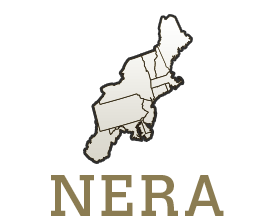
NE1021: Hydropedology: Genesis, Properties, and Distribution of Hydromorphic Soils
(Multistate Research Project)
Status: Inactive/Terminating
NE1021: Hydropedology: Genesis, Properties, and Distribution of Hydromorphic Soils
Duration: 10/01/2004 to 09/30/2009
Administrative Advisor(s):
NIFA Reps:
Non-Technical Summary
Statement of Issues and Justification
Because of the environmental and economic importance of wetlands and coastal resources to northeast, pedologists in this region have been developing expertise in the identification, characterization, classification, and land use interpretations for soils within these areas. In this project, we will establish a framework for the systematic study of saturated, hydric, and subaqueous soils across the northeastern US. This project will focus on: documenting the physical, chemical and morphological characteristics of these soils; establishing soil-landscape and soil-vegetation relationships; and developing an understanding of how these characteristics and relationships vary across the region and with changes in land use.
Soil interpretation rating guides (developed by the Natural Resource Conservation Service, NRCS) list over 30 land use decisions that are dependent upon knowing the depth to seasonal saturation (Soil Survey Staff, 1996). Some of the more important interpretations based on the depth to seasonal saturation include the construction of buildings and roads, surface application of waste, and the use of on-site waste disposal systems (ISDS). Water table depth is also a key factor in identification of wetlands (Environmental Laboratory, 1987). Wetland delineation is a million dollar industry and identification of hydric soils is essential in the delineation process. Of particular interest is identifying the variation in seasonal tables in soils that are wet, but where certain properties of the soil mask the typical morphology found in most wetland soils. These so called "problem soils" may include sandy soils, soils derived from Triassic redbed sediments, carboniferous and other dark parent material soils, soils formed in pre-weathered parent materials, soils having episaturation, and certain soils in floodplains. These problem areas occur across the entire region and need to be addressed from such a perspective.
Inventories of continuously submerged soils, referred to as "subaqueous soils" have been completed in estuarine environments in Maryland (M. Rabenhorst, PI) and Rhode Island (M. Stolt, PI), and are in progress in Maine (L. Osher, PI) and Delaware (M. Rabenhorst, PI). These resource inventories are providing the template for other near shore soil surveys that will be completed in estuarine environments throughout the US. Subaqueous soil inventories will provide numerous interpretations for coastal managers. King (2003) listed over 15 different subaqueous soil interpretations that federal, state, local agencies, and private companies have requested information about. Some of the most common requests have been in regard to: clam stocking, sustainable shellfish production, effects of dredging on benthic ecology, tidal marsh protection and creation, horseshoe crab habitat, dune maintenance/replenishment, and submerged aquatic vegetation (SAV) restoration. Little is known of these subaqueous soils and the associated interpretations. Nothing is known regarding how these interpretations vary across the region.
One of the most important interpretations from an inventory of subaqueous soils may be SAV restoration. Submerged aquatic vegetation such as eelgrass provide nursery habitat for economically important fin and shell fish and are important for sediment and nutrient filtering, nutrient cycling, and buffering wave effects. Aerial coverages of eelgrass beds have severely declined over recent years. Therefore, eelgrass restoration has become a focus of many coastal managers. Eelgrass revegetation and restoration projects cost on the order of $100,000 per acre, but few of these projects have been successful. It is highly likely that the projects fail because of site selection. Eelgrass revegetation sites are commonly located where past eelgrass meadows had been, not at sites where present soil conditions are optimum for success. However, loss of eelgrass tends to result in erosion of the submerged soils. The subsequent soils in the intertidal could be significantly different than those that supported the eelgrass. A detailed knowledge of the relationship between subaqueous soil properties and submerged aquatic vegetation is essential for improving the success of eelgrass revegetation efforts. An understanding of the eelgrass-subaqueous soil system will help resource managers identify the sites where revegetation efforts can be most successful (Bradley, 2001). Although eelgrass is the dominant SAV across the northeast region, knowledge of how well eelgrass-subaqueous soil relationships hold across the region with the breadth of tidal ranges and gradient of temperatures is unknown.
