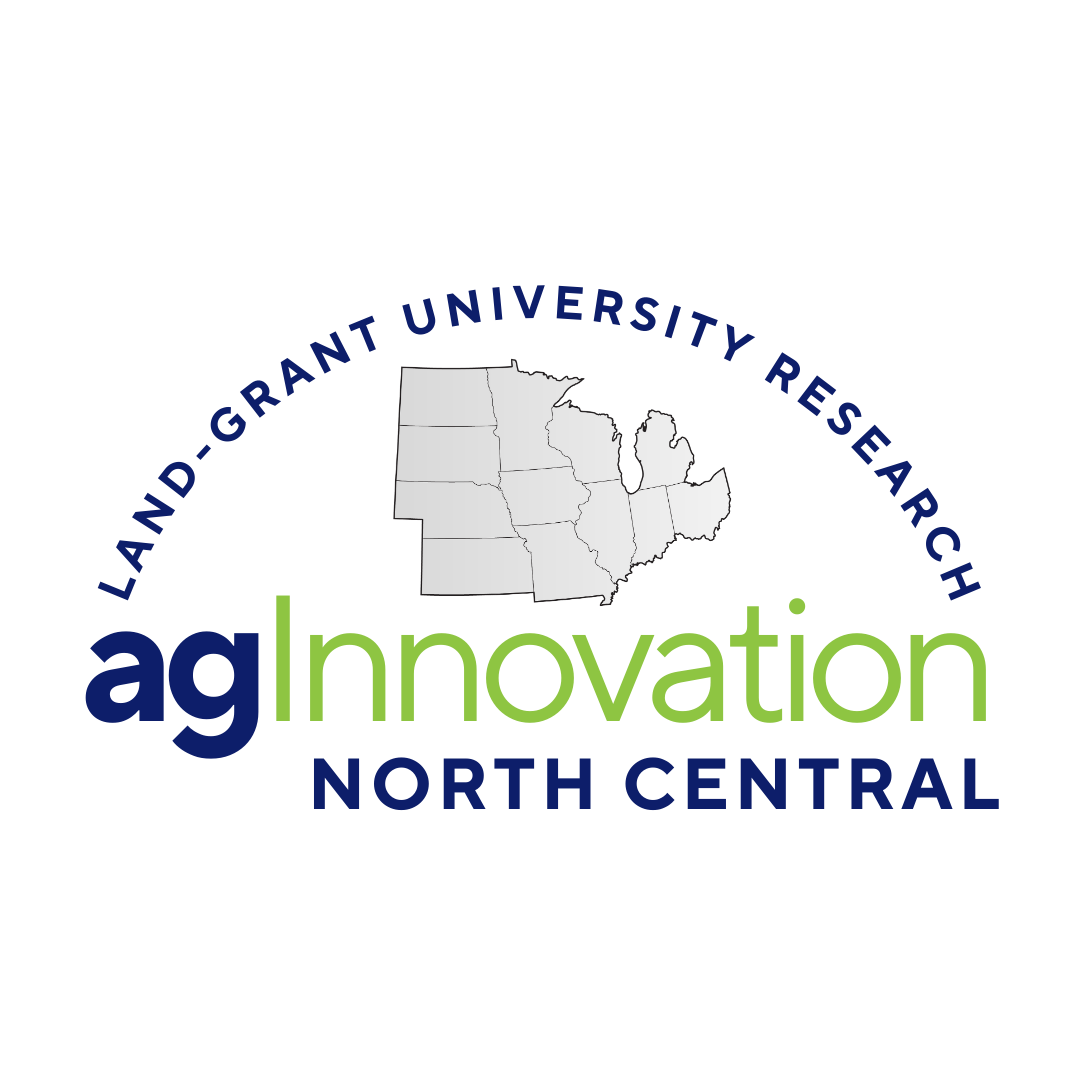
NC1019: Control of Emerging and Re-emerging Poultry Respiratory Diseases in the United States (was NC-228)
(Multistate Research Project)
Status: Inactive/Terminating
NC1019: Control of Emerging and Re-emerging Poultry Respiratory Diseases in the United States (was NC-228)
Duration: 10/01/2004 to 09/30/2009
Administrative Advisor(s):
NIFA Reps:
Non-Technical Summary
Statement of Issues and Justification
THE NEED AS INDICATED BY STAKEHOLDERS. Poultry meat production has been continually increasing and consumption has surpassed all other meats. Because of the efficiency and competitive nature of the poultry industry, the cost of poultry meat is very attractive to consumers. Furthermore, the National Chicken Council released results of a survey showing that chicken was preferred to beef and pork, in terms of versatility, taste, ease of preparation, being healthful and nutritious, price and consistency of quality. In 2000, per-capita annual consumption of chicken was 81 pounds, the highest of any of the major meats. In addition, annual per capita turkey meat consumption was approximately 18 pounds. Beef consumption was 69 pounds per person on a retail weight basis, while pork was 52 pounds per person. A survey conducted by Bruskin Research found that 89% of the respondents ate chicken at least once per week and 36% of the respondents consumed chicken three times per week or more. The poultry industry is a highly integrated growth industry and is a major contributor to animal agriculture. In 2002, broiler production was valued at $13.4 billion. The number of broilers produced was 8.6 billion, up 2% from 2001. Turkey production was valued last year at $2.7 billion, represented 7.4 billion pounds, compared with 7.2 billion pounds in 2001. Layers totaled 334 million and consisted of 274 million table or commercial-type egg birds, 58 million broiler breeders, and 2.8 million table egg breeders. The value of sales from chickens (excluding broilers) in 2002 was $49 million, up 6% from the $46 million a year ago. Chicken eggs are an important source of high quality protein and other nutrients in the diet. IMPORTANCE OF THE WORK, AND WHAT THE CONSEQUENCES ARE IF IT IS NOT DONE. Respiratory diseases are the major group of diseases affecting poultry. Consequently, losses induced by these diseases are of major economic impact on the producer, the local economy and the US economy. Many endemic respiratory infectious diseases in the USA continue to decrease the profitability of commercial poultry production. Viruses, bacteria and fungi cause respiratory diseases or interact to cause disease. Management of poultry is also a critical factor in controlling respiratory disease. One of the diseases, avian influenza (AI), has significant potential to impact the industry both in terms of animal losses and through export restrictions. AIV which is endemic in live poultry markets in the Eastern USA and in the Western USA will remain a significant threat to commercial poultry producers. A major AI outbreak occurred in commercial poultry in Virginia in 2002 resulting in $130 million in losses. Outbreaks in smaller commercial poultry populations occurred in Pennsylvania, Connecticut, Rhode Island, Minnesota and California. It has been estimated that approximately 16% of the total poultry production is exported (Economic Research Service, USDA; http://www.ers.usda.gov/Briefing/Poultry/). These export markets are subject to immediate restriction when poultry respiratory diseases such as avian influenza or exotic Newcastle disease (END) are diagnosed and reported. Since our last project was initiated, important new threats to poultry production have become a concern of the industry and require a focus of our project. A major epidemic of END occurred in California from September 2002-May 2003. Smaller outbreaks in non-commercial poultry occurred in Arizona and Nevada. An apparently unrelated END outbreak in backyard poultry took place in Texas. The agrobioterrorism threat to the food of Americans is a post 9/11 2001 reality. Two poultry pathogens, AIV and END are classified as agrobioterrorism agents. Our project will develop strategies for rapid diagnosis and control of END and AI. Considering the above developments and threats it is essential that the proposed studies should proceed to protect the nations food supply. THE TECHNICAL FEASIBILITY OF THE RESEARCH. The technical challenges posed by the goal of improved respiratory disease control are significant but approachable. Participants in the project have experience and training to undertake the work and complete the objectives. In addition, physical facilities, equipment, and other resources are committed by member institutions to guarantee the success of the proposed activities. THE ADVANTAGES FOR DOING THE WORK AS A MULTISTATE EFFORT. Control of respiratory infectious diseases lends itself to collaborative multistate research. The diseases are endemic in many if not all poultry producing states. Furthermore the challenges posed by the number of different disease etiologies and their complexities require a multistate effort. NC-228 SIGNIFICANT FINDINGS AND PRODUCTIVITY. The understanding of respiratory diseases of poultry has been advanced considerably through the current NC-228 project which merged in 2000 with the now defunct NE-138 project, Epidemiology and Control of Emerging Strains of Poultry Disease Respiratory Agents. Of notable mention are the advances made in rapid diagnostics for avian influenza and Newcastle disease viruses and genotype identification of infectious bronchitis virus and infectious bursal disease virus. DNA and recombinant vector based vaccine technologies have also moved forward at a