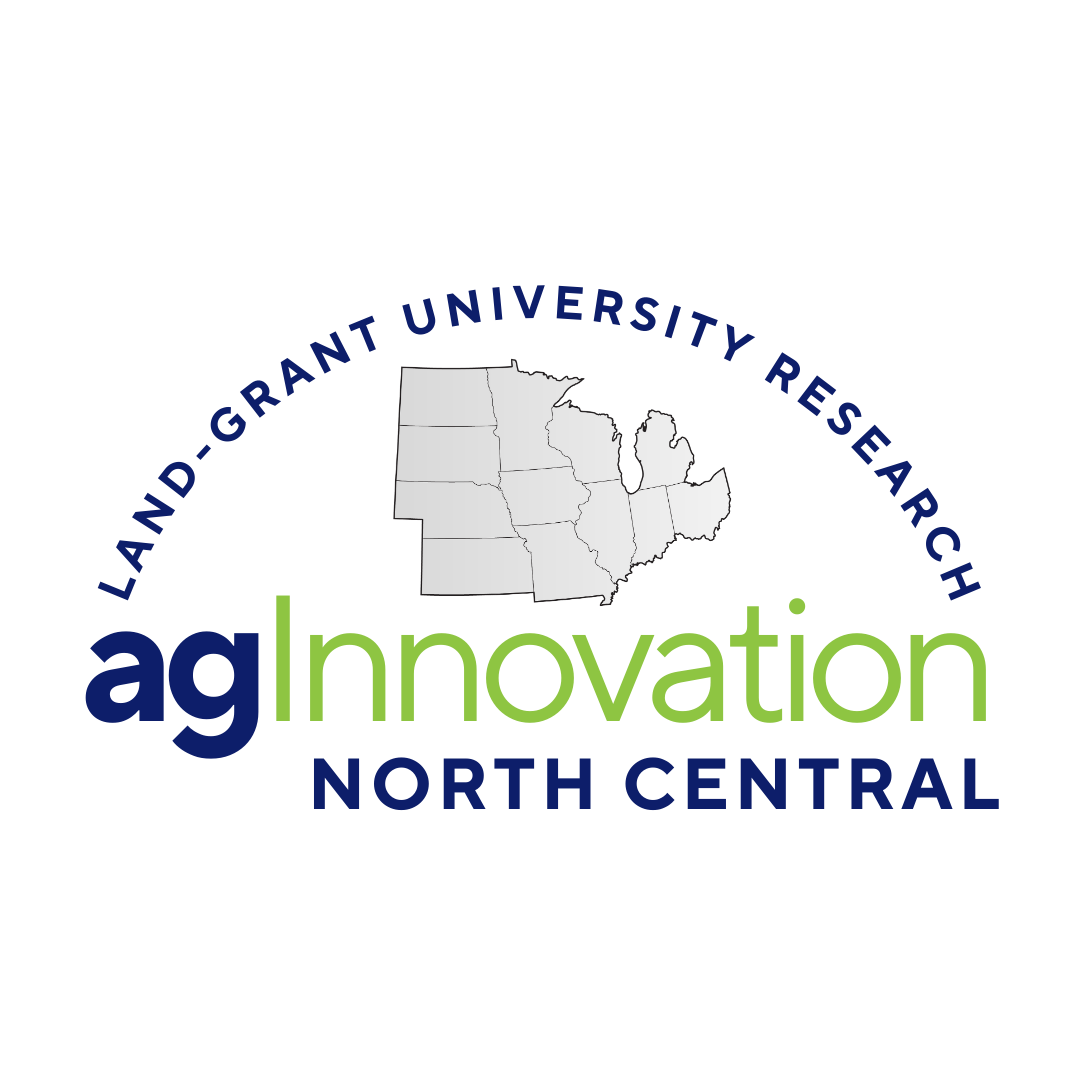
NC174: Management of Eroded Soils for Enhancement of Productivity and Environmental Quality
(Multistate Research Project)
Status: Inactive/Terminating
NC174: Management of Eroded Soils for Enhancement of Productivity and Environmental Quality
Duration: 10/01/2001 to 09/30/2003
Administrative Advisor(s):
NIFA Reps:
Non-Technical Summary
Statement of Issues and Justification
Soil erosion results in a tremendous economic loss to society. Crosson (1984) estimated a monetary loss in the USA from soil erosion effects on cropland productivity to be 40 million dollars in any one year. For many soils, continued erosion results in degraded topsoil and declines in yield which are cumulative over time as root restrictive layers, such as fragipans, high clay content, or coarse sand, move closer to the soil surface (Langdale et al., 1985). Offsite damages to the environment from soil erosion are also considerable. Environmental costs due to soil erosion in the USA are estimated at 3.1 billion dollars each year (Pimentel, 1992). JUSTIFICATION: This research project was initially designed to strengthen the scientific foundation for describing the potential crop production of selected soils, for predicting changes in the potential crop production of these soils as erosion occurs, and for evaluating the potential to extrapolate this information to other soils not studied. A coordinated regional effort provided the diverse range in soil and climatic characteristics necessary to develop the basic principles relating erosion to soil and crop productivity. In the first 5-year phase of the NC-174 project (1983-1988) we identified and documented the effects of erosion on soil properties and corn or small grain yield. Five years of data were collected to better document the effects of weather on the interaction between soil properties and corn yield in the north central United States. Once the database was enlarged emphasis was placed on selection of management and restoration alternatives at either the initial or a new site. The NTRM (Nitrogen, Tillage, Residue and Management) and EPIC (Erosion-Productivity Impact Calculator) models were used in conjunction with the existing data base collected during the initial phase of the NC-174 project to identify factors limiting crop productivity of each soil series. The models were used to evaluate long-term effect of management and restoration alternatives prior to field testing. The second phase of the project (1988-1993) was to field test the practices selected to maintain or enhance current productivity and to determine the extent to which productivity of eroded soils can be restored. The third phase of the project (1993-1998) determined threshold soil property values for the restoration of productivity and quality of eroded soils. Understanding soil erosion in relationship to crop productivity, environmental, and economic impacts is of particular importance since the passage of The Food Security Act of 1985 (P.L. 99- 198) (U.S. Congress, 1985) and the 1990 and 1996 Farm Bills. These acts significantly change the adoption of soil and water conservation practices because they required strict conservation measures be used on land classified as highly credible if the farm operator chose to continue eligibility for farm price and income support programs. Getting needed information on land management requires substantial regional effort. The North Central Regional Association of Agricultural Experiment Stations developed a May 1995 list of research priorities for seven Cross-cutting Research Areas and Objectives. The proposed Regional Project address the top three objectives for the Natural Resources and the Environment. These include: (1) Identify and apply ecosystem management principles and practices or the utilization and protection of resources, restoration of natural systems and management of rural landscapes. (2) Define sustainable principles for resource management, utilization and land use. (3) assess the relationships of agricultural/forestry practices (primary production) upon soil and water systems and bio-diversity.