Saturated soils store significantly more carbon than well-drained soils per unit area. Most investigations, however, focused on quantifying soil carbon sequestration in better drained landscapes. Studies of carbon storage and loss in fresh water (Garnett et al. 2001) and coastal (Choi 2001) marshes are an excellent start, but these only describe a small subset of organic wetland soils. While marshes and the organic soils that support them are important ecosystems, the majority of subtidal soils are mineral soils. Very little is known about the amount of C sequestered in these subaqueous soils. As a result, predicting the impact of regional scale land use change, climate change, or rising sea levels on C storage in coastal zone is difficult at best. The results of this research will enable us to improve our estimates of the amount of carbon stored in seasonally saturated, wetland, and subaqueous soils in the northeastern US. In addition, the work will better enable us to model the effects of land use and climate change on soil C pools in seasonally saturated or hydric soils.
Working within the proposed regional framework will allow for testing of hypotheses regarding carbon storage, soil-water table relationships, and subaqueous soil characteristics across climatic gradients, across parent material types (coastal plain, residual, and glacial), and for the subaqueous soils and marsh settings across a diverse range in daily tidal fluctuations. For example, are subaqueous soil interpretations developed for restoration of eelgrass in Maryland estuaries the same for estuaries in Maine? Testing such a hypothesis is not possible for a single investigator working within a single state and must be done across the region. Addressing these questions within a regional framework is also critical because the major agencies that use the soils information that pedologists collect, such as USDA-NRCS, ACOE, EPA, all work in a region-wide context. In addition, working groups such as the New England Hydric Soil Technical Committee and Mid-Atlantic Hydric Soils Committee (see NEHSTC, 2004 and MAHSC, 2004 as examples of their work), who offer guidance to regional regulatory bodies like the New England Water Pollution Control Commission (http://www.neiwpcc.org/), need soils information that is not restricted by state boundaries. Data gathered, relationships that are established, and interpretations that are made are therefore much more meaningful to the user if the science was tested within a region-wide context.
One of the goals of this project is to develop testable hypothesis and collect critical data in order to strengthen our standing in a very competitive field of scientists pursuing funds related to wetlands, environmental quality, global change, coastal environments, and natural resources. The proposed multi-state project meets the USDA-NRI criteria of studies that "address key problems of regional and multistate importance" and will strongly advance our efforts as we compete for funds from the NRI in the area of Natural Resources and Environmental Quality. As an example, investigators working on this multistate project proposal are preparing proposals that focus on subaqueous soils for the Soils and Soil Biology program. In addition, this proposal will provide additional leverage as we strive to compete for funds directed toward water quality, restoration, ecosystem sustainability, and coastal resource initiatives from agencies such as EPA, NOAA, ACOE, and Sea Grant. In the end the proposed project will support our long-term goal to provide soils based information to natural resource managers for determining how resources and ecosystems should be managed to insure sustainability and health.
Related, Current and Previous Work
This project is aimed at improving our understanding of the processes, characteristics, and interpretations of saturated, hydric, and subaqueous soils and establishing a framework for the integration of studies investigating soils from a hydropedological approach throughout the northeastern US. A review of current and proposed multi-state research projects found only one project where there would be any possible overlap. The project (NC temp 921) is studying "Carbon sequestration and distribution in soils of eroded landscapes". The proposed hydropedology multi-state project is concerned with carbon sequestration, but these studies will be focused in saturated, hydric, and subaqueous soils. Thus, the likelihood that the proposed studies will overlap with other multi-state projects is minimal and the efforts proposed will begin to close the gap in the regional understanding of hydrologically linked soils.
Objectives
-
Determine frequency and duration of water table depths of wetland soil and agricultural soils with imperfect drainage. Address interpretation problems between measured hydrology and vegetation characteristics, and field hydric soil indicators as used by regulatory personnel.
-
Characterize the morphology, chemistry and physical properties of subaqueous soils. Develop terminology that will be used by soil scientists to describe these soils, and propose adaptations to Soil Taxonomy for subaqueous soil characterization.
-
Quantify carbon sequestration in seasonally staurated, hydric and subaqueous soils of of the northeastern US. Estimate changes in C-storage and other pedological process in response to land use change and predicted sea-level rise.