dramatic pace and hold much promise for improved control of respiratory diseases. Studies with the newly recognized diseases turkey rhinotracheitis and chicken swollen head syndrome caused by avian pneumovirus have led to better understanding of the disease, development of diagnostic tools, and development of a vaccine that is used successfully to control the disease. The combination of these and other findings have led to a very productive first four years of the current project as indicated by the number of papers published or in press of which many include joint publications. Please refer to the Composite Annual Station Reports attached to this proposal for more infomation on findings and publications for the years 2000-2001, 2001-2002, and 2002-2003. WHAT THE LIKELY IMPACTS WILL BE FROM SUCCESSFULLY COMPLETING THE WORK. The overall impact of a successful outcome will be improved diagnosis and control of respiratory diseases that will benefit the poultry industry. Impact of the research will be derived from identification of disease agent reservoirs, factors involved in agent transmission to poultry, the development and delivery of differential immuno and gene based diagnostics, determination of infection status, rapid strain identification, evaluation of vaccines, and the design and implementation of eradication protocols for selected agents. The overall outcome of the project is to produce findings that enable poultry industry to remain competitive and profitable. The specific NCRA Crosscutting Research Areas and Priority Research Objectives addressed directly or indirectly by this NC-228 research proposal are: i. Agricultural Production, Processing and Distribution. Objective 1. Develop alternative agricultural production systems to enhance economic competitiveness in the rural landscape; and Objective 2. Develop improved animal, plant and microbial production, processing and marketing systems that are competitive, profitable and environmentally sound over the long term. ii. Genetic Resources Development and Manipulation (Genomics and Germplasm). Objective 1. Develop new genotypes that increase product value, enhance global competitiveness, improve human nutrition, nurture environmental quality and foster rural development.; Objective 2. Broaden and enrich the knowledge base about genomics; Objective 3. Collect, preserve, share, enhance and evaluate germplasm at the molecular, cellular and/or organismal levels; and Objective 5. Develop increased knowledge of the interactions and interrelationships of the various life forms. iv. Natural Resources and the Environment. Objective 7. Develop remediation systems to reduce agricultural, non-agricultural, and chemical waste contamination of soil, water and air; and Objective 10. Document the link between animal welfare/behavior, care and management and their environment. v. Economic Development and Policy. Objective 1. Develop profitable technologies and systems. Determine the potential profitability of production, processing and distribution technologies (innovations, i.e., agricultural information, technology, precision agriculture) that are environmentally sound and socially acceptable; Objective 2. Enhance U.S. global competitiveness. Enhance international market development by analyzing factors including the increasing adoption of agricultural biotechnology that determine U.S. competitiveness in global markets and analyze alternative policies to modify these factors to the advantage of U.S. agriculture; and Objective 3. Create new management decision-making models. Design optimal management systems for cropping systems, forest systems, non-cropped ecosystems, animal systems, whole farm and watershed systems, fishery and wildlife and data needs of agricultural businesses, research organizations and consumer groups. The specific CREES/GPRA Goals and Strategic Objectives addressed directly or indirectly by this NC-228 research proposal are: I. An Agricultural System That is Highly Competitive in the Global Economy. Objective1. Facilitate informed decisions by agricultural producers, policy officials, and other decision makers by developing and sharing knowledge promoting agricultural production and marketing; Objective 2. Expand the knowledge base leading to improvements in productivity and marketability, development of new and enhanced commercial products, and expansion of foreign and domestic market opportunities. Objective 3. Ensure the long-term economic viability and sustainability of production agriculture as it makes the transition from Federal subsidies to world market orientation; and Objective 4. Strengthen and coordinate the capabilities of the REE agencies to enable joint action and rapid response to emerging issues and problems in a global context. II. A Safe and Secure Food and Fiber System. Objective 1. Reduce the impact of threats to agricultural production by expanding the knowledge base needed to rapidly and effectively manage pests, disease, and natural disasters. IV. Greater Harmony Between Agriculture and the Environment. Objective 1. Promote sustainable agricultural production and enhance environmental quality by enabling producers to use cost-effective, environmentally friendly production practices and systems. V. Enhanced Economic Opportunity and Quality of Life for Citizens and Communities. Objective 1. Promote the effectiveness of rural policies and programs by (a) enhancing understanding of the conditions that promote economic opportunities, and (b) identify rural needs.; and Objective 2. Promote new businesses and growth in existing businesses, including farms and ranches, by transferring knowledge and technologies developed by or in partnership with REE agencies to private sector entrepreneurs.