Related, Current and Previous Work
The following regional research projects have similar focus and/or directions as those of the proposed project.
NC-218 | Characterizing Nitrogen Mineralization and Availability in Crop Systems to Protect Water Resources | 9/00 |
NCR-59 | Soil Organic Matter and Soil Quality | 9/00 |
NCR-180 | Site Specific Management | 9/01 |
S-207 | Mineralogy and Charged Properties of Readily Dispersed Fraction from Selected Soils and Sediments | 9/01 |
S-257 | Classify for Solute Transport as Affected by Soil Properties and Landscape Position | 9/98 |
SR-1EG-69 | Inventory Classification and Waste Application Potential of Disturbed Lands | 12/00 |
SERA-1EG-17 | Minimizing Agriculture Nutrients Runoff Losses for Protect of Water Resources | 9/98 |
SERA-IEG-20 | Southern Tillage Conference | 9/99 |
SR-DC-98-03 | Develop and Assessment of Precision Farming Techniques for the South | 9/98 |
WCC-021 | Re-vegetation and Stabilization of Deteriorated and Altered Lands | 9/99 |
W-190 | Water Conservation, Competition and Quality in Western Irrigated Agriculture | 9/99 |
NEC-100 | Application of Sewage to Soils in Northeast; Long-term Impacts and Beneficial Uses | 9/99 |
The two existing Regional Projects which appear to relate to our proposal are NC-218 (Characterizing Nitrogen Mineralization and Availability in Crop Systems to Protect Water Resources) and NCR-59 (Soil Organic Matter and Soil Quality).
The objectives of the NC-218 include: (1) determination of the feasibility of using thermal units coupled with an appropriate measure of potentially mineralizable soil N to predict current N availability and (2) characterize the active and N and C pools subject to mineralization in cropping systems. NC-218 focus relates primarily to the feasibility of measuring mineralizable soil N and the impact of cropping systems on mineralization. NC-174 emphasis is on management effects on eroded soil productivity and quality of soil, air and water resources. Total soil C is used as an indicator of the impact of erosional impacts on soil processes and properties.
The objectives of NCR-59 (an approved but non-funded regional project) include: (1) development and application of methodology for characterizing the biotic component of soil quality and its interaction with nonliving and living soil organic matter and (2) defining the relationship of the biotic to the descriptive, and physical and chemical components of soil quality. Most of NCR-59 work is on the biotic component of soil quality and its relationship to soil organic matter as well as physical and chemical components. There is little overlap with NC-174 which has focused more on the chemical and physical properties of soil with the biological component limited primarily to total C or total N. Proposed NC-174 emphasis relates to the effects of management on eroded soil productivity and quality of soil, air and water resources. Total soil C is used as only one indicator of the impact of erosional impacts on soil processes and properties. NC-174 and NCR-59 committees have developed a close working relationship which has resulted in two joint meeting's to share information and contribute to joint efforts such as a Methods book. Aside from this, the two projects complement each other since the work will be conducted in the same region of the U.S. with similar soils and weather patterns.