Methods
The research outlined in this proposal will rely on the efforts and expertise of the members of the hydropedology project committee. The focus areas of each participant, as discussed during proposal development, are outlined below. These focus areas are meant to be flexible so that new collaborators may join the project. ----------------------------------------------------------------------------------- HYDROPEDOLOGY FOCUS AREA (INVESTIGATORS) Hydric Soil and Seasonal Saturation Indicators: (Baker, Ciolkosz, Galbraith, Lin, Rabenhorst, Stolt, Vasilas, Veneman) Subaqueous Soil Mapping, Inventory, and Classifcation: (Needelman, Osher, Rabenhorst, Stolt, Veneman) Carbon Sequestration: (Galbraith, Lin, Needelman, Osher) --------------------------------------------------------------------------------------- To keep track of project activities, maintain standard protocols, and highlight project accomplishments an interactive website is under development that will be housed at the Soil and Water Geospatial Analysis Lab (SAWGAL). The website will help maintain strong ties and partnerships within the project participants and serve as a source of information to those interested in the application of pedology to environmental and land use issues in the northeast. We acknowledge that both soil and water are connected across the landscape in a seamless pattern from the seasonally-saturated soils of the upland to the continuously inundated subaqueous soils along the coast. Thus, by taking a hydropedological approach across the northeast region, we hope to study the cause and effect relationships between water and soil along this continuum. To do this, we will study a gradient of representative soils based on wetness: seasonally saturated soils, hydric soils, and subaqueous soils. In addition, we will focus on one ecosystem function along the continuum-carbon sequestration. Sites will be chosen so that representative areas across the region are studied. Standard procedures, protocols and analytical methods will be followed by each investigator to insure that results are comparable across the region. These methods are detailed below. HYDRIC AND SATURATED SOIL MONITORING AND IDENTIFICATION Using various existing (NRCS, state) sources we will identify problem hydric soils in the northeast (ie. sandy soils, soils derived from red Triassic or Paleozoic sediments, carboniferous and other dark parent material soils, soils formed in pre-weathered parent materials, soils having episaturation, and certain soils in floodplains). Based on this inventory we will select representative sampling and monitoring sites in cooperation with NRCS personnel and members of the federal and regional hydric soil committees. Sites chosen to be monitored will be representative of the indicators and/or soil conditions of concern. This screening can be accomplished by examining a number of sites in a reconnaissance survey which have the presupposed conditions. The most representative sites will be chosen to monitor. Another alternative is to use previous experience in the area that suggests that the sites meet criteria established for the indicators or conditions. At least three sites will be chosen to monitor for each indicator and/or condition of concern. Each site will have locations that are obviously wetland, obviously upland, and a transitional area that can be monitored without problem. Each site will be instrumented and monitored using methods similar to those described by Faulkner et al. (1989) and NHSTC (2004). Water table wells will be installed at the wetland, upland, and transition zone along a transect at each site. Wells will be constructed of slotted PVC pipe with a well point on one end and a PVC cap on the other. Each well will be equipped with a Maximum Water Table Level Device to measure water table levels. This system has been shown to provide very detailed water table fluctuation patterns when water table data loggers are used to establish water table rise and fall rates following a precipitation event (Morgan and Stolt, 2004). We will use data loggers set at 6 hour intervals to establish water table rise and fall rates following precipitation. Wells will be installed by augering to the lower depth of the well, inserting the well in the auger hole, back-filling with sand, and capping the well with bentonite. Water table levels will be monitored with a voltmeter system. Soil temperature will be measured at each site at depths of at 12.5 cm in sandy soils, 25 cm in loamy soils, and 50 cm in all soils with a soil thermometer. Selected sites will have temperature monitored with temperature loggers. Redox potential will be measured at 12.5 cm in sandy soils, 25 cm in loamy soils at each location. Five platinum electrodes will be used to determine average redox potential at a given location. Redox potential will be measured with voltmeter that is attached to a reference electrode (calomel cell) and the platinum electrode. A salt agar bridge will be installed at each location for the calomel cell. Samples will be collected at 12.5 cm in sandy soils, 25 cm in loamy soils to test for ferrous Fe using alpha alpha dipyridel. The intervals between monitoring will be based on soil temperature data in correspondence with the concepts and definitions of the "growing season". Monitoring will be at one month intervals except late fall and spring where measurements will be made once a week. Once a week