Related, Current and Previous Work
A search of the CRIS project database using the agents studied in this proposal (avian influenza virus, infectious bronchitis virus, Newcastle disease virus, infectious bursal disease virus , infectious laryngotracheitis virus, Mycoplasma gallisepticum and Mycoplasma synoviae) as keywords found 57 projects, a mixture of applied and basic research. Thirty-one of these projects were with members of NC-228 and related directly to this project. Avian Influenza Virus (AIV). AIV is a Type A influenza virus that is endemic in certain wild bird species and often spreads to our domestic poultry species. The virus is extremely variable and has at least 15 hemagglutinin (H) and nine neuraminidase (N) antigenic subtypes. Influenza viruses may have any possible combinations of H and N subtypes. In poultry, AIV can be differentiated into two distinct groups based on their ability to cause disease. Highly pathogenic avian influenza (HPAI) is a systemic disease that can produce high morbidity and high mortality. Low pathogenic avian influenza causes a localized mucosal infection of the respiratory or enteric tract and is often characterized as having high morbidity but low mortality unless disease is exacerbated by other problems. Only the H5 and H7 subtype have been reported to cause HPAI, although most H5 and H7 viruses as well as all the other H subtypes cause the low pathogenic form of the virus. Avian influenza remains a recurring an important threat to the U.S. poultry industry both by causing disease as well as disrupting trade, particularly from disease outbreaks from H5 and H7 AIV, either LPAI or HPAI. Control of AIV in chickens and turkeys are accomplished though eradication of HP strains and some LP strains. In addition, vaccination has been used commonly used to control the disease in turkeys, and recently vaccination of chickens to control disease has become a more commonly used strategy. Currently, killed adjuvanted vaccines are the primary tools for vaccination, but increased interest in vaccines that would allow differentiation of infected from vaccinated birds (DIVA) or vaccines that can be more easily administered are an important research goal. Parameters for evaluating vaccines include shedding from the respiratory and digestive tracts, protection against clinical signs and death. Also of concern is establishing the level of virus in feces and carcasses to minimize possible transmission during farm clean up. Avian Pneumovirus Virus (APV). The US was considered free of APV until a virus was recovered from turkeys suffering from an acute rhinotracheitis in Colorado in 1997 by the National Veterinary Services Laboratory. Subsequently, the virus was reported in turkeys in Minnesota and, more recently, in neighboring states. APV infections are associated with a marked increase (up to 25 %) mortality rates on turkey farms as well as increased condemnation of carcasses at processing plants, resulting in losses totaling more than $15 million per year in Minnesota. APV (originally called turkey rhinotracheitis virus) belongs to the Paramyxoviridae family of viruses, and has a single-stranded non-segmented positive sense RNA genome of 13,000-15,000 nucleotides comprising of 8 genes (Randhawa et al., 1997). Although genetic analysis studies shows that the US isolates are distinct from other avian pneumoviruses, there is very little information on the prevalence of APV in the US and its relationship to other previously characterized pneumoviruses. Similarly, knowledge of the mechanism of virus evolution and extent of genetic diversity (presence of different viral strains) is incomplete. Due to the non-pathognomonic nature of the clinical signs of the syndrome, it is important to identify the viral agent in order to make a diagnosis. There are two methods for detecting the virus in affected flocks: virus isolation or DNA-based (Reverse transcriptase-polymerase chain reaction (RT-PCR)) diagnostics. Virus isolation has a relatively poor sensitivity, is time-consuming and is often difficult to perform. This situation is further complicated with the relatively rapid disappearance (within 3 days) of virus following onset of clinical signs in turkeys (Cook, et al., 1993; Jones, 1996). Hence, polymerase chain reaction (PCR)-based approaches are the methods of choice for the rapid detection of APV infections in infected birds. In addition, a serological test (ELISA) has been developed that effectively detects APV antibodies in infected flocks. Previous experience with APV (TRTV) in Europe, Africa, and Asia and experimental studies suggested that wild birds may play a role in the transmission of avian pneumoviruses. For instance, guinea fowl have been shown to possibly harbor TRTV particles in their respiratory tracts (Picault et al., 1987), and both guinea fowl and pheasants seroconvert upon intranasal inoculation of TRTV (Gough et al., 1988). Whereas recent studies in the US also support the role of wild birds in disease spread, including isolation of APV RNA and infectious virus from these birds, the mechanism of transmission, natural hosts, and potential vectors of APV remain unknown. Bordetella avium causes bordetellosis, a highly contagious upper-respiratory disease in various avian species but is most lethal for turkeys (Jackwood et al., 2003). Clinical signs of the disease include an oculo-nasal discharge, rhinitis, tracheal collapse and dyspnea (Jackwood et al., 2003). Additionally, impaired growth, immunosuppression, and increased susceptibility to secondary disease agents contribute to the negative impact on the industry. The bacteria are motile, strictly aerobic, and grow easily on various media including MacConkey, trypticase soy blood agar, and brain heart infusion (Skeeles et al., 1998). Commercial vaccines include a live temperature sensitive (ts) mutant and a whole cell bacterin which have been used by the industry with mixed results (Hofstad et al., 1985, Jackwood et al., 1985). Treatment regimes have included antibiotics (Kelly et al., 1986; Skeeles et al., 1983; Van Alstine et al., 1985), niacin (Yersin et al., 1991), and chemical therapies (Pardue et al., 1998). Current field management strategies have centered on chlorination of the drinking water to reduce bacterial numbers and heightened biosecurity to limit bird exposure. Although these strategies are important, there is clearly a need in the industry for other control measures and efficacious vaccines. Additional research would help to improve the current understanding of the aspects of tracheal colonization, the specific role of toxins, host susceptibility, and the basis of immunosuppression in turkeys. Escherichia coli. Colibacillosis is one of the three most significant infectious diseases affecting turkeys, layers, and broilers (Gross, 1984; Gross, 1994; Gyles, 1993). It occurs most commonly in 5- to 12-week old broiler chickens, but it also occurs in newly hatched chicks and turkey poults (Gross, 1984). Recent outbreaks in laying hens demonstrate that it can be a devastating problem in older birds, as well (Wallner-Pendleton et al., 1999). Collectively, these diseases result in annual multimillion dollar losses by the poultry industry. Despite the