Based on a CRIS survey of over 28,000 other CSRS, ARS, and state p rejects, only 17 were found that relate to restoration of eroded land and soil productivity. Some that relate directly or indirectly to the objectives of this project are:
MANAGEMENT OF ERODED LAND FOR ENHANCEMENT OF PRODUCTIVITY AND ENVIRONMENTAL QUALITY
Agency | Investigator | Title |
CSRS MO | Thompson | Erosion, crop productivity, and water management |
CSRS MO | Gantzer | Soil and water conservation: maintaining a quality nvironment with sustainable environment |
CSRS MO | Hammer | Temporal and spatial variability in soil landscapes |
CSRS MO | Ward | Effect of selected agricultural practices on profitability, water quality and soil properties |
ARS6613 | Wilkinson | Optimizing biochemical processes for agroecosystems |
ICAR | Juma | Soil structure development in eroded black chernozems and gray luvisols |
ARS3640 | Allmaras | Soil and water management to optimize production and enhance natural resources |
ARS3645 | Lindstrom | Enhancement of soil properties through soil management |
CSRS NEB | Powers | Soil physical relationships for best management practices to protect water quality |
CSRS SD | Gartner | Water intake, runoff, and vegetation characteristics of soils with various histories of land |
CSRS OK | Stoecker | Economic analysis of soil erosion and water quality constraints on crop production systems |
ARS5348 | Smith | Enhancing soil, water and air quality in the Northwest wheat region |
ARS6209 | Unger | Managing soil and water resources for sustainable agriculture in the Southern Great Plains |
CSRS CO | Peterson | Crop and soil management systems in dryland agroecosystems |
ARS6420 | Erbach | Improving soil productivity and environmental quality with organic wastes |
ARS6645 | Miller | Assessing the condition of agricultural systems in the United States |
CSRS IL | Olson | Crop yield and productivity index ratings for Illinois soils |
CSRS IN | Engel | Information systems for environmental and natural resource management |
The extent of erosion and the phase of eroded soil is identified on the basis of the properties of soil that remains (Soil Survey Staff, 1993). Classes of erosion are defined in Chapter 4 of Soil Survey Manual (Soil Survey Staff, 1993) on the basis of the percentage of original A horizon lost or the uppermost 20 cm if the original horizon was less than 20 cm thick.
Researchers compared the uncultivated and undisturbed sites with eroded sites to determine the intensity of erosion. These techniques include the comparison of soil properties (Olson et al., 1994) and geomorphological effects (Kreznor et al., 1989; 1992). In Southern Illinois, Olson et al. (1994) found the removal of a layer of 7.5 cm from a cultivated site, with slopes in the range of 5-10 percent, during 80 years because of accelerated erosion on a backslope. They arrived at these results by comparing the soil properties of the cultivated site with a nearby forested site with similar soil, slope, and landscape characteristics. Kreznor et al. (1989) compared the accelerated erosion effect on cultivated site with an uncultivated and uneroded cemetery site in Northwestern Illinois having similar slopes in the range of 6-10 percent. They found a decrease in A horizon thicknesses and organic C contents and an increase in clay contents because of cultivation and erosion. Among the landscape positions, the shoulder was slightly eroded, whereas lower backslope and upper footslope were severely eroded due to greater slope length.
Jones and Olson (1990) reported that fly ash constituents of more than 75 percent of the magnetic fraction in upper 12.5 cm soil layer, on a uncultivated stable ridgetop near Springfield, Illinois. At this site, presence of less than 1 percent fly ash in deeper horizon was thought to be the mixing by soil fauna activity. Fly ash was found to a 45 cm depth in Sangamon river floodplain and deeper occurrence of fly ash on the floodplain was attributed to stream deposition. Physical appearance of fly-ash is the spheroids of siliceous glass with the diameter of less than 20 microns. Fly-ash particles are able to travel long distances in airstream because of the low settling velocities of particles ranging from 1 to 20 microns with respect to normal wind speed (Wark and Wamer, 1976). The deposition of inorganic spherules of fly ash was observed to a distance of 45 km from the coal-powered plant in Glasgow, Scotland (Rose, 1996).
Soil erosion and sediment deposition are perceived by the scientific and nonscientific communities as serious threats to our economy and environment. An estimated 3-4 billion tons of soil are removed from our farmland annually (Schwab et al., 1966; Beasley, 1970; Pimentel et al., 1976). Most of this soil is lost from the fertile, organic-enriched surface horizon.
Pimentel et al. (1976) estimates that one-third of the topsoil has been lost from the U.S. cropland in the past 200 years. Despite such soil loss, food production has increased substantially over the past 200 years, because of technological advances (including additional fertilizer). Consequently, we do not know to what extent present and future yields are or will be limited by land abuse. These relationships have been investigated by researchers using a number of approaches including artificial removal of the soil surface (scalping), studying cut and fill areas, and naturally-eroded sites.
Earlier studies where exposed subsoil by land leveling was equated to exposed subsoil due to water erosion found that high crop yield may be retained by increasing fertilizer input on cut areas of leveled fields (Whitney et al., 1950; Heilman and Thomas, 1961; Reuss and Campbell, 1961; Phillips and Kamprath, 1973).
In Kentucky (Frye et al., 1982) corn grain yields for eroded soils decreased 12-21% when compared to uneroded soils. Spomer and Piest (1982) compared yields from 16 years for summit, backslope, and footslope positions; they found that footslope positions produced higher yields. In North Carolina researchers (Stone et al., 1985) showed landscape position to be a better parameter than erosion phase as a predicator of corn yield due to associated moisture condition reflecting the net effects of surface and subsurface water movement.
In the Midwest and Western U.S., precipitation and available soil water limit crop production (Eck, 1969) when nutrients are in adequate supply. The extent of production loss on eroded soils depends largely on landscape position, runoff and internal drainage. Nebraska research has indicated that lower slope positions had more available water than those at higher positions on the same slope (Hanna et al., 1982; 1983). For droughty areas which are highly eroded, residue maintenance on the soil surface can reduce runoff and increase infiltration and hydraulic conductivity (Pikul and Allmaras, 1986).
