy cows. J. Dairy Sci. 5:361-366.
Stott, G. H. 1981. What is animal stress and how is it measured. J. Anim. Sci. 52:150-153.
Stott, G. H., and R. J. Williams. 1962. Causes of low breeding efficiency in dairy cattle associated with seasonal high temperatures. J. Dairy Sci. 45:1369-1375.
Stott, G. H., and F. Wiersma. 1976. Short-term thermal relief for improved fertility in dairy cattle during hot weather. Int. J. Biometeorol. 20:344.
Stott, G. H., Wiersma, F., and Woods, J. M. 1972. Reproductive health program for cattle subjected to high environmental temperatures. J. Am. Vet. Med. Assn. 161:1369-1375.
Sugiyama, S. 1998. Development of a model to study the direct effects of hyperthermia on bovine ovum and embryo development. Ph.D. Thesis, University of Queensland, Brisbane.
Thatcher, W. W., F. C. Gwazdauskas, C. J. Wilcox, T. Toms, H. H. Head, D. E. Buffington, and W. B. Frederksson. 1974. Milking performance and reproductive efficiency of dairy cows in an environmentally controlled structure. J. Dairy Sci. 57:304-307.
Thatcher, W. W., and R. J. Collier. 1986. Effects of climate on bovine reproduction. In: D. A. Morrow (Ed.) Current Therapy in Theriogenology 2. pp. 301-309. W. B. Saunders, Philadelphia.
Thatcher, W. W. 1974. Effects of season, climate, and temperature on reproduction and lactation. J. Dairy Sci. 57:360.
Thatcher, WW, F Moreira, JEP Santos, RC Mattos, FL Lopes, SM Pancari, CA Risco. 2001. Effects of hormonal treatments on reproductive performance and embryo production. Theriogenology 55:75-89.
Thulasiraman, V., C. F. Yang, and J. Frydman. 1999. In vivo newly translated polypeptides are sequestered in a protected folding environment. EMBO J. 18:85-95.
Trout, J. P., L. R. McDowell, and P. J. Hansen. 1998. Characteristics of the estrous cycle and antioxidant status of lactating Holstein cows exposed to heat stress. J. Dairy Sci. 81:1244-1250.
Ullah, G, JW Fuquay, T Keawakhomg, BL Clark, DE Pogue, EJ Murphey. 1996. Effects of GnRH at estrus on subsequent luteal function and fertility in lactating Holsteins during heat stress. J Dairy Sci 79:1950-1953.
Ulberg, L. D., and P. J. Burfening. 1967. Embryo death resulting from adverse environment on spermatozoa or ova. J. Anim. Sci. 26:571577.
Warren, W. P., F. A. Martz, K. H. Asay, E. S. Hilderbrand, C. G. Payne, and J. R. Vogt. 1974. Digestibility and rate of passage by steers fed tall fescue, alfalfa and orchardgrass hay in 18 and 32
C ambient temperature. J. Anim. Sci. 39:93-96.
West, J. W., B. G. Mullinix, and T. G. Sandifer. 1991. Effects of physiologic responses of lactating Holstein and Jersey cows during hot, humid weather. J. Dairy Sci. 74:840-851.
*West, J. W., B. G. Mullinix, and J. K. Bernard. 2000. Influence of environmental conditions on body temperature, dry matter intake, and milk yield for lactating cows from spring through summer in the southeast. J. Dairy Sci. 83 (Suppl. 1):232. (Abstr.)
*Willard, S., S. Gandy, S. Bowers, K. Graves, A. Elias, C.S. Whisnant. 2003. The effects of GnRH administration on serum concentrations of progesterone and pregnancy rates in dairy cows exposed to mild summer heat stress. Theriogenology 59:1799-1810.
Williams, R. J, A. M. Chapa, T. O. Riley, D. O. Pogue, S. T. Willard and T. R. Smith. 2004. The use of tunnel ventilation cooling and brown mid-rib (BMR) corn silage to reduce the effects of heat stress in lactating dairy cows. J. Anim. Sci. 82(Suppl. 2):21(Abstr. 83).
Wilson, S. J., C. J. Kirby, A. T. Koenigsfield, D. H. Keisler, and M. C. Lucy. 1998a. Effects of controlled heat stress on ovarian function of dairy cattle. 2. Heifers. J. Dairy Sci. 81: 2132-2138.
Wilson, S. J., R. S. Marion, J. N. Spain, D. E. Spiers, D. H. Keisler, and M. C. Lucy. 1998b. Effects of controlled heat stress on ovarian function of dairy cattle. 1. Cows. J. Dairy Sci. 81 81: 2139-2144.
Wise, M. E., D. V. Armstrong, J. T. Huber, R. Hunter, and F. Wiersma. 1988a. Hormonal alterations in the lactating dairy cow in response to thermal stress. J. Dairy Sci. 71:2480-2485.
Wise, M. E., R. E. Rodriguez, D. V. Armstrong, J. T. Huber, F. Wiersma, and R. Hunter. 1988b. Fertility and hormonal responses to temporary relief of heat stress in lactating dairy cows. Theriogenology 29:1027-1035.
Wolfenson, D., F. F. Bartol, L. Badinga, C. M. Barros, D. N. Marple, K. Cummings, D. Wolfe, M. C. Lucy, T. E. Spencer, and W. W. Thatcher. 1993. Secretion of PGF2a and oxytocin during hyperthermia in cyclic and pregnant heifers. Theriogenology 39:1129-1141.
Wolfenson, D., I. Flamenbaum, and A. Berman. 1988a. Hyperthermia and body energy store effects on estrous behavior, conception rate, and corpus luteum function in dairy cows. J. Dairy Sci. 71:3497-3504.
Wolfenson, D., I. Flamenbaum, and A. Berman. 1988b. Dry period heat stress relief effects on prepartum progesterone, calf birth weight, and milk production. J. Dairy Sci. 71:809-818.
Wolfenson, D., M. Kaim, Y. Folman, R. Meidan, H. Sonego, A. Bloch and A. Shaham- Albalancy. 2002. Seasonal differences in progesterone production by luteinized bovine thecal and granulosa cells. Dom. An. Endocr. 22:81-90.
Wolfenson, D., B. J. Lew, W. W. Thatcher, Y. Graber, and R. Meidan. 1997. Seasonal and acute heat stress effects on steroid production by dominant follicles in cows. Anim. Reprod. Sci. 47:9-19.
Wolfenson, D., W. W. Thatcher, L. Badinga, J. D. Savio, R. Meidan, B. J. Lew, R. Braw-Tal, and A. Berman. 1995. Effect of heat stress on follicular development during the estrous cycle in lactating dairy cattle. Biol. Reprod. 52:1106-1113.
Wolffe, A. P., A. J. Perlman, and J. R. Tata. 1984. Transient paralysis by heat shock of hormonal regulation of gene expression. EMBO J. 3:2763-2770.
Younas, M., J. W. Fuquay, A. E. Smith, and A. B. Moore. 1993. Estrous and endocrine responses of lactating Holsteins to forced ventilation during summer. J. Dairy Sci. 76:430-436.
Zamet, C. N., V. F. Colenbrander, C. J. Callahan, B.P. Chew, R. E. Erb, and N. J. Moeller. 1979. Variables associated with peripartum traits in dairy cows. II. Interrelationships among disorders and their effects on intake of feed and on reproductive efficiency. Theriogenology 11:245.