importance of colibacillosis in poultry, little is known about the mechanisms used by avian E. coli to cause disease. Multiple stations have been working to identify bacterial characteristics that distinguish virulent avian E. coli from commensals and to better define the interaction between host and pathogen in an effort to identify targets for future control programs. Infectious Bronchitis Virus (IBV). IBV, a coronavirus, causes an acute highly contagious disease of the chicken. IB is the most common cause of respiratory disease of commercial chickens in the US. IB in poultry other than the chicken has also been reported (Cavanaugh et al., 2002; Guy, 2000) but the role of turkeys and pheasants in the disease as it affects chickens is not clear. Control of IB is complicated by the existence of many viral serotypes found in different geographic regions of the USA as well as other countries. Evolution of IBV continues to result in new variant serotypes (Gelb et al., 1991) and has practical significance for controlling the disease because immunity following infection or vaccination with one serotype often is not protective against subsequent infections with unrelated serotypes (Gelb and Jackwood, 1998). IBV strains cause three forms of disease; respiratory disease in young chickens leading to airsacculitis, mortality and condemnation at slaughter; reproductive disease in hens characterized by reduced egg production and quality; and renal disease resulting in mortality (Cavanagh and Naqi, 1997). The virus enters the chicken by inhalation or ingestion and initially replicates in the upper respiratory tract and trachea, the primary target organ and is then disseminated to enteric tissues, such as proventriculus, intestine and cecal tonsil (Ambali and Jones, 1990; Gelb and Jackwood, 1998), as well as kidney (Cumming, 1969), and oviduct (Crinion and Hofstad, 1972). Infection of the lymphoid tissue-containing cecal tonsil in the intestine commonly results in a persistent infection lasting weeks or months after the acute disease episode (Cook, 1968; Alexander and Gough, 1977). Vaccination for IBV uses modified live or inactivated preparations. Live vaccines are generally derived from field strains that are attenuated by egg passage or heat treatment. Live vaccines often contain one or two IBV strains/serotypes and a lentogenic strain of NDV. IBV has been reported to interfere with NDV replication and the development of immunity (Ragi and Lee, 1963, Gelb et al., 1996, Winterfield, 1984). Inactivated IBV oil emulsion vaccines are used as boosters only in breeder and layer chickens because of their high cost and their ineffectiveness as primary immunogens in broilers. The use of attenuated vaccines poses a concern of introducing the virus into a flock and provides the potential of emerging new IBV strains by either point mutation or recombination. Recombinant DNA vaccines potentially provide a safer and more efficient way of inducing immunity against IB. Infectious Bursal Disease Virus (IBDV). Infectious bursal disease (IBD), caused by the birnavirus, infectious bursal disease virus (IBDV), is characterized by destruction of the lymphoid cells in the bursa of Fabricius (BF). Severe B cell suppression (Kibenge, et al., 1988) leads to immunosuppression resulting in exacerbation of host susceptibility to other disease agents, as well as reduction of the chickens ability to respond to other vaccines (McFerran et al., 1980). There are two distinct serotypes of IBDV. Serotype I IBDV are pathogenic to chickens, whereas serotype II viruses, mostly isolated from turkeys, are avirulent for chickens (Ismail et al., 1988). Antigenic variant strains that break through immunity provided by vaccination have been isolated (Rosenberger and Cloud, 1986; Snyder et al., 1988; Ismail and Saif, 1991). Detection and differentiation of IBDV strains is accomplished using monoclonal antibodies and PCR (Wu et al., 1992; Lin et al., 1994; Jackwood and Jackwood, 1994). In recent years, very virulent IBDV (vvIBDV) producing >70% mortality has appeared in several countries (van den Berg et al., 2000). In 2001, vvIBDV was detected in Dominican Republic, the closest country yet to the US. Great concern is that vvIBDV may soon spread to commercial poultry in the US. The genome of IBDV consists of two segments of double-stranded RNA. The smaller segment B encodes VP1, a 97-kDa multifunctional protein with polymerase activity (Kibenge et al., 1988). The larger segment A encodes a 110-kDa-precursor protein in a single large open reading frame (ORF), which is processed into mature VP2, VP3 and VP4 proteins (Hudson, et al., 1986). VP2 and VP3 are the major structural proteins of the virion, whereas VP4 is a minor protein involved in the processing of the precursor protein (Azad et al., 1987; Jagadish et al., 1988). VP2 is the major host-protective immunogen of IBDV and contains the antigenic region responsible for induction of neutralizing antibody and antigenic variation (Bayliss et al., 1990; Heine et al., 1991; Vakharia et al., 1994). VP3, as a group specific antigen, forms a complex with VP1, which may have an essential role for the morphogenesis of IBDV particles (Lombardo et al., 1999). Segment A also encodes a 17-kDa nonstructural VP5 protein from a small ORF, which is found in infected cells (Mundt et al., 1995). This protein is not essential viral replication and VP5-deficient mutant IBDV given to chickens did not induce bursal lesions (Yao et al., 1998). Besides recombinant subunit vaccines, other strategies to combat IBDV infection, including ribozyme (Akin et al., 1999), DNA vaccines (Chang et al., 2002, 2003), and reverse genetics (Mundt et al., 2003) approach are being developed. Infectious Laryngotracheitis Virus (ILTV). ILT, caused by alphaherpesvirus ILTV, is a disease of the repiratory tract often resulting in severe production losses in chickens. Field isolates may also be of relatively low pathogenicity causing milder signs resembling those of infectious bronchitis and lentogenic ND. Attenuated vaccines are used to contol ILT but not on a continuous basis. Chicken embryo origin derived vaccines are at times associated with risks such as insufficient attenuation, spread of the vaccine to nonvaccinates and production of latently infected carriers. More recently, recombinant vaccines have been developed. The orgin of field strains is not always known, although attenuated vaccines have been implicated in some outbreaks. Because the diphtheritic form of fowlpox causes respiratory signs similar to ILT, differentiation of fowlpox from ILT is important. Mycoplasma. Mycoplasma gallisepticum (MG) infections produce respiratory disease in chickens and turkeys. Severe disease losses occur MG infections are complicated by other viral and bacterial agents. Likewise Mycoplasma synoviae (MS) can produce synovitis and when complicated by avian viral infections, airsacculitis can occur in commercial poultry operations. MG and MS were eradicated from commercial broiler chicken and turkey flocks in the U.S. but both MG and MS have reemerged in recent years. In 1999 an MG outbreak occurred in North Carolina involving 54 broiler and turkey breeders, broiler chicken and growing turkey flocks (Vallancourt et al., 2000). In the Delmarva broiler production region the first MG outbreak in over 22 years was recorded (Dohms, 2000). In the same region, a one million-bird egg layer complex broke with MG (Dohms, 2000). More recently, MG infected eggs from a North Carolina broiler breeder flock were shipped, hatched and grown in Delmarva. This risky practice has long been known to spread MG. Newcastle disease virus (NDV). NDV, also known as avian paramyxovirus (APMV)-1, occurs worldwide in commercial poultry as well as many avian species including wild and pet birds. ND can vary from clinically inapparent to highly virulent depending upon virus strain pathotype and host species (Alexander, 1997). In chickens, NDV strains are grouped into three pathotypes based on virulence. Lentogenic strains are the least virulent pathotype and cause mild respiratory disease with low mortality in chickens. Lentogenic strains (e.g. B1 and LaSota) are routinely used as live vaccines in the US. Mesogenic strains of intermediate virulence cause high mortality in young chicks, but not in older chickens. Velogenic strains may be neurotropic (VNNDV) or viscerotropic (VVNDV). Both produce high mortality in all ages of chickens. Pigeon paramyxoviruses in the US have some characteristics of virulent NDV (Gelb et al., 1987; King, 1996; Kommers et al., 2001) and thus potentially may cause problems in commercial poultry. Outbreaks of ND continue to occur in commercial poultry in the USA. The vast majority of field isolates are lentogenic strains (King and Seal, 1998). However, NDV isolates of intermediate to high virulence have also been recovered (King, 1996; King and Seal, 1998). These viruses are not normally found in the U.S. and are considered foreign animal diseases (FAD) and are often referred to as Exotic Newcastle Disease (END). Recently, END was introduced into California and several surrounding states causing the destruction of over 5 million birds with a federal control cost of over 180 million dollars. Fortunately, eradication efforts were successfully, but END is endemic in many parts of the world, and reintroduction of the virus is likely. Vaccination for NDV uses primarily live and inactivated vaccines is almost universally used with commercial poultry. Live vaccines, derived from lentogenic strains, are most commonly used and can provide good protection, but may result in vaccine reactions. The killed adjuvanted vaccines can provide good antibody titers, but because of cost and withdrawal times are typically only used as boosters in breeder and layer chickens because of their higher value and longer production life. Recombinant vaccines using fowl pox (Taylor et al., 1990) and herpes virus of turkeys (Morgan et al., 1992) are licensed but are not widely used because of their high cost. Ornithobacterium rhinotracheale causes respiratory disease in domestic chickens (Sprenger et al., 2000, Van Veen et al., 2000) and turkeys (Roepke, et al., 1998) as well as other wild avian species (Amonsin et al., 1997). Clinical signs include facial edema, sinusitis, tracheitis, pericarditis, and exudative pneumonia (Chin et al., 2003). The disease may increase the susceptibility of secondary infections from Escherichia coli, resulting in excessive mortality in layers and increased airsacculitis in turkeys. The organism is a gram-negative, pleomorphic, non-motile rod-shaped bacterium which grows aerobically, microaerobically, or anaerobically (Chin et al., 1998). Isolation of clinical specimens typically occurs from the respiratory tract (trachea, infraorbital sinuses, and lungs) but may also involve other tissues (Back et al., 1998). There is no current commercial vaccine available for use in the United States, although some studies have examined the use of a temperature sensitive mutant (Lopes et al., 2002) and antibiotic therapy is questionable (Van Veen et al., 2000). Due to the small size of the colonies upon culture and frequent contamination during sample collection, masking by other bacterial agents typically distorts identification and may result in faulty diagnosis. The development of additional identification methods for use by diagnostic laboratories and the poultry industry could assist in determining accurate field outbreaks and estimating the risk potential as a pathogen. The understanding of virulence mechanisms and transmission routes would be essential for vaccine development and reduction of losses associated with primary and secondary infections. Pasteurella multocida is the causative agent of fowl cholera (avian cholera, avian pasteurellosis, and avian hemorrhagic septicemia); a contagious disease appearing in acute or chronic forms (Glisson et al., 2003). In poultry, the disease is initiated through colonization of mucosal epithelium, followed by invasion and dissemination throughout the host. Clinical signs include fever, anorexia, ruffled feathers, diarrhea, and mucous discharge from the mouth (Glisson et al., 2003). Chronic forms of the disease typically follow acute episodes or occur after infection with low virulence organisms. The organism is a gram negative, nonmotile, non-spore forming rod and grows aerobically or anaerobically (Glisson et al., 2003). Colony morphology, physiological properties, and methods for isolation and identification have been described in detail (Glisson et al., 2003). Like many bacterial respiratory diseases, the prevention, control and treatment for fowl cholera has had limited success by the industry. Current vaccination programs with either commercial vaccines or autogenous products have not yielded consistent protection for the poultry industry. This is related to many factors such as duration of immunity, serotype specificity of bacterins, and mortality or clinical disease resulting from live-attenuated vaccines (Bierer et al., 1972; Burns et al., 2003; Heddleston et al., 1972). The Foot-and-Mouth Disease (FMD) outbreak in Great Britain in 2001 presented challenges of biocontainment when officials had to find a way to dispose of large numbers of diseased carcasses in a short period of time (Trevelyan et al., 2001). The subsequent outbreak of AI virus in Virginia reinforced the need for a biosecure disposal method for contaminated carcasses. In the past, emergency disposal of infected carcasses, feed, and bedding have been accomplished through the use of open pyre burning or burial (Trevelyan et al., 2001). Both methods, however, have potential environmental and logistical consequences that have prompted agricultural and environmental officials to request an evaluation of the feasibility and safety of on-farm composting. The composting process typically produces high temperatures and is a routinely used method for on-site disposal of carcasses in the poultry industry. However, in the poultry industry it is designed for small numbers of carcasses/animal tissue and there is usually little regard to biosecurity. In order to be better prepared for a catastrophic event in animal agriculture, such as a foreign animal disease, it is imperative that we discover whether composting is a safe and effective tool for disposal of large amounts of contaminated animal carcasses/tissue in the event of a contagious disease outbreak.