The casual relationship for loss of production is continuing to be evaluated. Variation in crops, soil types, climate and landscape position each contribute to crop response. Restoration of eroded soil should be such that their production potential is equivalent to a similar noneroded soil. The general principal of restoration is to replace one or more properties of topsoil that was lost from the field when erosion occurred.
Many efforts have been made to restore eroded soils to higher production levels by adding fertilizer. This approach assumes that fertilizer, especially nitrogen, is the major limiting factor. Fertilizer has been responsible for improving yields in Wisconsin on silt loam soils (Hayes et al., 1948). Where precipitation (Englestad and Schrader, 1961) and restrictive soil layers such as fragipans (Shrader and Langsdale, 1979) limit production, fertilizers have not been successful in increasing yields of eroded soils to levels similar to the noneroded areas. Other chemical amendments such as lime and gypsum have also been beneficial to crop production on acid soils in the Southeast (Hammel et al., 1985).
Another approach to the restoration process is to replace topsoil onto eroded soils. Although this is a relatively cumbersome and expensive procedure, topsoil can provide benefits to plant growth which cannot be readily replaced such as increased rooting depth and available water- holding capacity. Wheat yields on eroded soils receiving 150 mm of topsoil out yielded the eroded soil receiving 68 kg/ha N (Massee and Waggoner, 1985). An addition of 100 mm and 200 mm were required to maximize corn and oat production on an exposed C horizon (Mieike and Schepers, 1986), respectively.
Water is one of the major yield-limiting factors on eroded soils. Only by irrigation can we replace, on demand, the loss of available water. However, other water management techniques may be employed as ameliorating processes for eroded soil. In addition to irrigation, water management by surface mulching has been shown to be an alternative means of sustaining yields on eroded soils (Batchelder and Jones, 1972).
See attachment for additional Previous Work
Objectives
-
Determine erosional and landscape impacts on soil processes
-
Assess management effects on eroded soil productivity and quality of soil, air and water resources
Methods
Objective 1. Determine erosional and landscape impacts on soil processes and properties.
The USDA, Soil Conservation Service has established "T" values (soil loss tolerance values) for all soils in the north central U.S. to indicate the maximum rate of annual soil erosion that will permit a sustainable level of crop productivity (McCormack et al., 1982). With our existing data base which includes numerous soils with root-restricting subsoils, we believe simulation models can help to evaluate the long-term productive capacity of the soil and determine if the T value for a soil is too high to permit a sustainable level of crop productivity. Illinois, Iowa, North Dakota and Ohio will address the need to better determine the rate of topsoil formation (increase in organic carbon content) in order to assess the time required to restore lost soil productivity. Rates of topsoil formation will be documented by measuring the organic carbon content, soil color, and topsoil thickness differences between cropping systems, amendments, and soil and water management treatments. Additional changes between check plots in soils formed under prairie or forest vegetation and treatments can be used to document the rate of topsoil formation. A regional approach rather than a state approach is required since weathering rates of parent materials and rates of topsoil formation appear to vary with climate (rainfall and temperature), parent material, crop, landscape position, depth to the unaltered parent material, and existing topsoil thickness.
The severity of accelerated erosion is dependant upon slope gradient, shape of slope, slope length, and tillage practices. 137 and fly ash methods will be used to determine erosional and landscape impacts on soil processes and properties. Fly ash, the product of high temperature coal combustion, was used a sedimentation marker in some studies. The objective of this study will be to determine the extent of soil removal from a hillslope due to accelerated erosion and its subsequent deposition on a lower landscape position using the presence, depth and concentration of fly ash and magnetic susceptibility to identify the transported soil. The study will be done by comparing the amount of fly ash deposited in the soil profile as different landscape positions of the cultivated site with an uncultivated woodland site (Illinois. Iowa and North Dakota).
Objective 2. Assess management effects on eroded soil productivity and quality of soil. air and water resources.
Soil and crop management methods to be investigated for the maintenance and/or restoration of soil productivity on eroded soils will be based on evaluation of the literature, assessment of current research, simulation modeling using the existing data base (1st phase of project) to identify limiting factors of a specific soil, and field testing of promising management alternatives (All participating states). Long term data are necessary to adequately evaluate the management-climate interactions on yields. Prior to the initiation of this project, most yield and management systems research were conducted on uneroded soils and soils not subject to significant erosion. Various cropping systems, amendments, and soil management systems were used by each state. The contributions from each state allow a comparison of yields obtained from the first phase of the project to be used in an evaluation of the cropping systems, amendments, and soil and water management among a range of soil and climatic conditions in the region.
