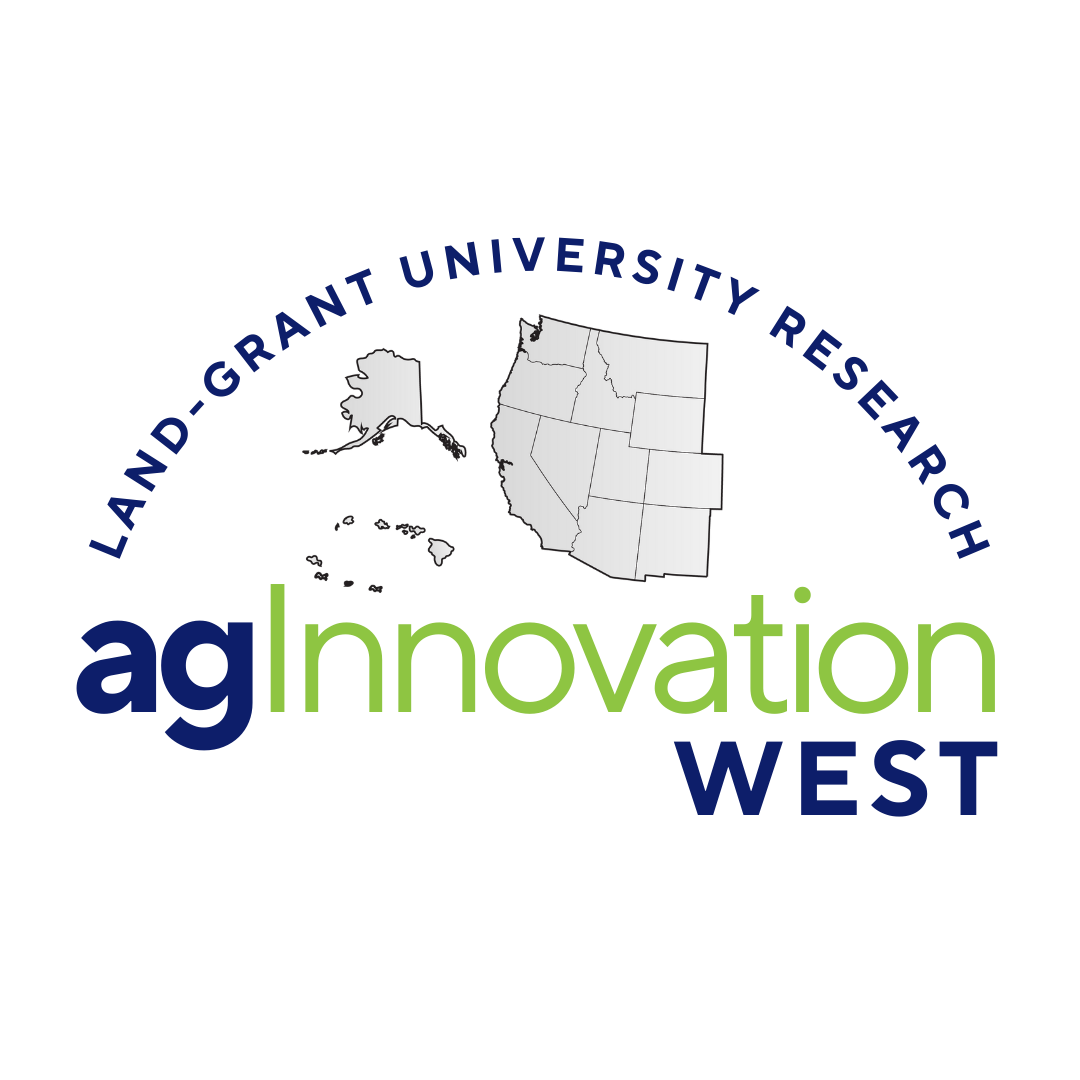
W1188: Characterizing Mass and Energy Transport at Different Scales
(Multistate Research Project)
Status: Inactive/Terminating
W1188: Characterizing Mass and Energy Transport at Different Scales
Duration: 10/01/2004 to 09/30/2009
Administrative Advisor(s):
NIFA Reps:
Non-Technical Summary
Statement of Issues and Justification
Citizens, our ultimate stakeholders, consider that affordable, high-quality food and fiber are central to their quality of life. At the same time, they overwhelmingly desire a clean and safe environment in which to live. Soils are critically important components of the earth's biosphere. They are central to food and fiber production, and to global environmental quality (Doran and Parkin, 1994). Balancing societal goals for a clean environment with profitable and sustainable agricultural systems requires sound fundamental understanding of the complex interrelationships between mass (e.g., water, nutrients, pesticides) and energy (e.g., heat, light) transport in soils and exchanges with the adjacent atmosphere. These processes are highly variable in space and time, and the transfer of our understanding derived at given scales to applications at greater or smaller scales has not always been successful. Identifying relevant core processes, developing new means for quantifying, measuring or simulating essential components, and understanding the interrelationships between agricultural and environmental goals or problems will lead to more powerful management of our natural resources. Because of the broad and interrelated nature of the problems associated with mass and energy transport in soils and atmosphere, collaborative multidisciplinary approaches are necessary to substantially enhance our abilities to manage agricultural landscapes. No single individual or institution has sufficient talents or resources to undertake the required activities. A synergism of multidisciplinary scientists working collaboratively through the auspices of our multistate research committee can have a significant impact on immediate as well as longer-term new technologies that will provide our society with safe, low-cost food supplies, while simultaneously reducing the environmental impacts of food production and related practices. Management strategies that utilize the unsaturated soil (vadose) zone are widely considered superior to those involving groundwater, due to long chemical residence times and compounded access problems once contaminants enter groundwater reservoirs. In the latter case, damages are often irreversible, so prevention and remediation of soil and groundwater contamination should begin with informed management of the unsaturated zone (van Genuchten, 1994). The transport of mass and energy define many of the most relevant soil processes. Knowledge concerning the behavior of soil water and soil-applied chemicals including nutrients, pesticides and contaminants greatly expanded during the era following World War II, and has continued at an increasing pace to the present. Transport of energy in the form of heat impacts all biogeochemical reactions taking place in soil environments. Water, heat, and chemical transport are often coupled. Greater understanding has led to increased appreciation for the complexity and myriad interrelationships of mass and energy transport phenomena in soils, and of the necessity to achieve greater knowledge and technology to gainfully manage these processes. Members of this committee and their colleagues both here and abroad are leaders in fields most closely aligned with these topics. The multistate efforts of this project are consistent with USDA national program goals, including protection of surface and ground waters, development of new instrumental and analytical techniques, and supporting a secure and sustainable agriculture. Efforts are also consistent with the goals of other programs and initiatives, including Sustainable Agriculture (SARE). The multiple-collaborations that characterize the proposed and past project activities can provide increased benefits relative to more focused yet restrictive approaches such as common field sites or identical experimental approaches carried out at different locations. While the proposed activities do in fact include some of these endeavors, committee members have a productive history of working together in small teams to develop complementary knowledge, tools, or approaches, sharing findings with each other, then incorporating and applying these new resources in re-configured teams to further advance the states of knowledge and technology. We view this as a more flexible, more synergistic, and (importantly) a more productive approach to multi-state, multi-agency, multi-investigator collaborative research. W-188 members have willingly worked very productively with each other and with those outside the committee, without the necessity to closely proscribe the nature of the interactions. The multistate committee structure provides an official and convenient means for periodic formal professional interactions among the entire group, which we all find extremely valuable. Objective 1 - To develop an improved understanding of the fundamental soil physical properties and processes governing mass and energy transport, and the biogeochemical interactions these mediate. Despite our best effort some physical properties and processes have eluded our understanding, especially at differing scales. The dynamics of water and solute transport are well understood at the lab or column scale, but we are unable to fully translate these processes to the field scale. Many current approaches are limited because of the enormous amount of data required to characterize flow of mass and energy at scales larger than a few square centimeters or meters. We will work to increase our understanding in several areas related to water, gas and temperature fluxes in near surface soils. These include, but are not limited to, preferential flow of water and/or solute, carbon sequestration, transport of reactive chemicals, scale- and time-dependent hydraulic properties, coupled heat and mass transport, effects of micro-gravity on plant growth systems for space exploration, root water uptake, and colloid transport or colloid assisted transport, and the transport of biologically active chemicals (hormones and other pharmaceuticals). Preferential and Unstable Flow Transport in highly heterogeneous materials such as structured soils and coarse sediments is often associated with preferential or unstable flow pathways. Transport models using the advection-dispersion and Richards equations often fail to simulate reality because large portions of the infiltration fluid may flow in discrete open pathways such as fractures or unstable fingers driven primarily by gravity (Kampf et al., 2002). Preferential water flow plays an important role in accelerated transport of contaminants toward ground water, resulting in unexpected concentrations or species found in drinking water and other wells. Preferential flow is not only found in natural soils, but also poses difficulties relative to heap leach mining operations common in Nevada and other western states (Kampf et al., 2002). In this process, irrigation of a reactive solution, typically cyanide, onto gold-bearing ore piled 10-100 m tall results in leaching of residual gold for extractive recovery. At mine closure, large amounts of rock material remain saturated with cyanide solution and effective rinsing of this material is an important step in environmental remediation. Our ability to predict the accelerated transport of contaminants through preferential flow paths is severely limited. We cannot yet forecast where, when, and at what velocity the contaminants will move. We can effectively predict accelerated movement only when we know that the pathways are present in the soil and at what matric potential(s) they are filled and flowing. We need new methods that can that can help us to predict where, when and at what velocity. The overwhelming and compelling advantage to doing this important work as part of the multistate effort will be the combined expertise. A second justification is the diverse set of circumstances that are present in the region. Biogeochemistry Soil physical properties and processes influence the rate and extent of most soil biogeochemical processes (Smith et al., 2002). Carbon dioxide emissions from agricultural systems are partially responsible for globally increased greenhouse gases (Cole et al., 1997). Agricultural soils under reduced tillage may have the potential to sequester C (West and Post, 2002; Six et al., 2002), but measurements to support this claim are scarce. The dynamic interactions between soil CO2 concentration, temperature, soil water, and root versus soil respiration are not well-understood (Bouma et al., 1997). Improved measurement and modeling methods are desperately needed to advance our understanding of C-cycling and to predict future changes in CO2 emission from agricultural operations. Seasonal transitions in arid climates control the form and mobility of nitrogen and can in turn impact terrestrial and aquatic ecology. There is a significant body of work indicating that seasonal transitions can play a critical role in affecting the mobility of nitrogen and eventual nitrogen status in seasonally dry ecosystems (Vitousek and Field, 2001; Vitousek and Field, 1999; Meixner and Fenn, 2003). Halogenated organic compounds are widely used chemicals. Some of these compounds such as the halogenated fumigants and pesticides, chlorinated solvents, polychlorinated benzenes, and chlorofluorocarbons have been found to contaminate the environment. These compounds are often resistant to natural environmental degradation, which has led to major efforts to prevent contamination and to remediate contaminated systems. There are relatively few safe and efficient methods to decontaminate porous systems such as soils. Research has being conducted using microorganisms to degrade these compounds, but such organisms are rare and it is often difficult to transport them to contaminated