monitoring will begin in the fall when the shallow soil temperature (12.5 cm in sandy soils and 25 cm in loamy soils) reaches 40 degrees F. When the soil temperature at 50 cm reaches 40 degrees F, once a month monitoring will resume. In the spring when the shallow temperature reaches 40 degrees F once a week monitoring will begin. We will resume monitoring once a month when the 50 cm soil temperature is greater than 40 degrees F. Each monitoring station will have a detailed profile description. Samples will be collected from the depths that redox potential measurements are being made to determine soil pH and organic carbon content. Selected sites will be sampled to examine extractable Fe. Detailed vegetation data will be collected at each monitoring station. Precipitation amounts will be recorded with rain gauges and climate data collected at nearby weather stations. SUBAQUEOUS SOILS In the proposed studies we will address specific questions in regard to subaqueous soils including: soil-landscape relationships; soil-SAV relationships; and soil taxonomy, diversity, distribution, and interpretation. Sites will be chosen to address one or more of these questions and to establish how these attributes and relationships vary across the northeast. Site selection will be based on a number of factors including: geomorphic setting; depositional environment; presence, absence, density, and type of SAV; type of estuary or subestuary; and the types of landforms and soil-parent materials on the adjacent upland environment. Each site chosen to study should be representative of the estuaries or subestuaries in the study area. Terminology for landforms and parent materials will follow those established by Soil Survey Staff (2002) and Stolt (2003). Amendments to the terminology will be discussed and agreed upon among the project participants. A submerged topography (bathymetric) map, at a minimum scale of 1:10,000-scale and with contour intervals of 30 cm or less, will be constructed for each study site (Demas, 1998; Bradley, 2001). These bathymetric maps will be used as a base map to delineate landforms and landscape units (Demas and Rabenhorst, 1998; Bradley and Stolt, 2002) and to derive landscape attributes such as percent slope, land-surface shape, and geographic location. Bathymetric maps will be created using a combination of fathometer, GPS, and software (ie. Geolink, Inc) to collect the depth of the water at known locations (Demas, 1998; Demas and Rabenhorst, 1998; Gorman et al., 1998. Boat speed will be set so that bathymetric data will be collected at 20 m intervals along transects. We will simultaneously collect tide levels from fixed points of known elevation from 3 areas within each estuary. The 3 locations will be: at the mouth of the estuary, at the farthest distance from the mouth, and at a midpoint. These data will be collected with data loggers (Infinities, Inc) set to record levels at 30 minute intervals. The tide level data will be used to adjust for tidal fluctuations during collection of the bathymetric data. The tide-normalized bathymetric data will be critically analyzed to identify and remove outliers. A minimum of 10 data points per hectare will be used to develop the bathymetric maps. Contour lines will be drawn over the digital orthoquadrangle image by utilizing a Triangular Irregular Network (TIN) model within the 3-d Analyst extension in Arc View (Environmental Services Research Institute, 1999). Soils will be examined at multiple locations within each landscape unit. Sampling locations will be chosen in order to capture both the variability and extent of soil types within each landscape unit. Sampling will be done either by wading or from a boat. The spatial location of each description will be recorded using a Global Positioning System (GPS). Soils will be described using samples collected with a standard bucket auger. For very soft and fluid material (high n-value soils) and organic soils, a MacCauley peat sampler will be used. Based on these descriptions, representative soils will be sampled for laboratory analysis from each landscape unit using a vibracorer (Lanesky et al., 1979), standard bucket auger, or a McCauley peat sampler. Core barrels will be cut open length-wise and the soils described following standard procedures (Soil Survey Staff, 1993). All soil samples (vibracore, bucket auger, and McCauley sampler) will be frozen until needed for lab analysis. In studies of subaqueous soil-SAV relationships, SAV cover will be determined for representative soil sampling and description locations using a point-intercept transect (Bauer, 1943). These determinations will be based on point counts of SAV by a diver at one-meter intervals along a fifteen-meter transect. Soil samples will be analyzed for percent coarse fragments, percent shell fragments (larger than 2 mm), pH, electrical conductivity, particle size distribution (PSD), levels of sulfides, CaCO3, and organic carbon. Particle size analysis will follow methods outlined in Gee and Bauder (1986). Salts will be removed through dialysis tubing. The clay fraction will be determined by pipette and sand fractions separated by sieving. Percent coarse fragments (rocks and shell fragments larger than 2 mm) will be determined by weight. We will make measurements of electrical conductivity and pH following standard and modified Soil Survey Staff (1992) guidelines. Electrical conductivity will be measured on saturated