Lindstrom et al. 1992; Schumacher et al. 1994; and Shaffer et al. 1994, have demonstrated the sensitivity of crop productivity in many of the soils studied in the North Central Region to available water holding capacity as well as growing season precipitation. The model output can be evaluated statistically if the inputs are replicated. All participating states have received outputs from the model to be used as a tool in the interpretation of mechanisms acting on soil productivity. Sensitivity analysis was conducted on selected soil properties to determine if erosion induced variation accounts for observed productivity effects. The model simulation outputs have been combined with conventional data analysis and interpretation. Results have been used in the selection of an appropriate restoration or management strategy for field testing. Management factors have been changed in the simulation models to evaluate the optimum potential for the maintenance and restoration of soil productivity on eroded soils and to quantify the management factors contributing to production. Methods included: (1) cropping systems (Illinois, Iowa, Ohio, South Dakota, Missouri, and North Dakota) (2) amendments (Wisconsin, North Dakota, Minnesota and Michigan) and (3) tillage and water management (Illinois, South Dakota, Ohio, Missouri, and Minnesota). State assignments (by voting members of NC-174 committee) were based on the specific factors limiting crop productivity on their site (have been determined using models and findings from the 1983 to 1988 study). The more promising management alternatives for a soil, based on previous research and simulation modeling, were or are being field tested to determine the yield potential, time required for productivity maintenance and/or restoration, and economic efficiency (All participating states). (1) The existing eroded plots (3 replications on moderately eroded and 3 replications on severely eroded) are being compared to the 3 untreated slightly eroded plots in an effort to reduce the yield differences. (2) The promising treatment are being applied to all plots (slightly, moderately, and severely eroded) to determine if the yield differences observed during the initial 5 years can be reduced or eliminated. (3) An alternative required the location of 6 plots in each of the slightly, moderately, and severely eroded phases. Then subplots in each phase could remain under the initial treatment (check) and would receive the new treatment. Existing site constraints, management application difficulties, and landowner restrictions limited the approach being used by individual states.
The threshold soil values and productivity parameters serve as an early warning signal of reduced productive capacity of soils common to the north central United States. Specific soil parameters and threshold values will be suggested for both surface and subsoil layers. AH participating states will use data previously collected as part of the NC-174 project to evaluate the soil parameters and to determine threshold values for degraded productive capacity. Possible changes in the surface layer properties that appear to affect productivity under a high level of management include: erosion phase, porosity, bulk density, aggregation, organic C, infiltration, texture, and coarse fragments. Changes in the subsoil properties that affect soil productivity include mechanical strength, structure, aeration porosity, water storage, porosity, residual porosity, bulk density, hydraulic conductivity, pH, and rooting depth.
Minor and major reductions in inherent soil productivity will be considered as a basis for setting threshold values for measurable and observable soil properties or conditions based on current methods of technology and research. Threshold values will be determined by correlating soil properties with yields obtained from research fields as well as from simulation models. The threshold values will serve as an indicator of reduced productivity capacity. These soil parameters and specific threshold values will be based on erosion-productivity relationships shown by previous NC-174 research as affected by soil conditions in the north central United States which can vary with location, crop, soil, management, and climate (all participating states). Our previous analyses have identified fourteen soil properties and cultural practices as being limiting to crop production in addition to precipitation. The specific properties limiting production were soil and/or location dependent. These facts demonstrate the advantage of a regional approach in which there is a wide range in soil and climatic variables. Olson (1992) defined soil quality standards for soil physical properties as related to productivity. He suggested threshold values for physical properties of the surface and subsoil layers which affect soil productivity. Values for soil properties were defined as no reduction (less than 1%), minor (1 to 15%) reduction, major (more than 15%) reduction, in productivity as reflected in corn yields. Similar procedures for chemical and biological parameters can be developed. These values could serve as warning signals of reduced productivity. Sensitivity analyses will be conducted on selected soil properties to determine if erosion induced variation accounts for observed productivity effects. The model simulation outputs will be combined with conventional data analyses and interpretations. The results from the sensitivity analyses will be compared with physically determined threshold values. Based on a region-wide comparison of these analyses, threshold values and properties will be determined for selected soils not included in the original study. A number of states are working on joint research efforts to solve problems and it is anticipated that multi-state publications will result.
Due to the complex response of soil properties and crop yield to different tillage systems and amendments, a soil quality index approach (Harris et al. 1996) will be evaluated. The hypothesis will be tested by quantifying soil properties into a soil quality index with the objective of the evaluation of different tillage systems and amendments effect on soil quality within a state or region (Illinois). In the process of soil quality assessment, the selection of proper soil quality indicators, standardized scoring function, and threshold limits for soil quality indicators are important for the sensitivity of the model to the management practices and local conditions could change the soil quality index. Additional objectives will be to test the Harris et al (1996) soil quality index and modify it according to the state and regional conditions and to test the validity of different soil quality indexes by using correlation between dependant variables (erosion, yield and plant population) and soil quality function ratings.
Measurements will be made on existing eroded and non-eroded sites corresponding to' inputs for a soil quality index designed to include soil properties that are critical for sustaining productivity while maintaining or improving off-site environmental quality (Doran and Jones, 1996).
Restorative effects of BMPs on enhancement in soil quality and C sequestration will be measured through periodic measurement of SOC contents of soil (Nelson and Sommers, 1982). Gaseous emissions of CO; can also be measured using the static chamber technique (Ohio and Minnesota) (Rolston, 1986).
Measurement of Progress and Results
Outputs
Outcomes or Projected Impacts
- This project will contribute mainly with scientific publications and guidebooks. These publications will address the specific benefits of various innovative and beneficial management practices for enhanced productivity and environmental quality. The knowledge communicated through these publications will benefit producers and the general public by maintaining and improving environmental quality. The knowledge will allow for better management decisions to insure environmental quality into the future.