regions and to maintain their viability. Chemical oxidation reactions and physical extraction methods may be inefficient and/or cause additional harm to the environment. There is a great need to find new, more selective approaches to decontaminate halogenated organic compounds. Soil Physical-Microbial Relationships Despite the recognized importance of soil physico-chemical properties and processes to microbial community ecology, fundamental conceptual and experimental issues have hindered the close integration of soil physical principles with soil microbiology (Or, 2003). Members of the regional research committee will work together to quantify the primary physical influences on microbial habitats and activities in variably-unsaturated soils. Of particular interest are diffusional transport processes (Or, 2003; Qureshi et al., 2003), including diffusion within exopolymeric substances (EPS) and coupled diffusion among EPS and the soil matrix. The amount of soil water and its energy state are critical to soil microbial activity. Because different soil types will contain substantially different amounts of water at a given matric potential, and microbes congregate in aqueous habitats within soil pore space, it is the configuration of water rather than its energy state that will directly define the pathways and the extent of diffusion processes to and from soil organisms (Or, 2003). As soil pores drain and water films on solid surfaces become thinner, nutrients and wastes must follow a more tortuous path in diffusing to or from cells. Desaturation thus results in fragmentation of aqueous habitats, and increased tortuosity of liquid phase nutrient and metabolite mass transfer (Wraith et al., 2003). An ultimate goal is to infer controls on microbial community colonization of specific microsites in heterogeneous field soils. This information will be important to myriad agricultural and environmental applications. Soil Hydraulic and Thermal Flux Properties Research and quantification of soil hydraulic properties has been ongoing for decades. Recent advances in measurement abilities, consideration of spatial and temporal differences, and enhanced recognition concerning the critical importance of soil hydraulic properties to mass and energy transport advocate that this topic merits continued attention. Parameters describing soil hydraulic properties and root water uptake are important inputs for various models that simulate mass and energy transport in the vadose zone. Direct determination of these parameters in the laboratory or in the field is difficult and/or time consuming. Soil hydraulic properties are key to quantitatively describing soil water flow and chemical transport. Hydraulic properties of natural soils are scale-dependent, time dependent, and spatially variable. In agricultural soils, temporal changes in soil hydraulic properties are primarily caused by tillage (Or et al., 2000), clay content and clay mineralogy, and water and soil quality. Changes in soil volume and pore space induced by swell-shrink behavior of clays present a challenge to the development of predictive models for flow and transport, in particular to development of constitutive hydraulic functions. Such functions are important not only for design of man-made hydrologic barriers such as clay liners constructed for waste isolation but also for fluid flow predictions in porous media (Benson et al., 1994; Mitchell, 1993). Recent advances in pore scale modeling of fluid flow and liquid distribution in rigid angular pores have been developed through past collaboration of W-188 scientists. These consider both capillarity and adsorption (Tashman et al., 2003; Tuller and Or, 2001; Masad et al., 2000; Tuller et al., 1999; Or and Tuller, 1999) and provide the basis for a proposed multiscale-modeling framework in swelling soils. Pore scale processes (Tuller and Or, 2003) must be upscaled via appropriate schemes to represent sample and profile scale behavior while retaining the essential physical, chemical, and geometrical features operating at the microscale. Colloid transport Colloids are ubiquitous in soils, and play an important role in soil formation and contaminant fate and transport. Many contaminants in the vadose zone including pesticides, radionuclides, and viruses can associate with colloids, affecting the migration of these contaminants through soils. Some contaminants like viruses, bacteria, or other microorganisms are colloids themselves. We need to understand the mechanisms of colloid retention at different interfaces to make accurate predictions of colloid transport and to design effective management and remediation schemes to prevent soil and water contamination. Objective 2 - To develop and evaluate instrumentation and methods of analysis for characterizing mass and energy transport in soils at different scales. Several key areas of instrumentation and analysis are needed to provide sufficient accuracy in our predictions of mass and energy transport. Key areas to be investigated under this proposal are given below. Improved and Multifunction Measurement Devices In agriculture, ecology, hydrology, global change, and related sciences, there is a pressing need for improved instrumentation to augment the general understanding of water flow, nutrient/chemical transport, and heat transport processes in rooting zones, the vadose zone, and ground water (Hopmans et al., 1999). As during past years, effective new laboratory and field techniques to measure and monitor soil environmental parameters will be developed as part of this project. Evapotranspiration Evapotranspiration (ET) is the water flux through soil and plant systems to the atmosphere. Understanding spatial and temporal variations of ET is important for resource management, precision irrigation, controlling water fluxes carrying potential pollutants to groundwater, precision agriculture, and on larger scales for weather modeling and forecasting, carbon sequestration, and other environmental studies (Bastiaanssen et al., 1998ab; Peters-Lidard et al., 1997, 2001). Unfortunately, ground truth technologies have not kept abreast of remote sensing for estimating and measuring variations in ET (Allen et al., 2002). Hydraulic Property Instrumentation and Models Accurate measurement of soil hydraulic conductivity requires careful and often time-consuming attention on the part of scientists or practitioners. Most of the labor involved with steady state methods consists of a feed-back between the operator, the experimental settings, and the degree of steady state attainted. By automating the actions necessary for attaining steady-state, the measurements become nearly operator-independent and potentially much faster. As a result, the number of measurements will increase thereby increasing the detail of the measured hydraulic conductivity characteristics. Pedo-transfer functions (PTF) are often needed in applications where critical soil properties are not known or not reliable. A common example is where soil survey information is used in landscape-scale applications. Critical questions still remain about whether PTF predictions might exhibit regional bias and whether they can account for spatial variability and scale effects. Objective 3 - To develop and evaluate scale-appropriate methodologies for the management of soil and water resources. Computer capabilities have evolved to a point where it is now possible to use multi-dimensional physically based hydrologic models to study spatial and temporal patterns of mass and energy flow in the vadose zone. However, so far these models have received limited attention because of their computational, distributed input, and flux parameter requirements. Global optimization algorithms may enable exploration of the utility of various mechanistic models with differing complexities to analyze the transport of salts and nutrients in irrigated areas, at spatial scales as large as a watershed. Numerical simulation of dissolution/precipitation reactions in soils and other porous media do not strongly couple these reactions to the fluid flow domain. For example, precipitation of calcite in soils can result in plugging of pores and significant drop in hydraulic conductivity. However, typical reactive transport models contain only a porosity adjustment when precipitation reactions occur and, at best, a simple relationship between porosity and saturated conductivity only. Researchers will use tools developed in multiple states to assist this effort by comparing the hydraulic properties of soils to those of an evolving (through precipitation reactions) in volcanic tuff matrix. Surface and ground waters in southwestern states are often impaired by nutrient runoff and leaching from irrigated agriculture. While actions to enhance the quality of surface waters will be addressed through TMDLs, degradation of aquifers under agricultural lands is a growing problem. Adoption of best management practices (BMPs) is the most effective way to prevent surface and ground water degradation from agricultural operations. However, an assessment of potential management problems should be conducted before BMPs are chosen or implemented. Several nitrogen indices have been developed to help growers assess nitrate leaching potential, but they are not directly applicable to irrigated agricultural lands in the Southwest. Zone soil sampling may make precision farming practical in the Northern Great Plains, but defining zones is currently subjective. Comparing and evaluating methods of zone nitrogen determination will make zone development more objective and automated. This should include evaluation of sampling methods, determining ways to combine information from different sampling methods, automation of nutrient zone boundary determination, evaluation of water quality impacts from precision agriculture, and exploration of economic and environmental consequences of different zone methods with respect to nitrogen fertilization. Field-scale water content and soil property mapping approaches are needed to provide linkages between in-field measurements and remotely sensed data for improved resource management.