paste extracts using a conductivity meter. Due to small sample sizes, 10 g of soil will used to make a saturated paste and the pore-water separated using a centrifuge. Measurements of pH will be made on thawed samples in a 1:1 soil-to-deionized water mixture. Samples will be moist incubated at room temperature for approximately 2 months. During this time the pH will be recorded on a weekly basis to determine potential acidity. Levels of organic carbon and CaCO3 will be determined using a C analyzer (ie. Carlo-Erba, Milan, Italy). Acid-volatile and Cr-reducible sulfides (AVS and CRS, respectively) will be determined following procedures similar to those described by Cornwell and Morse (1987), Cline (1969), Fossing and Jorgenson (1989), and Ulrich et al. (1997). Amendments to these methods are described in Bradley (2001). Soils will be classified within the Soil Taxonomy system (Soil Survey Staff, 1999) and amendments to Soil Taxonomy will be developed across the region to accommodate the unique characteristics of subaqueous soils. CARBON SEQUESTRATION In the proposed studies we will examine carbon sequestration along the hydrologic gradient we have established across the region. Potential sites will be screened based on soils and land use data. GIS layers will be utilized in the initial screening procedure. Soils that have a considerable extent within the area will be considered first for study. Because of the effects of land use on carbon pools, study locations for the seasonally saturated and palustrine hydric soils components of the hydrologic gradient will be focused on forested ecosystems. Forest age will be estimated from achieved remote sensing data such as aerial photographs. Sites that have been in forest for more than 40 years will be preferentially chosen for study. Selected locations may also be sampled to quantify carbon pools in soils with agriculture or urban land covers to compare with forested soils of the same soil type. Carbon storage in tidal and subtidal soils will be examined in representative estuaries or subestuaries in the study area. Marshes or subaqueous soil landscape units that show substantial evidence of filling or dredging will be avoided. Field visits will be used to confirm that the GIS soil and vegetation coverages were correct and to collect detailed site information. Soils will be investigated at each reconnaissance site to insure that the location is representative of soil type or subaqueous landscape unit before the site is chosen for study. At each selected site, soils will be sampled at 5 random locations within the soil landscape unit (mapping unit) boundary. The exact spatial coordinates of each sampling location will be recorded with a global positioning system. Soils at each sampling location will be described from auger borings, McCauley peat samples, or vibracore samples (Schoeneberger et al. 2002). Soil samples will be collected by master horizon (O, A, B, and C) for each sampling location. Undisturbed samples of shallow organic horizons will be collected from the forested sites as 15 x 15 cm blocks for analysis and bulk density measurements. Undisturbed samples from mineral horizons from all sites will be collected to 1 m using a split core sampler or a vibracore (Lanesky et al. 1979). Deep organics or high n-value materials will be sampled with a McCauley peat sampler. Bulk density will be determined from the undisturbed samples. Total carbon and nitrogen will be determined using an automated CN analyzer (ie. Carlo-Erba, Milan, Italy; Nelson and Sommers, 1996). Bulk density measurements will be used to convert carbon values to a mass per area basis so that pools can be summed over the entire landscape (ie. Homann and Grigal, 1996). Selected subaqueous, tidal, and organic soils may be sampled to determine sedimentation rate using Lead 210 (Goldberg, 1963). Samples from sequential depths will be analyzed for %C and %N, stable C and stable N isotope composition. The age of the organic matter in specific horizons will be determined using radiocarbon analysis (14C) of representative samples (Paul et al. 1997). The turnover time of the organic matter will be estimated according the methods described in Wang and Hsieh. (2002). Stable carbon and nitrogen isotope ratios in soil/sediment organic matter will be used to determine the source of that organic matter (Boutton 1991, Hemminga and Mateo 1996, Keats et al, 2004). Relative contribution of each carbon source will be estimated using a three component linear, mass balance, and mixing model. Most of the published C biogeochemistry studies of estuaries have not included data from both 14C and 13C analyses of organic matter (Graham et al. 2001, Goni et al. 2003, Bouillon et al. 2003). The combination of these analyses allows for the identification of sources of organic matter, an estimate of their time of deposition, and the calculation of turnover times, (Raymond and Bauer 2001). Over the long term, this research will increase our knowledge of the role that estuaries play in storing C fixed from the atmosphere by terrestrial plants.Measurement of Progress and Results
Outputs
- An annual project report highlighting the results for the previous year will be made available on the project website, and forwarded to the participants in the related project focus areas identified above.
- Participants will submit appropriate finding research findings for publication in peer reviewed journals.