Objectives
-
Identify reservoirs of infectious respiratory disease agents in wild birds and poultry.
-
Develop improved diagnostic capabilities including real time PCR as well as other rapid on-farm tests for economically important respiratory diseases.
-
Investigate the pathogenesis and polymicrobial interactions of specific infectious agents associated with poultry respiratory diseases.
-
Develop new prevention and control strategies for poultry respiratory diseases.
-
Methods
Objective 1. Identify reservoirs of infectious respiratory disease agents in wild birds in the United States. AVIAN INFLUENZA VIRUS (AIV). Several laboratories will perform surveillance in commercial and non-commercial poultry, including live bird markets and wild bird populations. Live bird markets in the Northeast are a reservoir of AIV. H7N2 AIV has been endemic in the markets since 1994 and has been associated with four commercial poultry outbreaks, including the costly Virginia outbreak in 2002. Currently, this lineage of AIV has infected several large operations in Connecticut. AIV surveillance will be conducted in the Northeast by the CONNECTICUT Veterinary Medical Diagnostic Laboratory, as part of the ongoing USDA control program for AI. Examination of auction markets in Delaware will be undertaken by DELAWARE in conjunction with the state veterinarian's office. Efforts to examine commercial poultry will include both active and passive surveillance by several states. CONNECTICUT and INDIANA will test for AIV using real-time RT-PCR, serology and virus isolation on diagnostic samples. DELAWARE will test sera from commercial broilers collected at processing. Wild bird studies will continue through collaborations with the SEPRL, Univ. of Georgia, OHIO, Univ. of Alaska and USDA NVSL (Hanson et al., 2003). AIVs collected in surveillance studies will be characterized by hemagglutinin and neuraminidase subtyping and sequence analysis. The SEPRL has agreed to serve as a central repository for isolates and to provide characterization assistance. This collaboration will be for research purposes only, and is not meant to circumvent the normal diagnostic characterization as supervised by NVSL. AVIAN PNEUMOVIRUS (APV). MINNESOTA (Njenga, Halvorson and Nagaraja) and collaborators from Indiana (Wu), Iowa (Trampel), North Dakota (Dyer), Ohio (Saif), South Dakota (Zeman, Graham), and Wisconsin (Schultz-Cherry) will determine the prevalence of APV in commercial turkeys and wild birds in the Midwestern US. Six years after the initial APV outbreak, the disease remains a problem, primarily in Minnesota. Commercial turkey flocks in neighboring states (Indiana, Iowa, Minnesota, North Dakota, Ohio, South Dakota, Wisconsin, and southern Canada) will be tested using nasal turbinate swabs, turbinate tissues, and serum samples. The turbinate tissues and swabs will be tested for APV RNA by RT-PCR and virus isolation, and sera will be assayed for APV antibodies by ELISA. The frequency of APV in wild birds in the Midwest will also be determined. Nasal turbinate tissues and/or swabs and sera will be obtained from captured wild birds from Minnesota, Iowa, Wisconsin, South Dakota, and North Dakota and tested. INFECTIOUS BRONCHITIS VIRUS (IBV). DELAWARE (Gelb) will collaborate with INDIANA (Wu) and CONNECTICUT (Khan) to monitor IBV genotypes in commercial chickens in the Mid-Atlantic, Midwest, and Northeast regions. Novel genotypes are known to arise in layers raised in multi-age flock management systems. These genotypes may spread to other layers and broilers. INFECTIOUS BURSAL DISEASE VIRUS (IBDV). OHIO (Jackwood). Reservoirs (specify species to be tested) for the re-emerging IBDV have not been identified. The virus is endemic throughout the world. Determining if a reservoir exists for this virus could spawn new options for the control of this immune suppressive disease. Real-time RT-PCR will be conducted using procedures previously developed in our laboratory (Jackwood and Sommer, 2002). An RNA amplification kit for hybridization probes will be used. The primers will amplify a 743 bp sequence region of the VP2 gene (Jackwood et al. 1997). NEWCASTLE DISEASE VIRUS (NDV; Avian Paramyxovirus-1). Surveillance for Newcastle disease will be an emphasis from APHIS for FY2004 and will include many states involved with this program. Specific plans to assess the prevalence of avian PMV-1 infection in wild pigeons by testing sera collected between 1999-2002 for antibodies will be conducted by ALABAMA at Auburn University. ORNITHOBACTERIUM RHINOTRACHEALE. USDA, ARS, Ames, Iowa will conduct sampling from commercial egg-laying complexes and turkey growing operations in the Mid-west or from diagnostic laboratories (Iowa, Minnesota, Missouri, Ohio). Samples will include tracheal swabs and blood for bacterial isolation and serology, respectively. Production data (mortality, body weight, feed conversion, egg weight, egg production, and condemnations) from sample groups will be reviewed. Objective 2. Develop improved diagnostic capabilities including real time PCR as well as other rapid on farm tests for economically important respiratory diseases. AVIAN INFLUENZA VIRUS (AIV). The first generation real-time RT-PCR (RRT-PCR) tests for AIV have been developed and validated for use by the National Animal Health Laboratory network (Spackman et al., 2002; Wise et al., In press; Suarez, et al. 2003). However, continued improvements to the test to achieve greater sensitivity, higher throughput and identify more subtypes remains a goal. Ongoing efforts at the SEPRL and state diagnostic laboratories are to provide additional experience using the RRT-PCR and continue to improve the test to achieve optimal performance. Additional diagnostic tests and reagents will be developed. This includes using a baculovirus expression system and affinity column purification to produce proteins for the HA, NA, and NP subtypes for the development of improved ELISA tests by MARYLAND. DELAWARE in collaboration with SEPRL will develop microarray diagnostics for rapid characterization