Related, Current and Previous Work
Some other multistate projects also focus on related soil- and water-quality issues. Projects W-82, W-128, W-170, W-190, and W-195 focus on soil or water conservation and quality management. Projects outside the western region, including NC-174, NC-218, NC-1022, IEG-073, S-271, S-280 evaluate farm, water, and/or soil management practices. Though some of these projects share a focus on similar subject matter, there is little or no duplication of these projects with past W-188 or proposed W_TEMP1011 core activities. IEG-073 probably has the closest relationship to W-188, and we recognize that occasional shared meetings with this group of southern and southeastern colleagues would be mutually beneficial. It should be noted that we do routinely interact with many colleagues from IEG-073 and other committees at national meetings and other venues. Participants in that group have focused primarily on the development of soil classification systems, linking mapped soil properties to solute transport, and related goals. The W-188/W_TEMP1011 group is focused on understanding mass and energy transport processes in soils, at multiple time and space scales, and on developing related methods and technologies to advance applications of such knowledge. Many of the experimental research efforts in the past decades on flow and transport processes in field soils are attributed to the seminal studies of Nielsen et al. (1973) and Biggar and Nielsen (1976), both of whom were members of past versions of this multistate research committee. Their research produced several new directions in soil science (Mulla et al., 1998). Their findings stimulated the transition in solute transport research from an emphasis on the laboratory to field-scale experimentation, and brought to light the inherent field soil heterogeneity, and its tremendous influence on field-scale flow and transport. In addition, their papers suggested applying stochastic approaches to describe field-scale water and solute fluxes. In previous projects, large-scale field experiments were established to test theories of water (Hills et al., 1991) and solute transport (Schulin et al., 1987; Ghodrathi and Jury, 1990). These field experiments confirmed that soil heterogeneity controlled large-scale flow and transport, including preferential flow, and confirmed the difficulty of applying deterministic modeling to predict field-scale mass and energy transport processes. Hence, stochastic approaches were developed, which can characterize field-scale transport using scaling (Bresler and Dagan, 1981), Monte-Carlo analysis (Amoozegar-Fard et al., 1982), stochastic-convective stream tube modeling (Dagan and Bresler, 1979; Jury et al., 1986; Jury and Roth, 1990; Toride and Leij, 1996) and stochastic-continuum modeling using an ensemble-averaged transport equation with parameters described by random functions (Russo and Dagan, 1991). Prediction of large-scale flow problems has followed similar lines, with initial attempts to characterize flow regimes by deterministic modeling. Although studies such as that of Hills et al. (1991) showed a qualitatively acceptable comparison between field-measured and predicted water contents using the deterministic approach, other studies have shown the need for either distributed physically-based modeling (Loague and Kyriakidis, 1997) or stochastic modeling (Famigllietti and Wood, 1994) at the watershed scale. However, flow or transport processes have been shown to be scale-dependent, hence requiring scale-dependent parameterizations. For example, Merz and Plate (1997) pointed out the difficulty of applying scale-effective soil parameter values for scale-dependent processes. The scale-dependency of water flow through porous systems was also discussed by Dooge (1997), who hypothesized that physical laws such as the Navier-Stokes and Darcy equations are appropriate only for specific spatial scales. The general theme of the previous 5-year W-188 multistate project addressed improved characterization and quantification of flow and transport processes in soils, which focused on the development of new approaches, instrumentation and data analysis methodologies to characterize flow processes in field soils. New experimental methodologies were developed that, in combination with large-scale measurements, process-based modeling and data analysis techniques provided the framework to continue a focus on mass and energy transport in soils. New or improved experimental and data analysis approaches included those for measurement of soil water, soil heat transport, infiltration and solute breakthrough, application of geostatistical and modeling techniques to characterize field-scale transport, the use of pedotransfer functions and neural network procedures, and improved inverse parameter procedures for estimation for the unsaturated hydraulic parameters. These methodologies, including remote sensing techniques, will be applied to improve soil water management practices to reduce erosion and improve surface and ground water quality. However, the critical importance of mass and energy transport in soils to agricultural and environmental applications, the sheer magnitude and scope of an emphasis on flow and transport processes, and the continually evolving states of knowledge and technology, together argue that we continue with a revised focus in this area. The revised objectives of this multistate project will address fundamental issues, will evaluate new or improved instruments, methodologies and data analysis techniques, and will focus on extrapolation of local-scale parameters and processes to larger spatial scales in the landscape, such as agricultural fields and watersheds. Relationships between soil/atmosphere mass and energy transport processes across spatial and temporal scales are needed to manage water and chemicals in agriculture, to manage waste disposal sites, to quantify soil solution changes near the land surface, and to address many additional land use issues. Experimental results and simulation models will be applied at a variety of scales, and are intended to solve basic and applied problems from point (plot, field) to basin (watershed, region) scales. The revised project will combine expertise from member states to develop new knowledge and methods that will help the USDA provide truly sustainable agricultural and natural resources programs that will benefit current and future generations.
Objectives
-
To develop an improved understanding of the fundamental physical properties and processes governing mass and energy transport, and the biogeochemical interactions these mediate.
-
To develop and evaluate instrumentation and methods of analysis for characterizing mass and energy transport in soils at different scales.