Milestones
(0):0Projected Participation
View Appendix E: ParticipationOutreach Plan
Organization/Governance
The Regional Technical Committee will follow the operational procedures listed on pages 19-22 and 34-36 of the CSRS "Manual for Cooperative Regional Research" revised and dated October 1992. The voting membership of the Regional Technical Committee includes one representative from each cooperating agricultural experiment station or institution appointed by the director and a representative of each cooperating USDA-ARS research unit or location. The administrative advisor and the CSRS representative are non-voting members. All voting members of the Technical Committee are eligible for office. The offices of the Regional Technical Committee includes the Chair, the Vice-Chair and the Secretary. These officers will be elected annually at the Technical Committee meeting, and they may succeed themselves. The duties of the Technical Committee are to coordinate work activities related to the project. The Chair, in accord with the Administrative Advisor, will notify the Technical Committee of the time and place of the meeting, will prepare the agenda and preside at meetings of the Technical Committee and Executive Committee. He or she is responsible for preparing the annual progress report and coordinating the preparation of regional reports. The Vice-Chair assists the Chair in all functions and the Secretary records the minutes and performs other duties assigned by the Technical Committee or Administrative Advisor. The Chair appoints subcommittees as needed. Annual meetings will be held by the Technical Committee, as authorized by the Administrative Advisor, for the purpose of conducting business related to the project.
Literature Cited
Acton, D.F. 1989. The development of a soil quality evaluation system for assessing agricultural sustainability in Canada-a Research Proposal. Land Resource Research Centre. Mimeo. Batchelder, A.R., and J.N. Jones, Jr. 1972. Soil management factors and growth of Zea mays L. on topsoil and exposed subsoil. Agron. J. 64:648-652. Beale, O.W., G.B. Nun, and T.C. Peele. 1955. The effects of mulch tillage on runoff, erosion, soil properties, and crop yields. Soil Sci. Soc. Am. Proc. 19:244-247. Beasley, R. P. 1972. Erosion and sediment pollution control. 6th ed. The Iowa State University Press, Ames, Iowa. Blaikie, P., and H. Brookfield. 1987. Approaches to study of land degradation, p. 27-48. In P. Blaikie and H. Brookfield (ed.) Land Degradation and Society. Methuen and Company, New York. Bouwman, A.F. (Ed). 1990. Soils and the Greenhouse Effect. J. Wiley and Sons, Chichester, U.K. Crosson, P. 1984. New perspectives on soil conservation policy. J. Soil Water Cons. 30:222-225. Daniels, R. B., J. W. Gilliam, D. K. Cassel, and L. A. Nelson. 1985. Soil erosion class and landscape position in the North Carolina Piedmont. Soil Sci. Soc. Am. J. 49:991-995. DeWalle, F.B. 1987. Soil standards for hazardous waste disposal and cleanup in the Netherlands. p. 461-468. In Second International Conference on New Frontiers for Hazardous Waste Management. U. S. Environmental Protection Agency, Hazardous Waste Engineering Research Laboratory, Cincinnati, Ohio. USEPA Rep. 600/9-87/018F. Doran, J.W., and A. J. Jones. Methods for Assessing Soil Quality. Soil Science Society of America. SSSA Special Publication Number 49: 410 p. Doran, J. W., and T. B. Parkin. 1994. Defining and assessing soil quality, Chapter 1, p:3-21. In Doran, J. W., D. C. Coleman, D. F. Bezdieck and B. a. Stewart (eds.). Defining soil quality for a sustainable environment. (1st ed.) Soil Sci. Soc. Am. Pub. No 35. Soil Sci. Soc. Am., Inc, Madison, WI. Eck, H.V. 1969. Restoring productivity on Pullman silty clay loam subsoil under limited moisture. Soil Sci. Soc. Am. Proc. 33:578-581. Eleveld, B., G.V. Johnson, and R.G. Dumsday. 1983. SOILEC - Simulating the economics of soil conservation. J. Soil Water Conserv. 38:387-389. Engelstad, O.P., and W.D. Shrader. 1961. The effect of surface soil thickness on corn yields: II. As determined by an experiment using normal surface soil and artificially exposed subsoil. Soil Sci. Soc. Am. Proc. 25:497-499. Engelstad, O.P., W. D. Shrader, and L.C. Dumenil. 1961. The effect of surface soil thickness on corn yields: I. As determined by a series of field experiments in farmer-operated fields. Soil Sci. Soc. Am. Proc. 25:494-497. Frye, W.W., S.S. Ebelhar, L.W. Murdock, and R.L. Blevens. 1982. Soil erosion effects on properties and productivity of two Kentucky soils. Soil Sci. Soc. Am. J. 46:1051-1055. Frye, W.W., O.L. Bennett, and G.J. Buntley. 