- Amendments to Soil Taxonomy, Hydric Soil Indicators, and Terminology for landforms and parent materials will be composed and submitted for consideration to the appropriate organizations.
- A final report will be issued at the conclusion of the project.
Outcomes or Projected Impacts
- This research will result in improved region-wide guidelines for the identification of soils that although wet, do not display typical hydromorphic features associated with seasonally saturated or hydric soils. This will lead to improved decision-making by state regulators concerning on-site sewage disposal, wetland, and similar environmental restrictions. The research results also provide needed data to municipalities and conservation commissions to improve land use decisions.
- Sub-aqueous soil characterization data and inventories will augment soil survey data and mapping outputs from MLRA soil survey offices within the region. This will result in enhanced selection of sites for submerged aquatic vegetation restoration and directly improve fish nursery, water filtration and nutrient cycling functions in estuaries.
- The sub-aqueous soil research results will improve interpretations for coastal areas throughout the US.
- The C-data from the resource inventories will allow for better estimates of carbon sequestration in palustrine, near shore, and recently submerged wetlands, and better predict the impact of land use change and sea level rise on C-storage and loss from these ecosystems. This will provide input into carbon cycling and global warming models that has not been previously available.
Milestones
(2004): Establish project website documenting project objectives and research deliverables for communication to the committee members. Assign participants charges within each research focus area based on his or her expertise and interests. Evaluate field sites in a set of reconnaissance surveys to identify appropriate field sites for detailed studies.(2005): Using criteria outlined in the methods section choose appropriate sites for detailed studies. Initiate instrumentation (ie. hydrologic), mapping (ie. bathymetric data collection), and sampling strategies (ie. carbon storage). Visit selected sites during biannual Northeast Pedology Fieldtrip. Update web site to include site information and discussions during the regional fieldtrip to selected sites.
(2006): Continue instrumentation (ie. hydrologic) and mapping (ie. bathymetric data collection). Collect hydrologic data at instrumented sites. Construct contour maps from sites where bathymetric and tidal data have been analyzed and normalized. Describe and sample subaqueous soils within various landscape entities. Begin characterization efforts. Begin to analyze morphologic data from hydric and seasonally saturated soils with hydrologic data. Collect and analyze carbon sequestration data. Visit selected sites during the International Soil Science meetings tours. Update web site to include site and monitoring information and discussions during the international fieldtrip to selected sites.
(2007): Continue to collect hydrologic data at instrumented sites. Describe and sample subaqueous soils within various landscape entities. Continue characterization efforts. Continue to analyze morphologic data from hydric and seasonally saturated soils with hydrologic data. Collect and analyze carbon sequestration data. Visit selected sites during biannual Northeast Pedology Fieldtrip. Update web site to include new monitoring and analytical information and discussions during the regional fieldtrip to selected sites.
(2008): Complete hydric and saturated soil, subaqueous soil, and carbon sequestration analysis. Synthesize results across the region. Write final report and other output works.
Projected Participation
View Appendix E: ParticipationOutreach Plan
Results from the proposed multistate project activities will be published as project reports, on the project web site, and as peer-reviewed publications. Participating members involved in undergraduate teaching, graduate student advisement, and extension activities associated with Land Grant Universities will promote the general dissemination of knowledge developed from the proposed project activities. Research sites will be visited on local, regional, and national pedology, hydric soil, and soil-environmental science fieldtrips and workshops. Northeast Pedology Fieldtrips have been run at least every two years since 1985. Participants include National Cooperative Soil Survey personnel from the NE region and graduate students from participating schools. Annual fieldtrips are also run by the New England Hydric Soils Technical Committee and the Mid-Atlantic Hydric Soils Committee. These committees are made up of university faculty, consulting soil scientists, NRCS soil scientists, and state and regional regulators.
Organization/Governance
The core membership in the new multi-state project will likely come from the current NEC1006 Multistate Research Coordinating Committee and Information Exchange Group. Preliminary discussions within the current NEC1006 committee and exchange group have demonstrated considerable support for the multi-state project. The current NEC 1006 participants are: Edward Ciolkosz (Penn State University), James Baker (Virginia Tech), Peter Veneman (University of Massachusetts), Martin Rabenhorst (University of Maryland), Bruce Vasilas (University of Delaware), Mark Stolt (University of Rhode Island), John Galbraith (Virginia Tech), Laurie Osher (University of Maine), Brian Needelman (University of Maryland), and Henry Lin (Penn State University). A Chair, a Chair-elect, and a Secretary will be selected from the above participants. Representatives from the member institutions will meet at least annually to assign tasks and review progress on the current research project Additional participants with expertise in pedology, mineralogy, soil ecology, hydrology, soil-environmental science, and other related disciplines will be invited to join the project.