-
To develop and evaluate scale-appropriate methodologies for the management of soil and water resources
Methods
Objective 1 - To develop an improved understanding of the fundamental soil physical properties and processes governing mass and energy transport, and the biogeochemical interactions these mediate. Preferential and Unstable Flow Several groups will study different aspects of preferential flow and transport. PNNL (WA) will collaborate with AZ, WA, and ID in improving our ability to describe subsurface flow and transport in heterogeneous systems. Anisotropic soil properties will be estimated from measured soil physical and hydraulic properties and knowledge of the geologic and engineering features of buried waste sites and incorporated in the transport analysis. The analytical element methods (Warrick and Knight, 2002; Furman et al., 2002) that have been employed to look at flow around buried objects will be compared to numerical methods using 3-D simulators to quantify contaminant transport in areas where there are buried tanks and other large obstructions (White and Oostrom, 2004). Collaborative research among CA will quantify the occurrence and the extent of unstable flow in soils. Members will experimentally study hypothesized relationships between finger appearances (frequency, size, volume, speed, tip-length and final depth of fingers) and system parameters (influx ratio q/Ks, soil structure/texture and initial water content). Experiments will evaluate unstable flow in different soils at various initial water contents and for a wide range of infiltration and redistribution rates so as understand the extent of unstable flow based on the existing work of Baker and Hillel (1990), Glass et al. (1989), Selker et al. (1992), Weisbrod et al. (2000) and Wang et al. (1998a, 2003a, 2003b, 2003c). They will derive physically based mathematical criteria for predicting the onset of unstable flow during infiltration and redistribution. The linear instability criteria of Wang et al. (1998b) will be tested by measuring unstable flow of different fluids (water and NAPLs) in the vadose and water-saturated zones. These criteria will also be generalized within a stochastic framework using the approach of Chen and Neuman (1996) to evaluate finger flow formation in heterogeneous media. Finally, numerical models based on experimentally validated key mechanisms (Jury et al. 2003, Wang et al. 2003b, Nieber 1996, Eliassi and Glass 2002) will be developed. Biogeochemistry California members will conduct much needed research to couple biological processes with soil physical properties. They will combine model development and experimental verification to elucidate the dynamic interactions between root respiration, soil respiration and environmental controls governing CO2 emissions from agroecosystems. Objectives are to determine whether inverse modeling can be applied to partition total CO2 fluxes into root and soil respiration, and to develop and test laboratory and modeling procedures for field conditions. Isotopic data will be used to improve simulation models that can predict the impacts of tillage on soil C storage. California will also continue work concerning hydrologic controls of biogeochemical processes (James, 2000). This focuses on how seasonal transitions in arid climates control the form and mobility of nitrogen and its impact terrestrial and aquatic ecology (Vitousek and Field, 2001; Vitousek and Field, 1999; Meixner and Fenn, 2003). They will characterize how seasonal transition from dry to wet influences biogeochemical processes and efficacy of models such as CENTURY (Del Grosso et al., 2000; Parton et al., 1987; Parton et al., 1988). A final emphasis will be to evaluate a recently developed mass transport equation (Yates and Enfield 1988, 1989) to describe the fate and transport of pesticides in the presence of nucleophilic materials (Gan et al, 1998, 2002; Zheng, et al. 2003, 2004) and other sulfur-containing compounds. This approach will be tested using experimental data obtained in saturated laboratory soil columns, and will be simulated using numerical and analytical solutions. Once the methodology has been tested, predictive technology will be developed to assist in remediation of contaminated environments. Soil Physical-Microbial Relationships Montana and Connecticut will use a combination of field and laboratory experiments, measurements and modeling, to evaluate microbial community responses to soil physical-chemical conditions. They will concentrate their efforts on diffusional transport processes (Or, 2003; Qureshi et al., 2003), including diffusion within exopolymeric substances (EPS) and coupled diffusion among EPS and the soil matrix. They will specifically evaluate how changes in particle size and wetness affect soil microbial community composition by looking at different pore-scale water configurations, and the relative magnitudes of microbial community selection pressure due to different sand size fractions (Wraith et al., 2003), wetness (Treves et al., 2003), and nutrient solutions. Soil Hydraulic and Thermal Flux Properties Combined efforts of members in multiple states will enhance our understanding of soil water and heat flow. The IA group will be looking at coupled heat and water transfer (Philip and de Vries, 1957) in an effort to incorporate newly important considerations of mass and energy transport (Parlange et al., 1998), including thermally-driven advection of soil atmosphere and soil wettability (Bachmann et al., 2001.).A network model (Berkowitz and Ewing, 1998) of coupled heat and water transfer in soils will be developed, facilitating examination of which properties and processes are important under which conditions, furthering our understanding and focusing the development of improved continuum models in the future. Changes in soil volume and pore space induced by swell-shrink behavior of clays will be upscaled to represent sample and profile scale behavior while retaining the essential microscale physical, chemical, and geometrical features, as a collaborative focus of ID and CN. In addition, they will constrain the overall sample volume as a function of water content (or potential) based on one of several universal relationships between hydration state and sample volume such as that of Giraldez and Sposito (1983). Connecticut and Idaho also propose to model dynamic changes in soil surface intake properties due to formation and closure of crack networks using concepts similar to those in the recently developed VIMAC (Vertical Infiltration through a Macroporous Clay) model by Greco (2002). Geometrical inputs regarding crack network, apertures, depths and connectivity from Chertkov (2000) and Chertkov and Ravina (2001) are considered, then an upscaled hydraulic conductivity function for the clay soil matrix will be applied, and finally the fractured porous medium hydrodynamic model of Or and Tuller (2003) will be modified to derive constitutive relationships for evolving surface crack networks leading to improvements over the VIMAC model in describing infiltration rates into swelling soils with surface cracks. Colloid transport Committee members will investigate mechanisms of colloid retention in unsaturated soils via a combination of experimental, theoretical, and numerical approaches. A common set of colloids and soil materials will be used, and the different experiments are designed to complement each other. We will use surface thermodynamics to calculate equilibrium free energies of colloid attachment to liquid-gas interfaces. We will determine surface thermodynamic properties of colloids, such as water-solid contact angles, water-solid interfacial tensions, electrophoretic mobility, specific surface charge, and specific surface areas, to calculate free adhesion energies of colloid attachment to liquid-gas interfaces (WA). Colloid interactions at liquid-gas interfaces and at the solid-liquid-gas contact line will be investigated using bubble-column techniques (Wan and Tokunaga, 1998) and micromodels (Wan and Wilson, 1994, Or and Tuller, pers comm). We will use a 2-m long bubble column to study the colloid attachment to air bubbles and will determine colloid-gas interface attachment coefficients. Transparent micromodels will be used to optically examine colloid attachment to the interfaces. We will use both closed and open micromodel techniques with stationary and moving liquid-gas interfaces and at the solid-liquid-gas contact line. At DE column experiments using washed colloid-free standard sand will be conducted under saturated and unsaturated flow regimes, using previously established protocols (Jin et al, 2000, Bradford et al., 2003, Cherrey et al., 2003). Steady-state as well as transient (drainage and imbibition) flow regimes will be employed (CA, WA, DE). Using the pore scale model of Tuller and coworkers (Or and Tuller, 1999, Tuller et al., 1999, Or and Tuller, 2000), we will calculate liquid-gas interfacial areas as function of water saturation of our porous media, and will determine the liquid-gas interfacial configuration in the porous medium. The liquid-gas interfacial area can be used to better define colloid attachment parameters, and elucidate physical colloid retention mechanisms (ID, CN). Colloid breakthrough curves and final spatial distributions from unsaturated column experiments will be analyzed using HYDRUS-1D (Simünek et al., 1998; CA). Different colloid retention mechanisms obtained from the experiments will be incorporated into HYDRUS-1D and tested with model simulations following an approach similar to the ones used by Chu et al., 2001 and Simünek et al., 2002. Micro-gravity Utah, Connecticut, Idaho, and Kansas will study the influence of the gravity field on retention and mass and energy transport within porous media. Fundamental properties to be studied include water retention and gaseous diffusion (Jones et al., 2003), two critical processes often found to be the most limiting for plant growth in space. Substrate-water retention data from a microgravity environment will provide a basis for modeling these processes and will suggest whether or not earth-based models such as the Richards equation are applicable to these conditions. Objective 2 - To develop and evaluate instrumentation and methods of analysis for characterizing mass and energy transport in soils at different scales. Improved and Multifunction Measurement Devices At CA and UT the water content/permittivity relationship will be experimentally quantified for various materials, and a coherent physical model developed that will enable us to increase the utility of these instruments in clayey or saline soils. Montana and Connecticut are developing a non-invasive electromagnetic method to estimate soil specific surface area using TDR. They will quantify the contributions of Maxwell-Wagner fragmentation polarization to electromagnetic measurements at a wide range of frequencies, and for diverse porous media and salinities. Montana will work with committee members and a Swedish colleague towards developing a small TDR-based matric potential sensor having greater effective range than existing methods. Utah will work to identify a hierarchy of pore- and sample-scale processes affecting dielectric and conductive responses such as constituent shape, diffuse double layer (DDL) characteristics, interfacial interactions, and measurement frequency. The development of pore- and sample-scale geometrical and electrochemical models describing the dielectric permittivity and bulk electrical conductivity of saline and heavy textured soils will improve water content determination using EM techniques. California, IA, KS, and MN will continue developing multi-function heat pulse probes for