1985. Restoration of crop productivity on eroded or degraded soils, p. 335-355. In R.F. Follett and B. a. Stewart, eds. Soil Erosion and Crop for productivity. ASA, 677 South Segoe Road, Madison, WI 53711, USA. Griffith, R.W., C. Goudey, and R. Poff. 1992. Current application of soil quality standards. In Proc. of the Soil Quality Standards Symposium. USDA. Forest Service. WO-WSA-2. Hammel, J.E., M.E. Sumner, and H. Shahandeh. 1985. Effect of physical and chemical profile modification on soybean and corn production. Soil Sci. Soc. Am. J. 49:1508-1511. Hanna, A.Y., P.W. Harlan, and D.T. Lewis. 1982. Soil available water as influenced by landscape position and aspect. Agron. J. 74:999-1004. Hanna, A.Y., P.W. Harlan, and D.T. Lewis. 1983. Effect of slope on water balance under center pivot irrigations. Soil Sci. Soc. Am. J. 47:760-764. Harns. R. F., D. L. Karlen and D. J. Mulla. 1996. a conceptual framework for assessment and management of soil quality and health. Chapter 4, p:61-82. In Doran, J. W., and a. J. Jones, (eds.). Methods for assessing soil quality. (1st ed.) Soil Sci. Soc. Am. Pub. No 49. Soil Sci. Soc. Am., Inc. Madison, WI. Karlen, D. L., M.J. Mausbach, J. W. Doran, R. G. Cline, R. F. Harris, and G. E. Schuman. 1997. Soil quality: a concept definition, and framework for evaluation (a guest editorial). Soil Sci. Soc. Am. J. 61:4-10. Hayes, O.E., C.E. Bay, and H.H. Hull. 1948. Increasing production on an eroded loess derived soil. Agron. J. 40:1061-1069. Heilman, M.D., and J.R. Thomas. 1961. Land leveling can adversely affect soil fertility. J. Soil Water Conserv. 16:71-72. Johnson, M.G., D.A. Lammers, C.P. Andersen, P.T. Rygiewiz, andJ.S. Kem. 1992. Sustaining soil quality by protecting the soil resource. In Proc. of the Soil Quality Standards Symposium. USDA. Forest Service. WO-WSA-2. Jones, R. L., and K. R. Olson. 1990. Fly ash use as a time marker in sedimentation studies. Soil Sci. Soc. Am.J.54: 1393-1401. Karlen, D. L., and D. E. Stott. 1994. a framework for evaluating physical and chemical indicators of soil quality, Chapter 4, p: 53-72. In Doran, J. W., D. C. Coleman, D. F. Bezdieck and B. a. Stewart. (eds.). Defining soil quality for a sustainable environment. (1st ed.) Soil Sci. Soc. Am. Pub. No 35. Soil Sci. Soc. Am., Inc, Madison, WI. Kreznor, W. R., K. R. Olson, W. L. Banwart, and D. L. Johnson. 1989. Soil, landscape, and erosion relationships in a Northwest Illinois. Soil Sci. Soc. Am. J. 53: 1763-1771. Kreznor, W. R., K. R. Olson, and D. L. Johnson. 1992. Field evaluations of methods to estimate soil erosion. Soil Sci. 153:69-81. Lal, R. 1995. Global soil erosion by water and carbon dynamics, p. 131-142. In R. Lal, J.M. Kimble, E. Levine and B.A. Stewart (eds). Soils and Global Change. CRC/Lewis Publishers, Boca Raton, FL Lal, R., J.M. Kimble, E. Levine and B.A. Stewart (eds). 1995a. Soils and Global Change. CRC/Lewis Publishers, Boca Raton, FL 440p. Lal, R., J.M. Kimble, E. Levine and B.A. Stewart (eds). 1995b. Soil Management and Greenhouse Effect. CRC/Lewis Publishers, Boca Raton, FL 385p. Langdale, G.W., J.E. Box. Jr., R.A. Leonard, A.P. Barnett, and W.G. Fleming. 1979. Corn yield reduction on eroded Southern Piedmont soils. J. Soil Water Conserv. 34:226-228. Langdale, G.W., H.P. Denton, A.W. White, Jr., J.W. Gilliam, and W.W. Frye. 1985. Effects of soil erosion on crop productivity of Southern soils, p. 251-269. In R. J. Follett and B.A. Steward, (ed). Soil Erosion and Crop Productivity. Am. Soc. Agron., 677 S. Segoe Road, Madison, WI. Larson, W. E., and F. J. Pierce. 1994. The dynamics of soil quality as a measure of sustainable management. Chapter 3, p:37-52. In. Doran, J. W., D. C. Coleman, D. F. Bezdieck and B. a. Stewart. (eds.). Defining soil quality for a sustainable environment. (1st ed.) Soil Sci. Soc. Am. Pub. No 35. Soil Sci. Soc. Am., Inc., Madison, WI. Larson, W.E. and B.A. Stewart. 1992. Thresholds for soil removal for maintaining cropland productivity. In Proc. of the Soil Quality Standards Symposium. USDA. Forest Service. WO-WSA-2. Lewis, D.T., and M.J. Lepele. 1982. Quantification of soil loss and sediment produced from eroded land. Soil Sci. Soc. Am. J. 46:369-372. Lindstrom, M.J. T.E. Schumacher, A.J. Jones and C. Gantzer. 1992. Productivity index model comparison for selected north central region soils. J. Soil Water Conserv. 47:491-494. Massee, T.W., and H.O. Waggoner. 1985. Productivity losses from soil erosion on dry cropland in the intermountain area. J. Soil Water Conserv. 40:447-450. McCormack, D.E., K.K. Young, and L.W. Kimberlin. 1982. Current criteria for determining soil loss tolerance, p. 41-52. In B. L. Schmidt (ed.). Determinants of Soil Loss Tolerance. Am. Soc. Of Agron., Spec. Pub. 45, Madison, WI, USA. Mieike, L.N., and J.S. Schepers. 1986. Plant response to topsoil thickness on an eroded loess soil. J. Soil Water Conserv. 