Literature Cited
Bauer, H.L. 1943. The statistical analysis of chaparral and other plant communities by mean of transect samples. Ecology 24:45-60.
Boutton, T. W. 1991. Stable carbon isotope ratios of natural materials: II. Atmospheric, terrestrial, marine, and freshwater environments. In Coleman, David C., and Brian Fry (editors), Carbon Isotope Techniques. Academic Press, Inc. Harcourt Brace Jovanovich, New York, New York.
Bouillon, S, F. Dahdouh-Guebas, AVVS Rao, AVVS, N. Koedam, and F. Dehairs. 2003. Sources of organic carbon in mangrove sediments: variability and possible ecological implications. Hydrobiologia 495:33-39.
Bradley, M.P. 2001. Subaqueous soils and subtidal wetlands in Rhode Island. Masters Thesis. Department of Natural Resources Science, University of Rhode Island, Kingston.
Bradley, M.P., and M.H. Stolt. 2002. Evaluating methods to create a base map for a subaqueous soil inventory. Soil Sci. 167:222-228.
Bradley, M.P., and M.H. Stolt. 2003. Subaqueous soil-landscape relationships in a Rhode Island coastal lagoon, Soil Science Society of America Journal 67:1487-1495.
Cline, J. 1969. Spectrophotometric determination of hydrogen sulfide in natural waters. Limnology and Oceanography 4:454-458.
Cornwell, J.C., and J.W. Morse. 1987. The characterization of iron sulfide minerals in anoxic marine sediments. Marine Chemistry. 22:193-206.
Choi, Y.H. 2001. Vegetation succession and carbon sequestration in a coastal wetland in northwest Florida: evidence from carbon isotopes. Global Biogeochemical Cycles 15:311-319.
Demas, G.P. 1998. Subaqueous soil of Sinepuxent Bay, Maryland. PhD Dissertation. Department of Natural Resource Sciences and Landscape Architecture, University of Maryland, College Park, MD.
Demas, G.P., and M.C. Rabenhorst. 1998. Subaqueous soils: a resource inventory protocol. In Proceedings of the World Congress of Soil Science, 16th. August 20-26th 1998, Symposium 17 on CD. International Society of Soil Science, Montpelier, France.
Environmental Laboratory. 1987. Corps of Engineers wetland delineation manual. U.S. Army Engineer Waterways Experiment Station, Vicksburg, MS, USA. Technical Report Y.
Environmental Services Research Institute. 1999. ArcView, ArcInfo Geographic Information System. ESRI Inc, Redlands, CA.
Faulkner, S. P., W. H. Patrick, Jr., and R. P. Gambrell. 1989. Field techniques for measuring wetland soil parameters. Soil Science Society of America Journal 53:883-890.
Fossing, H. and B.B. Jorgensen. 1989. Measurement of bacterial sulfate reduction in sediments: evaluation of a single-step chromium reduction method. Biogeochemisty 8:205-222.
Garnett, M. H., P. Ineson, A.C. Stevenson, and D.C. Howard. 2001. Terrestrial organic carbon storage in a British moorland. Global Change Biology 7:375-389.
Gee, G.W. and J.W. Bauder. 1986. Particle size analysis. p. 383-409. In Klute, A (ed). Methods of Soil Analysis: Physical and Mineralogical Methods, Part 1, 2nd edition. Soil Science Society of America, Inc, Madison, WI.
Goldberg, E.D. 1963. Geochronology with 210Pb. Radioactive Dating. International Atomic Energy Agency.
Goni, M.A, M.J. Teixeira, and D.W. Perkey. 2003. Sources and distribution of organic matter in a river-dominated estuary (Winyah Bay, SC, USA). Estuarine Coastal and Shelf Science, 57:1023-1048.
Gorman, L., A. Morang, and R. Larson. 1998. Monitoring the coastal environment; Part IV: mapping, shoreline changes, and bathymetric analysis. Journal of Coastal Research 14:61-92.
Graham, M.C., M.A. Eaves, J.G. Farmer, J. Dobson, and A.E. Fallick. 2001. A study of carbon and nitrogen stable isotope and elemental ratios as potential indicators of source and fate f organic matter in sediments of the Forth Estuary, Scotland. Estuarine, Coastal and Shelf Science, 52:375-380.