use under field conditions. California will combine field measurements with recently-developed numerical simulation models that couple the highly nonlinear multi-dimensional unsaturated water flow, nutrient, and heat transport equations with plant water and nutrient uptake using inverse modeling analysis techniques. Theoretical sample-volume analysis tools based on potential new sensor geometries will be developed in AZ and UT. The shared goal is to develop models that also take into account soil compaction effects and composite probe constituents. These tools will increase the efficacy of new sensor designs for water and salt contents. Robust sensors will then be developed by UT and CA for integrated vehicle mounted sensor platforms for mapping soil water content, temperature, EC, micro-elevation and position for improved field-scale salinity variability mapping. Mass and Energy Flux Instruments and Methods PNNL (WA) in collaboration with IA, KS, CA, MN, NV, and others will compare recently developed water flux meters (Brye et al. 1999; Gee et al. 2002) with equilibrium tension lysimeters and other water flux sensing devices under agricultural, turf grass and waste management settings. Tests of water flux measurements in the field using thermal probe methods will be initiated by KS, Japan and CA and these data will be compared with more conventional lysimeter measurements. Comparison will be made over a range of environments and drainage fluxes ranging from less than 10 to over 1000 mm/yr. Laboratory experiments measuring heat and water fluxes in sealed and unsealed soil columns will be performed by collaborators in IA, KS, MN, and CA. Heat pulse sensors similar to Ren et al. (1999; 2000) will be used to measure soil thermal properties and water content, allowing us to subject each soil column to a wide range of boundary conditions for model calibration and validation. A heat pulse technique for measuring water flux (Wang et al., 2002) will be reevaluated and refined under saturated and unsaturated steady-flow conditions. We will examine effects of soil texture and wettability, water content, mean temperature, direction of thermal gradient, and influence of fluctuating boundary conditions on coupled heat and water movement in sealed soil columns. We will then study effects of these on evaporation from open soil columns. This work will set the stage for future field validation and application of this technique for measuring simultaneous soil water and heat flow. Hydraulic Property Instrumentation and Models In support of physical model development (ID, CN, WA) the ID group will investigate the effects of electrolyte type and concentration, clay content, and porosity on mesopore evolution using a modified volume change apparatus. Maximum water ratios determined for clay-sand mixtures will be used to constrain sample-scale volume expansion of clay soils and mixtures. Application of constraining stresses (control of initial cell pressure and vertical strain) allows observation of volume expansion under confinement. Saturated and unsaturated hydraulic conductivities will be measured using an automated technology flexible wall permeameter. Using clay-sand mixtures will allow control of media parameters required to test the pore geometry models. The Rosetta pedo-transfer function software will be improved by CA. First new models that are calibrated on currently available databases will be incorporated and followed by data mining using the NRCS soil characterization database. Resulting models will be combined into Rosetta v.2.0. Version 1.0 only considers the Mualem-van Genuchten equations (van Genuchten, 1980) so additional soil water property models to be incorporated include those by Brooks-Corey (1964), Campbell (1974), Rossi-Nimmo (1994), Kosugi (1996), and Vogel et al. (2001). To address whether Rosetta predictions exhibit regional bias and whether they can account for spatial variability and scale effects, we will develop PTFs based on data from local projects with relatively uniform experimental procedures (e.g., Hanford Waste Disposal Area), from regional-scale hydrology projects (e.g., NASAs 1997 Southern Great Plains Experiment), and from other national and international databases. Results will allow assessment of whether Rosetta provides suitable predictions at different scales and geographies, and whether it is worthwhile to develop dedicated PTFs for individual regions or nations. If the predictions are not sensitive to scale and geographical origin, it may eventually meet the hydraulic data requirements of regions or countries that do not have enough data or resources to establish their own local PTFs. A flux-head steady-state measurement method that alleviates limitations of other steady state methods will be developed by CA, and evaluated by other states. The flux-head method will be radically automated by combining it with a precision syringe pump that can attain both high and low flux densities (104 and 10-4). Improved measurement capabilities will be applied to practical problems by committee members from multiple states. Objective 3 - To develop and evaluate scale-appropriate methodologies for the management of soil and water resources. Simulation Modeling To explore the applicability of inverse methods to estimate required spatially distributed vadose zone properties in the landscape (Hopmans and Schoups, 2004), CA proposes using a global optimization scheme (Vrugt et al., 2003; 2004). They will investigate the utility of mechanistic models of differing complexity to analyze the transport of salts and nutrients in irrigated areas, at spatial scales as large as a watershed. This work will develop tools to help determine model flaws and suggest potential improvements thus making environmental models more reliable for hypothesis testing, forecasting, and policy-making. The research will aid in evaluating optimal levels of watershed model complexity, the benefits of distributed models of water quality, and how to incorporate multiple fluxes into evaluations of how well models perform. Such questions were identified as important challenges for the science of hydrology (James, 2000). Nevada and CA will develop appropriate pore scale models of precipitation/dissolution that can be used to modify the retention properties and hydraulic conductivity functions of porous materials. The work will focus on reactions expected to occur adjacent to spent nuclear fuel in volcanic tuff using the numerical solver TOUGH-REACT (Xu et al., 2003). Data from the Rosetta database will be used to assist this effort by comparing the hydraulic properties of soils to those of an evolving (through precipitation reactions) volcanic tuff matrix. Spatial Sampling and Statistics Precision agriculture will be aided by collaboration among scientists who work with zone soil sampling (UT, CA), statistical modeling and analysis (CA), modeling sensor design (CSIRO) and field-scale mapping of soil properties (TX). A hierarchy of time domain transmissometry (TDT) and time domain reflectometry (TDR) probe designs for use with stop and go and potentially on the go measurements will be evaluated. Models will be developed accounting for secondary effects such as soil compaction around sensors in order to accurately determine soil water content and EC. Simultaneous spatial mapping of water content and EC in agricultural soils will be addressed by CA. They will monitor changes in soil salinity and boron in selected fields of the Broadview Water District in the western San Joaquin Valley of California, estimate field-scale boron and salt mass balances, calibrate HYDRUS on the field data, and subsequently use HYDRUS to design optimal shallow groundwater management practices. A secondary purpose is to evaluate the root and crop growth, root water uptake, and multiphase flow routines being developed under Objective 1 for HYDRUS. Other scientists will investigate the relationships between sample support, sample grid scale, and field size and how these interact with the spatial structure of variability in soil properties. This work is primarily focused on improving nutrient mapping and developing management methods that incorporate spatial uncertainty in the decision making process.Measurement of Progress and Results
Outputs
- Generation of new information on mass and energy transport processes in soils at spatial and temporal scales appropriate for effective resource management.
- Generation of new knowledge effecting the environmental impacts of soil, water and chemical-based agricultural practices.
- Information concerning the effects of colloids on the enhanced transport of chemicals and biological agents.
- New instruments for direct measurement of water flux, chemical flux and energy flux.
- Development of model components that reflect new knowledge of transport mechanisms including colloid transport and preferential flow.
- Output6;Methodologies including computer and analytical models, for improved prediction of mass and energy transport and resource management; Output7;Information on the effects of micro-gravity on soil physical and properties and plant growth; Output8;This project will continue to provide real time collaboration between committee members and soil scientists, engineers, and others around the world through the committee web site maintained by Dr. Casey at North Dakota State University; Output9;This research project will continue to engage in research that will provide answers to short-term problems affecting US Agriculture and environmental protection in the areas of salinity, water quality, solute transport, evapotranspiration, soil water and chemical transport properties and other areas. Our research will focus on long-term problems such as identification and characterization of the dominant processes affecting the transport of mass and energy through soils and other porous media at various management scales. The continuing project is unique among interstate research committee efforts because rather than collaborate on a single focused objective, many collaborative projects are conducted simultaneously by organized groups of participating members and others within the overall objectives of the project. These extensive collaborations are established and maintained through the organizational structure of the continuing interstate research project. This strategy is inevitable given the diversity of problems that need to be addressed, but is also highly desirable, as information gained from the specific collaborations are shared with the global soil and agricultural sciences communities.
Outcomes or Projected Impacts
- Increased knowledge of the fundamental processes governing water and chemical behavior in soils and other porous media at "problem-dependent" spatial and temporal scales. This new knowledge will constitute fundamental principles for the next generation of scientists, and as outreach material having immediate relevance for agricultural extension professionals.
- Reduced environmental impacts of agricultural practices on shallow groundwater and surface water quality.
- Assistance to producers in determining the optimal timing and amount of chemical, fertilizer, and irrigation application in order to sustain high agricultural productivity and profitability that is consistent with sustainable stewardship of natural resources .
- Newer and less expensive instruments and real-time data analysis systems for monitoring on-site management variables and for providing more timely assessment for management decisions.
- User-friendly management models for use at the county extension agent or farm manager level for increased management of risk and environmental impacts of alternative agricultural practices.