41:59-63. Moore, I. D., G. J. Burch, and E. M. O'Loughlin. 1986. Comments on "Soil erosion class and landscape position". Soil Sci. Soc. Am. J. 50:1374-1375. Neil, L. L. 1979. An evaluation of soil productivity based on root growth and water depletion. M.S. thesis, University of Missouri, Columbia. Nelson, D.W. and L.E. Somers. 1975. a rapid and accurate procedure for estimation of organic carbon in soils. Proc. Indian Aca. Sci. (1974) 84:456-462. Norton, L.D. 1986. Erosion-sedimentation in closed drainage basin in northwest Indiana. Soil Sci. Soc. Am. J. 50:209-213. Olson, K.R. 1992. Soil physical properties as a measure of cropland productivity. In Proc. of the Soil Quality Standards Symposium. USDA. Forest Service. WO-WSA-2. Olson, K.R., and A.H. Beavers. 1987. A method to estimate soil loss from erosion. Soil Sci. Soc. Am. J. 51:441-445. Olson, K.R., and E. Nizeyimana. 1988. Effects of soil erosion on corn yields of seven Illinois soils. J. Prod. Agric. 1:13-19. Olson, K. R., S. R. Phillips, and B. K. Kitur. 1994. Identification of eroded phases of an alfisol. Soil Science. 157:108-115. Onstad, C. A., F. J. Pierce, R. H. Dowdy, and W. E. Larson. 1985. Erosion and productivity interrelations on a soil landscape. Transactions of ASAE. 28:1885-1888. Phillips, J.A., and E.J. Kamprath. 1973. Soil fertility problems associated with land farming on the Coastal Plain. J. Soil Water Conserv. 18:69-73. Pierce, F.J., W.E. Larson, R.H. Dowdy, and W.A.P. Graham. 1983. Productivity of soils: Assessing long-term changes due to erosion. J. Soil Water Conserv. 38:39-44. Pikul, J.L., Jr., and R.R. Allmaras. 1986. Physical and chemical properties of a Haploxeroll after fifty years of residue management. Soil Sci. Soc. Am. J. 50:214-219. Pimentel, D., E.C., Terhune, R. Dyson-Hydson, S. Rochereau, R. Smais, E.A. Smith, D. Deuman, D. Reifschneider, M. Shepard. 1976. Land degradation: effects on food and energy resources. Science 194:149-155. Rain, D.D. 1983. COSTS - Selecting cost-effective soil conservation practices. J. Soil Water Conserv. 38:384-386. Reuss, J. 0., and R.E. Campbell. 1961. Restoring productivity to leveled land. Soil Sci. Soc. Am. Proc. 25:302-304. Rolston, D.E. 1986. Gas diffiisivity. p. 1089-1102. In A. L. Page (ed).. Methods of Soil Analyses, Part I. Physical and Mineralogical Methods. Agronomy Monograph 9< ASA, Madison, WI. Rose, N. L. 1996. Inorganic fly-ash spherules as pollution tracers. Environmental Pollution 91:245-252. Rosenberry, P., R. Knutson, and L. Harmon. 1980. Predicting the effects of soil depletion from erosion. J. Soil Water Conserv. 35:131-134. Schumacher, T.E., M.J. Lindstrom, D.L. Mokma and W.W. Nelson. 1994. Corn yield erosion relationships of representative loess and till soils in the North-Central United States. Soil Water Conserv. 49:343-348. Schwab, G.O., G.S. Taylor and J.L. Fouss. 1966. Crop response from tile and surface drainage. Soil Sci. Soc. Am. Proc. 30:634-637. Shaffer, M.J.. i".E. Schumacher, and C.L. Ego. 1994. Long-term effects of erosion and climate interactions on corn yields in the North Central Region. J. Soil Water Conserv. 49:272-275. Shrader, W.D., and G.W. Langsdale. 1979. Effects of soil erosion on productivity. In Frontiers in Conservation. Soil Cons. Soc. Am., Ankeny, LA. Smith, Jeffrey L., Jonathan J. Halvorson, and Robert I. Papendick. 1993. Using multiple-variable indicator kriging for evaluating soil quality. Soil Sci. Soc. Am. J. 57:743-749. Soil Survey Staff. 1981. Soil Survey Manual. National Soils Handbook. Govt. Printing Office, Washington, D.C., USA. Soil Survey Staff. 1993. Soil survey manual (Revised). USDA-SCS, U. S. Gov't Printing Office, Washington, DC. Spomer, R.G., and R.F. Piest. 1982. Soil productivity and erosion of Iowa loess soils. Trans. of the A.S.A.E. 25:1295-1999. Stone, J.R., J.W. Gilliam, D.K. Cassel, R.B. Daniels, L.A. Nelson, and H.J. Kleiss. 1985. Effects of erosion and landscape position on the productivity of Piedmont soils. Soil Sci. Soc. Am. J. 49:987-991. U.S. Congress. 1985. Food Security Act of 1985. P.L. 99-198. 99th Congress. Washington, D.C. USDA Staff. 1981. Soil and Water Resources Conservation Act program report and environmental impact statement. U.S. Gov't. Printing Office. 0-732-254/2680. Whitney, R.S., R. Gardner, and D.W. Robertson. 1950. The effectiveness of manure and commercial fertilizers in restoring the productivity of subsoils exposed by leveling. Agron. J. 42:239-245. Wark, K., and C. F. Wamer. 1976. Air pollution: Its origin and control. I. E. P., New York. 519p. Wischmeier, W.H. and D.D. Smith. 1978. Predicting rainfall erosion losses - a guide to conservation planning. Agr. Handbk. No. 537. U.S. Dept. Agr., Washington, D.C. 58pp.