Hemminga, M.A., and M.A. Mateo. 1996 Stable Carbon isotopes in seagrasses: variability and ratios and use in ecological studies. Marine Ecology Prog. Series 140:285-298.
Homann, P.S., and D.F. Grigal. 1996. Below-ground organic carbon and decomposition potential in a field-forest glacial-outwash landscape. Biology and Fertility of Soils. 23:207-214.
Keats, R, L Osher, and H. Neckles. 2004. The effect of nutrient loading on an estuarine foodweb: a stable isotope approach. Estuaries (in press).
Kelly, R.P. and S.B. Moran. 2002. Seasonal changes in groundwater input to a well-mixed estuary estimated using radium isotopes and implications for coastal nutrient budgets. Limnology and Oceanography 47:1796-1807.
King, P. 2003. Soil Survey of Delaware Update. USDA-NRCS, Dover, DE., In preparation.
Lanesky, D.E., B.W. Logan, R.G. Brown, and A.C. Hine. 1979. A new approach to portable vibracoring underwater and on land. Journal of Sedimentary Petrology 49:654-657.
Mid-Atlantic Hydric Soils Committee 2004. A guide to hydric soils in
the Mid-Atlantic Region, ver. 1.0. L.M. Vasilas and B.L. Vasilas
(eds.). USDA, NRCS, Morgantown, WV.
Morgan, C.P., and M.H. Stolt. 2004. A comparison of several approaches to monitor water table fluctuations. Soil Science Society of America Journal (In press).
National Technical Committee for Hydric Soils. 2004. Technical Note 11: Technical Standards for Hydric Soils. http://soils.usda.gov/use/hydric/ntchs/tech_notes/index.html
Nelson, D.W. and L.E. Sommers. 1996. Total carbon, organic carbon and organic matter. p. 961-1010 In D.L. Sparks et al. (eds.), Methods of soil analysis. Part 3; Chemical Methods, Book Series 5, Soil Science Society of America, Madison, WI.
New England Hydric Soils Technical Committee. 2004. Field Indicators for Identifying Hydric Soils in New England, New England Interstate Water Pollution Control Commission. Wilmington, MA.
Paul, E.A., R.F. Follett, S.W. Leavitt, A., Halvorson, G.A. Peterson, and D.J. Lyon. 1997. Radiocarbon dating for determination of soil organic matter pool sizes and dynamics. Soil Science Society of America Journal 61:1058-1067.
Schoeneberger, P.J., D.A. Wysocki, E.C. Benham, and W.D. Broderson. 2002. Field Book for Describing and Sampling Soils, Version 2.0. Natural Resources Conservation Service, National Soil Survey Center, Lincoln, NE.
Soil Survey Staff. 1992. Soil survey laboratory methods manual. Soil Survey Investigations Report No. 42, USDA-SCS, Washington, D.C.
Soil Survey Staff. 1993. Soil survey manual. Agric. Handbook No. 18, USDA-NRCS, U.S. Government Printing Office, Washington, D.C.
Soil Survey Staff. 1996. National Soil Survey Handbook, 430-VI, Natural Resources Conservation Service, U.S. Government Printing Office. Washington, D.C.
Soil Survey Staff. 1999. Soil Taxonomy: A basic system of soil classification for making and interpreting soil surveys. 2nd edition. USDA-NRCS, Agriculture Handbook 436. U.S. Government Printing Office, Washington, D.C.
Soil Survey Staff. 2002. Glossary of landforms and geologic materials. Part 629, National Soil Survey Handbook, USDA, Natural Resources Conservation Service, National Soil Survey Center, Lincoln, NE.
Stolt, M.H. 2003. Glossary of terms for subaqueous soils, associated landscapes, landforms, and related materials. Subaqueous Soils Committee Report, National Cooperative Soil Survey http://nesoil.com/sas/glossary.htm.
Ulrich, G.A., L.R. Krumholz, and J.M. Suflita. 1997. A rapid and simple method for estimating sulfate reduction activity and quantifying inorganic sulfides. Applied and Environmental Microbiology 63:1627-1630.
Wang, Y., and Yuch-Ping Hsieh. 2002. Uncertainties and novel prospects in the study of the soil carbon dynamics. Chemosphere 49:791-815.