- Outcome/Impact6;New or improved sensors and measurement systems for commercial application; Outcome/Impact7;Lasting scientific cooperation among states and institutions leading to enhanced opportunities for more effective management of soil, water, and environmental resources; Outcome/Impact8;Organization of multiple sessions at professional meetings, workshops and conferences with themes that are included in this proposal. For example, two sessions at the 2004 ASA/CSSA/SSSA meeting, as well as the upcoming Kirkham Conference focus, will address issues closely related to this project. Committee members are key organizers of these conferences
Milestones
(2005): Conduct controlled water flux experiments under lab and field conditions to evaluate the soil properties and mechanisms causing preferential flow of water and chemicals, during years 1, 2 and 3. Conduct lab and field experiments to assess design criteria for new instruments to measure mass and energy fluxes during years 1, 2, and 3. Develop theoretical and conceptual models for colloid-soil-air interface interactions, soil shrink-swell processes, and finger formation during unstable water flow during year 1 and 2. Conduct micro-gravity experiments during years 1, 2, and 3(2006): Develop numerical root growth and root water uptake model, global optimization inverse modeling approach to determine required spatially distributed soil properties in the landscape, calibrate available numerical water flow model for spatial nutrient modeling and network model of near soil surface energy balance, during years 2, 3, and 4. Conduct colloid transport experiments during years 2, 3, and 4. Construct multi-sensor field instruments for measuring water content, solute concentrations, and soil temperature for precision agriculture and variability mapping, during years 2 and 3
(2007): Conduct controlled environment experiments to quantify the response of soil microbial communities to soil physical and chemical conditions, during years 3 and 4. Make comparisons between the newly developed water flux instrumentation under varied representative field conditions, during years 3, 4, and 5. Experimental verification of models for pesticide transport, root growth and water uptake, preferential and unstable flow, infiltration into a shrink-swell soil and near surface energy and mass balance, during years 3, and 4
(2008): Evaluate modeling concepts for preferential flow, micro-gravity, near surface transport of mass and energy and advection of soil atmosphere, seasonal transition effects on nitrogen mobility and carbon sequestration, during years 4 and 5. Test multi-sensor field instruments, during years 4 and 5.
(2009): Complete and/or continue multiple ongoing and new activities. Continue to summarize and extend important findings. Review and ensure effectiveness of outreach and commercialization efforts. Prepare proposal for 5-year project renewal.
Projected Participation
View Appendix E: ParticipationOutreach Plan
The members of W-188 comprise a group of dedicated soil, water and environmental scientists and engineers who excel in the communication of their research findings. All members of the W-188 project are active participants in soil and environmental research at universities and federal facilities throughout the country. They provide leadership in this vital area through undergraduate and graduate instruction, as well as by mentoring post graduate, graduate and undergraduate research. A number of our members conduct extension workshops and software classes to provide thorough understanding of transport concepts, as well as cutting edge results. All W-188 members regularly publish their findings in top-tier, peer-reviewed journals, targeting both science and engineering communities. W-188 members are also active participants and presenters at many and varied professional society international/ national/regional meetings, as well as at the major workshops and symposia sponsored by these societies. W-188 members are frequent initiators of major workshops and symposia. Members serve on journal editorial boards and as ad hoc manuscript reviewers, and therefore, help to enhance the quality of published soil, water and environmental research by others. Members serve on competitive grant review panels of federal and regional funding entities, and as peer reviewers for domestic and international competitive grant proposals. Several new or improved instruments and new computer and analytical models have been pioneered by committee members; many have been developed commercially and are extensively used in agricultural sciences and management. We intend to continually emphasize development and outreach of such tools. Results of our work will also be made available through the annual project report, the project website (http://www.soilsci.ndsu.nodak.edu/W188/), periodic joint meetings with related multi-state research and/or coordinating groups, and through the international reputations and professional visibility of participants.
Organization/Governance
The current W-188 (proposed W_TEMP1101) multistate research committee consists of members representing State Agricultural Experiment Stations, the USDA-ARS, National Laboratories, and other research units. Visiting scientists commonly participate along with member hosts. Officers of W_1101 will be the Chairperson and Secretary. The Secretary will be elected each year at the annual meeting, and will advance to Chairperson the following year. For 2004 the committee officers will be Chairperson Yan Jin (DE), and Secretary Mark Oostrom (PNNL, WA). The chairperson may appoint members to serve on subcommittees for technical or administrative duties. Meetings will be called each year by the administrative officer, and the current Secretary will be responsible for making local arrangements. Committee meetings are generally held in Las Vegas, NV during early January. At the annual meetings research accomplishments will be reviewed, new opportunities and recommendations for multi-state coordination discussed, and strategies for maximizing the impact of committee productivity will be reviewed. The W-188 committee and its precursors have had strong historical participation at the annual meeetings (35-40 attendees per year), with new members inducted each year to ensure longevity and infusion of fresh perspectives. Most or all current W-188 members, and some new members, have indicated a strong desire to participate in the new project.
Literature Cited
Ashby, J., E. Houthoff, S.J. Kennedy, J. Stevens, R. Bars, and F.W. Jekat, P. Campbell, J. Van Miller, F,M Carpanini, and G. Ransall. 1997. The challenge posed by endocrine disrupting chemicals. Environ. Health Perspect. Environ. Health Perspect. 105, 164-169. Averner, M. M. 1989. Controlled Ecological Life Support System. p.145-153. In D. W. Ming and D. L. Henninger (ed.) Lunar Base Agriculture. American Society of Agronomy, Madison, WI. Bachmann, J., R. Horton, and R. R. van der Ploeg. 2001. Isothermal and nonisothermal evaporation from four sandy soils of different water repellency. Soil Sci. Soc. Am. J. 65: 1599-1607. Baker, R.S. and D. Hillel. 1990. Laboratory tests of a theory of fingering during infiltration into layered soils. Soil Sci. Soc. Am. J. 54:20-30. Benson, C. H., F.S. Hardianto, and E.S. Motan, 1994. Representatitive specimen size for hydraulic conductivity assessment of compacted soil liner., Hydraulic conductivity and waste contaminant transport in soil., ASTM STP 1142, D. E. Daniel and S. J. Trautwein, Eds., ASTM, pp., 3- 29. Berkowitz, B. and R. P. Ewing, Percolation theory and network modeling applications in soil physics, Surv. Geophys. 19:23-72, 1998. Bingham, G. E., M. A. Levinskikh, V. N. Sytchev, and I. G. Podolsky. 2000. Effects of gravity on plant growth. J. Gravitat. Phys. 7:5-8. Bouma, T.J., K.L. Nielsen, D.M. Eissenstat, and J.P. Lynch. 1997. Estimating respiration of roots in soil: Interactions with soil CO2, soil temperature and soil water content. Plant Soil 195:221-232. Bradford, S. A. , Simunek, J., Bettahar, M., van Genuchten, M.T. and Yates, S. R., 2003. Modeling colloid attachment, straining, and exclusion in saturated porous media. Environ. Sci. Technol., 37:2242-2250. Bugbee, B. G. and F. B. Salisbury. 1989. Controlled environment crop production: Hydroponic vs. lunar regolith. p.107-130. In D. W. Ming and D. L. Henninger (ed.) Lunar base agriculture: soils for plant growth. American Society of Agronomy, Madison, WI. Casey, F.X.M., G.L. Larsen, H. Hakk, and J. S?im?unek. 2003. Fate and transport of 17b-estradiol in soil-water systems. Environ. Sci. Technol. 37, 2400-2409. Casey, F.X.M., H. Hakk, J. S?im?unek, and G.L Larsen. 2004. Fate and Transport of Testosterone in Agricultural Soils. Environ. Sci. Technol. 38, (in press). Cherrey, K.D., Flury, M. and Harsh, J.B., 2003. Nitrate and colloid transport through coarse Hanford sediments under steady-state, variably-saturated flow. Water Resour. Res., 39:1165, doi:10.1029/2002WR001944. Chertkov, V.Y., 2000. Modeling the pore structure and shrinkage curve of soil clay matrix, Geoderma, 95:215-246. Chertkov, V.Y., and I. Ravina, 2001. Effect of interaggregate capillary cracks on the hydraulic conductivity of swelling clays. Water Resour. Res., 37(5):1245-1253. Chu, Y., Jin, Y., Flury, M. and Yates, M.V., 2001. Mechanisms of virus removal during transport in unsaturated porous media. Water Resour. Res., 37:253-263. Colborn, C.C., F.S. vom Saal, and A.M. Soto. 1993. Developmental effects of endocrine-disrupting chemicals in wildlife and humans. Environ. Health. Perspect. 101, 378-384. Cole, C.V., J. Duxbury, J. Freney, O. Heinemeyer, K. Minami, A. Mosier, K. Paustian, N. Rosenberg, N. Sampson, D. Sauerbeck, and Q. Zhao. 1997. Global estimates of potential mitigation of greenhouse gas emissions by agriculture. Nutr. Cycl. Agroecosys. 49:221-228. Dagan, G., and V. Cvetkovic. 1993. Spatial moments of a kinetically sorbing solute plume in a heterogeneous aquifer. Water Resour. Res. 29:4053-4061. Del Grosso, S. J., W. J. Parton, A. R. Mosier, D. S. Ojima, A. E. Kulmala, and S. Phongpan, General model for N2O and N2 gas emmisions from soils due to denitrification. 2000. Global Biogeochemical Cycles, 14:1045-1060. Derjaguin, B.V., N.V. Churaev, and V.M. Muller, 1987. Surface Forces, Consult. Bur., New York. Dutcher, F. D., E. L. Hess, and T. W. Halstead. 1994. Progress in plant research in space. Adv. Space Res. 14:159-171. Eliassi, M., and R. J. Glass. 2002. On the porous-continuum modeling of gravity-driven fingers in unsaturated materials: Extension of standard theory with a hold-back-pile-up effect, Water Resour. Res., 38(11), 1234, doi:10.1029/2001WR001131. Gan, J., Yates, S.R., Becker, J.O. and Wang, D. 1998. Surface amendment of fertilizer ammonium thiosulfate to reduce methyl bromide emission from soil. Environmental Science and Technology 32:2438-2441. Gan, J., Wang, Q., Yates, S.R., Koskinen, W.C. and Jury, W.A.2002. Dehalogenation of Chloroacetanilide Herbicides by Thiosulfate Salts. Proceedings of National Academy of Sciences of The United States of America 99:5189?5194. Giraldez, J.V., and G. Sposito, 1983. A general soil volume change equation: I. Effect of load pressure. Soil Sci. Soc. Am. J., 47:422-425. Glass, R.J., T.S. Steenhuis, and J.Y. Parlange. 1989b. Wetting front instability, 2. Experimental determination of relationships between system parameters and two dimensional unstable flow field behavior in initially dry porous media. Water Resour. Res. 25:1195-1207 Grant, C. D., P. H. Groenevelt, and G. H. Bolt, 2002. On hydrostatics and matristatics of swelling soils. In: Environmental Mechanics: Water, Mass and Energy Transfer in the Biosphere (eds., P.A.C. Raats, D.E. Smiles, and A. Warrick), Geophysical Monograph Series Volume 129. Greco, R., 2002. Preferential flow in macroporous swelling soil with internal catchment: model development and applications. Journal of Hydrology, 269(3-4):150-168. Hopmans, J.W., K.C. Roy, and W.W. Wallender. 1991. Irrigation water management and soil-water hysteresis - a computer modeling study with stochastic soil hydraulic properties. Transactions of the ASAE. 34:449-459. Hopmans, J.W., and K.L. Bristow. 2002. Current capabilities and future needs of root water and nutrient uptake modeling. Adv. Agronomy 77:104-184. Hopmans, Jan W., K.L. Bristow, and J. ?imünek. 2002. Indirect estimation of soil thermal properties and water flux from heat pulse measurements: Geometry and dispersion effects. Water Resour. Res. 38(1) 7.1-7.14. Hopmans, J.W. and G.H. Schoups. 2004. Soil water flow at different spatial scales. Encyclopedia of Hydrologic Sciences. Indelman, P., and G. Dagan. 1993b. Upscaling of conductivity of heterogeneous formations: General approach and application to isotropic media. Transp. Porous Media. 12:161-183. James, L. D., Multiple frontiers in hydrology unite for greater good. 2000. EOS, Trans. AGU. 81:310,316. Jin, Y. , Chu, Y. and Li, Y. 2000. Virus removal and transport in saturated and unsaturated sand columns. J. Contam. Hydrol., 43:111-128. Jones O.R. and Popham T.W. 1997. Cropping and tillage systems for dryland grain production in the southern High Plains. Agron. J. 89:222-232. Jones, S.B. and Or, D., 1999. Microgravity effects on water flow and distribution in unsaturated porous media: Analysis of flight experiments. Water Resour. Res., 35(4): 929-942. Jones, S.B., D. Or, and G. Bingham. 2003. Gas diffusion measurement and modeling in coarse-textured porous media. Vadose Zone J. 2:602-610. Kampf, S., M. Salazar and S. Tyler. 2002. Preliminary Investigation of Effluent Drainage from Mine Heap Leach Facilities. Vadose Zone Journal 1:86-196. Kolpin, D.W., E.T. Furlong, M.T. Meyer, E.M. Thurman, S.D. Zaugg, L.B. Barber, and H.T. Buxton. 2002. Pharmaceuticals, hormones, and other organic wastewater contaminants in U.S. streams, 1999-2000: A national reconnaissance. Environ. Sci. Technol. 36, 1202-1211. Lascono, R.W. and C.H.M. van Bavel. 1986. Simulation and measurement of evaporation from a bare soil. Soil Sci. Soc. Am. J. 50:1127-1132. Levy, G.J., I. Shainberg, and W.P. Miller. 1998. Physical properties of sodic soils. In: Sumner (ed.) Sodic soils. Distribution, properties, management, and environmental consequences. Oxford University Press, Inc. New York. 207 p. Masad, E., Muhunthan, B., and B. Martyz, 2000. Simulation of fluid flow and permeability of cohesionless soils. Water Resources Research, 36(4), pp. 851-864. Meixner, T. and M. E. Fenn. 2003. Biogeochemical budgets in a Mediterranean catchment with high rates of atmospheric N deposition - Importance of scale and asynchrony. Biogeochemistry. (in review). Mitchell, J.K., 1993. Fundamentals of Soil Behavior ? 2nd Edition. Wiley, John & Sons, New York. Moroke, T.S., R.C. Schwartz, K.W. Brown, A.S.R. Juo. 2004. Soil water depletion and root distribution of three dryland crops. Soil Sci. Soc. Am. J. (In review). Mori, Y., J.W. Hopmans, A.P. Mortensen, and G.J. Kluitenberg. 2003. Multi-Functional heat pulse probe for the simultaneous measurement of soil water content, solute concentration, and heat transport parameters. Vadose Zone J. 2:561-571. Neuman, S.P. 1990. Universal scaling of hydraulic conductivities and dispersivities in geological media. Water Resour. Res. 26:1749-1758. Nieber, J.L., 1996. Modeling finger development and persistence in initially dry porous media. Geoderma 70, pp. 209-229. Or, D., and M. Tuller, 2003. Hydraulic conductivity of unsaturated fractured porous media: Flow in a cross-section. Advances in Water Recources, Vol.26, No.8, 883-898. Or, D. and Tuller, M.,1999. Liquid retention and interfacial area in variably saturated porous media: Upscaling from single-pore to sample-scale model. Water Resour. Res., 35:3591-3606. Or, D., F.J. Leij, V. Snyder, T.R. Ghezzehei. 2000. Stochastic model for posttillage soil pore space evolution. Water Resour. Res 36:1641-1652. Or, D. and Tuller, M., 2000. Flow in unsaturated fractured porous media: hydraulic conductivity of rough surfaces. Water Resour. Res., 36:1165-1177. Or, D. 2003. Diffusional constraints affecting microbial habitats and activity in unsaturated soils. ASA Abstracts, ASA, Madison, WI. Parlange, M.B., A.T. Cahill, D.R. Nielsen, J.W. Hopmans, and O. Wendroth. 1998. Review of heat and water movement in field soils. Soil Till. Res. 47:5-10. Parton, W. J., D. S. Schimel, C. V. Cole, and D. S. Ojima. 1987. Analysis of factors controlling soil organic matter levels in the Great Plains grasslands, Soil Sci. Soc. Am. J., 51:1173-1179. Parton, W. J., J. W. B. Stewart, and C. V. Cole. 1988. Dynamics of C, N, P, and S in grassland soils: A model. Biogeochemistry, 5:109-131. Perfect, E., V. Rasiah, B. D. Kay. 1992. Fractal dimensions of soil aggregate-size distributions calculated by number and mass. Soil Sci. Soc. Am. J. 56:1407-1409. Philip, J.R., and D.A. de Vries. 1957. Moisture movement in porous materials under temperature gradients. Trans. Am. Geophys. Union 38:222-232. Qureshi, S., J.M. Wraith, G.E. Graves, R.E. Macur, and D. Or. 2003. Water status and nutrient constraints on microbial colonization of unsaturated smooth and rough porous surfaces. ASA abstracts, ASA, Madison, WI. Quirk, J.P., 1986. Soil permeability in relation to sodicity and salinity. Phil. Trans. R. Soc. Lond. A, 316:297-317. Reynolds, W. D., and D. E. Elrick, 1991, Determination of hydraulic conductivity using a tension infiltrometer, Soil Sci. Am. J. 55:633-639. Russo, D. 1992. Upscaling of hydraulic conductivity in partially saturated heterogeneous porous formation. Water Resour. Res. 28:397-409 Schwartz, R.C., S.R. Evett, and P.W. Unger. 2003. Soil hydraulic properties of cropland compared with reestablished and native grassland. Geoderma 116:47-60. Selker J.S, T.S. Steenhuis and J.Y Parlange, 1992. Wetting front instability in homogeneous sandy soils under continuous infiltration. Soil Sci. Soc. Am. J. 56:1346-1350. Simünek, J., M. Sejna, and M.Th. van Genuchten. 1998. The Hydrus-1D software package for simulating the one-dimensional movement of water, heat, and multiple solutes in variably-saturated media, version 2.0. U.S. Salinity Laboratory, USDA-ARS, Riverside, California. Simünek, J., Wendroth O. and van Genuchten M. Th. 1999. Soil hydraulic properties from laboratory evaporation experiments by parameter estimation. In M. Th. van Genuchten, F.J. Leij, and L. Wu (Eds.) Characterization and Measurement of the Hydraulic Properties of Unsaturated Porous Media, Part I. U.S. Salinity Laboratory, USDA-ARS. Simünek, J., M. Sejna, and M. Th. van Genuchten, The HYDRUS-1D software package for simulating the one-dimensional movement of water, heat, and multiple solutes in variably-saturated media. Version 2.0, IGWMC - TPS - 70, International Ground Water Modeling Center, Colorado School of Mines, Golden, Colorado, 202pp., 1998 Simünek, J., and van Genuchten, M. T., Jacques, D., Hopmans, J. W., Inoue, M. and Flury, M. 2002. Solute transport during variably saturated flow-inverse methods. In: Methods of Soil Analysis, Part 4, Physical Methods, Eds. J. H. Dane and G. C. Topp. Soil Science Society of America, Madison, WI, p. 1435-1449. Six, J., C. Feller, K. Denef, S.M. Ogle, M.J.C. Sa, and A. Albrecht. 2002. Soil organic matter, biota and aggregation in temperate and tropical soils - Effects of no-tillage. Agronomie: Agriculture & Environment 22:755-775. Smith, K.A., T. Ball, F. Conen, K.E. Dobbie,J. Masheder, and A. Rey. 2003. Exchange of greenhouse gases between soil and atmosphere : interactions of soil physical factors and biological processes. European J. of Soil Science, 54 :1-13. Spomer, L. A. 1974. Optimizing container soil amendment: The "threshold proportion" and prediction of porosity. HortScience 9:532-533. Tashman, L., Masad, E., Crowe, C., and B. Muhunthan, 2003. Simulation of fluid flow in granular material microstructure using a non-staggered grid scheme, International Journal of Computers and Fluids, 32(9):1299-1323. Tessier, D., 1990. Behavior and microstructure of clay minerals, In: Soil colloids and their associations in aggregates, De Boodt, M.F., M.H.B. Hayes, and A. Herbillon (Editors), Plenum Tessier, D., 1990. Behavior and microstructure of clay minerals, In: Soil colloids and their associations in aggregates, De Boodt, M.F., M.H.B. Hayes, and A. Herbillon (Editors), Plenum Press, New York. Treves, D.S., B. Xia, J. Zhou, and J.M. Tiedje. 2003. A two-species test of the hypothesis that spatial isolation influences microbial diversity in soils. Microbial Ecol. 45:20-28. Tuller, M., Or, D. and Dudley, L. M., 1999. Adsorption and capillary condensation in porous media: liquid retention and interfacial configurations in angular pores. Water Resour. Res., 35:1949-1964. Tuller, M., and D. Or, 2003. Hydraulic functions for swelling soils: pore scale considerations. Journal of Hydrology, 272:50-71. Tuller, M., and D. Or, 2001. Hydraulic conductivity of variably saturated porous media - Film and corner flow in angular pore space. Water Resour. Res., 37(5):1257-1276. Tuller, M., D. Or, and L.M. Dudley, 1999. Adsorption and capillary condensation in porous media -liquid retention and interfacial configurations in angular pores. Water Resour. Res., 35(7):1949-1964. Tyler, S. W., S. W. Wheatcraft. 1990. Fractal processes in soil water retention. Water Resour. Res. 26:1047-1054. Vitousek, P. M. and C. B. Field, Ecosystem constraints to symbiotic nitrogen fixers: a simple model and its implications. 1999. Biogeochemistry, 46(1):179-202. Vitousek, P. M. and C. B. Field, Input/output Balances and Nitrogen Limitation in Terrestrial Ecosystems, in Global Biogeochemical Cycles in the Climate System. 2001. Edited by E. D. Schulze, M. Heimann, S. Harrison, E. Holland, J. Lloyd, I. C. Prentice, and D. Schimel, pp. 217-225, Academic Press, New York. Vrugt, J.A., H.V Gupta, W. Bouten, and S. Sorooshian, A Shuffled Complex Evolution Metropolis algorithm for optimization and uncertainty assessment of hydrological model parameters, Water. Resour. Res., 39, 8, 1201, DOI: 10.1029/2002WR001642, 2003. Vrugt, J.A., G.H. Schoups, Jan W. Hopmans, C. Young, W.W. Wallender, T.H. Harter and W. Bouten. 2004. Identification of spatially-distributed vadose zone properties in hydrologic modeling using global optimization. Water Resour. Res. (Submitted) Wan, J.M. and Wilson, J.L., 1994. Visualization of the role of the gas-water interface on the fate and transport of colloids in porous media. Water Resour. Res., 30:11-23. Wan, J. and Tokunaga, T. K. , 1998. Measuring partition coefficients of colloids at air-water interfaces. Environ. Sci. Technol., 32:3293-3298. Wang, Z, A. Tuli, and W.A. Jury, 2003a. Unstable flow during redistribution in homogeneous soil, Vadose Zone Journal, 2: 52-60. Wang, Z., J. Feyen and C.J. Ritsema, 1998a. Susceptibility and predictability of conditions for preferential flow. Water Resour. Res., 34:2169-2182. Wang, Z., J. Feyen and D.E. Elrick, 1998b. Prediction of fingering in porous media. Water Resour. Res., 34:2183-2190. Wang, Z., L. Wu, T. Harter, J. Lu and W.A. Jury, 2003b. A field study of unstable preferential flow during soil water redistribution. Water Resour. Res. Vol. 39 (4): 1075, doi:10.1029/2001WR000903. Wang, Z., W.A. Jury, A. Tuli, and D.J. Kim, 2003c. Unstable flow during redistribution: Controlling factors and practical implications. Vadose Zone Journal. (in press) Warrick, A. W., 1992, Models for disc infiltrometers. Water Resour. Res. 28:1319-1327. Weisbrod, N., M.R. Niemet, and J.S. Selker, 2002. Imbibition of saline solutions into dry and prewetted porous media, Advances in Water Resources 25: 841?855 Wendroth O., Ehlers W., Hopmans J.W., Kage H., Halbertsma J. and Wösten J.H.M. 1993. Reevaluation of the evaporation method for determining hydraulic functions in unsaturated soils. Soil Sci. Soc. Am. J. 57:1436-1443. West, T.O., and W.M. Post. 2002. Soil organic carbon sequestration rates by tillage and crop rotation: a global data base analysis. Soil Sci. Soc. Am. J. 66:1930-1946. White, M.D., and M. Oostrom. 2004. User's guide of STOMP ? Subsurface transport over multiple phases, Version 3.1. PNNL-14478, Pacific Northwest National Laboratory, Richland, Washington. Wraith, J.M., J. Martin, S. Qureshi, R.E. Macur, and D. Or. 2003. Pore size and wetness impacts on microbial community selection. ASA abstracts, ASA, Madison, WI. Wraith, Jon M., and Dani Or. 2002. How reproducible are soil water retention measurements? p. 219. ASA abstracts, ASA, Madison, WI. Wright, B. D., W. C. Bausch, and W. M. Knott. 1988. A hydroponic system for microgravity plant experiments. Trans. ASAE 31:440-446. Yates, S.R. and Enfield, C.G. 1988. Decay of dissolved substances by second-order reaction. Problem description and batch?reactor solutions. Journal Environmental Science and Health, Part A. Environmental Science and Engineering A23:59?84. Yates. S.R. and Enfield, C.G. 1989. Transport of dissolved substances with second-order reaction. Water Resources Research 25:1757-1762. Zhang, R., A. W. Warrick and D. E. Myers. 1990. Variance as a function of sample support size. Math. Geology. 22:107-121. Zhang, R., A. W. Warrick and D. E. Myers. 1994. Heterogeneity, plot shape effect and optimum plot size, Geoderma, 62:183-197. Zhang, R., 1997. Determination of soil sorptivity and hydraulic conductivity from the disc infiltrometer. Soil Sci. Soc. Am. J. 61:1024-1030. Zheng, W., Papiernik, S.K., Guo, M. and Yates, S.R. 2003. Accelerated Degradation of Methyl Iodide by Agrochemicals. Journal of Agricultural and Food Chemistry. 51:673-679. Zheng, W., Papiernik, S.K., Guo, M. and Yates, S.R. 2004. Remediation of methyl iodide in aqueous solution and amended soils with thiourea. Environmental Science and Technology. (In press)