of AIV. AVIAN PNEUMOVIRUS (APV). MINNESOTA (Njenga, Halvorson and Nagaraja) will collaborate with colleagues from USDA ARS, Georgia (Swayne and Suarez), to develop a standardized real-time PCR test to monitor spread of type specific and subtype C specific APV. Another test to differentiate between A,B,C, and D subtypes will also be developed. E. COLI. IOWA (Nolan). The presence of the increased serum survival gene (iss) may be useful in distinguishing virulent from avirulent strains of avian E. coli (Pfaff-McDonough et al., 2000; Nolan et al., 2003). The protein encoded by this gene, Iss, has been purified, and is currently being used as an immunogen to make monoclonal antibodies (Mabs). These Mabs will be assessed for their abilities to identify virulent strains. FOWLPOX. Illinois and INDIANA will develop ways of differentiating new avianpox virus isolates using virus isolation, adaptation in cell culture and molecular techniques e.g. PCR. INFECTIOUS BURSAL DISEASE VIRUS (IBDV). OHIO (Saif) will produce specific monoclonal antibodies for vvIBDV for the purpose of differentiating them from IBDV of lesser virulence. Viruses used to produce the monoclonals will be inactivated to comply with USDA regulations. In addition, the usefulness of commercial ELISA kits in differentiating between serotype 1 (classic and variant) and 2 IBDV will be examined. Studies indicated that the early commercial kits did not differentiate serotype 1 and 2 viral antibodies. Our studies will use the new generation commercial kits. Real-time RT-PCR, useful for detecting differences in one major neutralizing epitope (hydrophilic region B) (Jackwood and Sommer, 2002), will be expanded to identify sequence differences in other regions of the genome that are known to influence antigenicity, virulence and tropism. MYCOPLASMA. CONNECTICUT (Khan). Multiplex PCR tests for avian mycoplasmas (MG, MS, MI, MM). Delaware (Dohms) will send mycoplasma cultures from field cases to Connecticut for multiplex PCR analysis. ORNITHOBACTERIUM RHINOTRACHEALE. USDA, ARS, Ames (Yersin) will attempt to identify targets for developing PCR-based diagnostic assays. Target sequences will be screened against 16S rRNA gene sequences for other pathogens and normal flora expected to be present in clinical samples. Amplified fragment length polymorphisms will be used to identify targets conserved among isolates. Primers ERIC1R and ERIC2 will be employed for repetitive element sequence-based PCR (REP-PCR). Other methods of discrimination will include restriction endonuclease analysis and ribotyping. Objective 3. Investigate the pathogenesis and polymicrobial interactions of specific infectious agents associated with poultry respiratory diseases. AVIAN INFLUENZA VIRUS (AIV). DELAWARE (Rosenberger and Cloud) will perform pathogenesis studies in chickens and turkeys to determine AIV dissemination and persistence in tissues. They will also assess the impact of immunosuppressive agents on subsequent infections with low path AIV. AVIAN PNEUMOVIRUS (APV). OHIO (Saif) will determine the pathogenic potential of the antigenically related human metapneumovirus for turkeys and chickens. Experimentally infected turkeys and chickens will be observed for clinical signs and examined for lesions. Ohio will also evaluate APVs immunosuppressive potential in avian species. In vivo and in vitro immunologic tests will be conducted on turkeys inoculated with APV to assess the immunosuppressive potential of the virus. BORDETELLA AVIUM. USDA ARS, Ames (Yersin) will evaluate mechanisms of virulence during colonization by challenging poults using both wild type and mutant organisms during direct challenge (trachea) and performing competitive-infection assays. Iowa will also examine the interaction of heat-labile and heat-stable toxins of B. avium. Toxicity assays using Vero cells and chicken embryo fibroblasts will be compared to T and B cell cultures. Based on the results, and that of the mutant study above, comparisons can be obtained that further define the role of toxins in mechanisms of virulence. E. COLI. DELAWARE (Keeler, Emara, and Dohms) and H. Lillehoj, (USDA, ARS) will evaluate interactions of pathogenic E. coli and macrophage using microarrays. Characteristics such as viability and growth in macrophages are of interest in determining pathogenic determinants. IOWA (Nolan) will compare virulent and avirulent avian E. coli by their possession of a variety of traits in order to develop a profile that can be useful in distinguishing the two groups of organisms. INFECTIOUS BRONCHITIS VIRUS (IBV). TEXAS (Collison) and DELAWARE (Gelb) will evaluate a full-length RNA molecular clone of the IBV Beaudette strain developed using a unique reverse genetics system originally designed by Ralph Baric. The clone was constructed and transfected into cell culture, resulting in viable infectious virus. The strategy of the system is that cDNA fragments representing the entire, exceptionally long genome of the virus have been amplified in E. coli plasmids and excised at precise restriction sites. The restriction sites serve as ligation sites for tethering the fragments together to make a cDNA of the complete IBV genome. RNA is transcribed from this cDNA and then transfected into cells to make viable, infectious virus. The system will be used to identify regions critical for pathotype and identify strategies for vaccine development. INFECTIOUS BURSAL DISEASE VIRUS (IBDV). INDIANA (Wu and Lin) will examine the pathogenesis of IBDV using reverse genetically engineered strains for the purpose of understanding the molecular events and mechanisms by which the virus interacts with bursa of Fabricius. Three-week-old SPF chickens will be orally inoculated with reverse genetically engineered infectious bursal disease viruses (Mundt and Vakharia, 1996), with mutations in VP1, VP5, VP2, VP3, and/or VP4. Bursal histopathology, flow cytometric analysis of bursal lymphocyte population, and bursal lymphocyte proliferation assay will be performed. NEWCASTLE DISEASE VIRUS (Avian Paramyxovirus-1). ALABAMA (Toro, van Santen, Roberts, Hoerr, and Dykstra) will determine the pathogenicity of avian PMV-1 isolates obtained recently from diseased feral pigeons for chickens. Endemic immunosuppressive pathogens chicken anemia virus (CAV) (van Santen et al. 2001.) and (IBDV, known to act synergistically with other infectious agents to cause and /or increase the severity of disease, will be included in the pathogenicity studies of the avian (pigeon) PMV-1 isolates. DELAWARE (Gelb and Ladman) will assess potential replication interference of NDV and IBV vaccine strains following simultaneous application. Quantitative RT-PCR will be used to measure the amount of each virus (genome) present in chicken trachea and the embryonated chicken egg. Effects on immunity in chickens will be evaluated. Objective 4. Develop new prevention and control strategies for poultry respiratory diseases. AVIAN INFLUENZA VIRUS (AIV). DELAWARE (Rosenberger and Cloud) will evaluate the efficacy of AIV recombinant and inactivated vaccines for chickens and turkeys. SEPRL will develop improved AIV vaccines (DNA, viral vectored, and DIVA vaccines). AVIAN PNEUMOVIRUS (APV). MINNESOTA (Njenga, Halvorson and Nagaraja) will continue developing live attenuated APV vaccines. Presently, APV is controlled through biosecurity, utilization of a commercially available killed vaccine of questionable efficacy, and a newly licensed high passage attenuated APV (Bioimmune, strain p63). A recently isolated an APV strain from Canada geese, which infected domestic turkeys with no apparent clinical symptoms but confers adequate protection in most birds will be compared to the commercial vaccines in efficacy trials. INFECTIOUS BRONCHITIS VIRUS (IBV). CONNECTICUT will continue evaluating recombinant plasmid DNAs containing S1 subunit and S genes of IBV as vaccines. The DNA vaccines have been shown to be effective against IBV challenge studies in six-week-old chickens. Additional studies are planned to determine the efficacy of the vaccines when given in ovo. The plasmid DNA (S1 and S genes) vaccines will be administered into the albumin on the 14th day of embryonation. Chicks will be challenged with the live pathogenic IB virus at seven days of age to assess their immune response. MARYLAND (Samal) and DELAWARE (Gelb) will evaluate the efficacy of a recombinant (r) NDV containing the S1 gene of the Massachusetts strain of IBV for chickens. The rNDV/IBV will be prepared using procedures developed in Samals laboratory (Krishnamurthy et al., 2000) using the lentogenic LaSota strain, commonly used as a NDV vaccine. Immunity will be assessed by immunizing different groups of chickens with rNDV/IBV S1, rNDV alone, and a commercial LaSota vaccine and challenging the birds with Massachusetts 41 IBV and Texas GB, a neurotropic velogenic strain of NDV. TEXAS (El-Attrache) will analyze CpG-motif oligonucleotides (ODN) as potential poultry immunostimulatory agents. Initially we will compare and evaluate chicken specific ELISA antibody responses to BSA following different routes of administration (oral, spray and subcutaneous) with and without CpG-motif ODN. Later studies will evaluate immune responses following set repetitive vaccination schedules with a killed ND and IB vaccine with and without CpG-motif ODN. Lastly the effect immunizing with IBV and CpG-motif ODN following homologous and heterologous IBV challenge will be determined. INFECTIOUS BURSAL DISEASE VIRUS (IBDV). DELAWARE (Rosenberger and Cloud) will collect IBDV field isolates from various regions of the US and characterize them by VP2 sequencing, monoclonal antibodies, virus neurtalization and cross-challenge studies in chickens. INDIANA (Wu and Lin) and MARYLAND (Vakharia) will examine the pathogenesis of IBDV using reverse genetically engineered strains for the purpose of understanding the molecular events and mechanisms by which the virus interacts with bursa of Fabricius. INDIANA (Wu and Lin) will evaluate DNA vaccination for IBDV (Wolff et al. 1990). DNA vaccines for IBDV are attractive because reverted virulence and environmental contamination can be minimized or avoided. However, in order to achieve 80% to 100% efficacy of protection in specific-pathogen-free (SPF) chickens against infectious bursal disease, at least three times of injection are required (Chang et al., 2002; Chang et al., 2003). Chicken cytokine has been shown to enhance vaccination against infectious diseases in chickens (Karaca et al., 1998). Thus, fewer times of injection and less doses of plasmid DNA co-administered with chicken cytokines may enhance protective immunity and protection efficacy against infectious bursal disease virus infection. Specific-pathogen-free chickens will intramuscularly injected with a chimeric DNA plasmid (carrying VP2 gene of IBDV and chicken cytokine gene) or a DNA plasmid carrying VP2 gene of IBDV alone (Chang et al., 2002; Chang et al., 2003) that will be co-administered with a DNA plasmid carrying chicken cytokine gene. Chickens vaccinated with the plasmid DNA vaccines will be challenged with homologous or heterologous IBDV. ELISA and virus neutralization tests as well as bursal pathology will be performed to determine the immune response and protective efficacy. OHIO (Jackwood) will examine the efficacy of autogenous vaccines for the control of antigenically unique wild-type IBDV by monitoring flocks with real-time RT PCR for viruses that have genetic identity with the autogenous vaccine being used. It has been speculated that quasispecies are the reason for observed cross-neutralization between some classic and variant IBDV strains. We will continue our studies on quasispecies of IBDV and how they are affected by passage in eggs, cell culture and chickens. INFECTIOUS LARYNGOTRACHEITIS VIRUS (ILTV). ILLINOIS (Tripathy) and DELAWARE (Keeler) will continue to evaluate a genetically modified ILTV vaccine. MYCOPLASMA. DELAWARE (Dohms and Keeler) and MINNESOTA (Kapur) will continue sequencing the genome of Mycoplasma synoviae (MS). The information will be used to construct a microarray chip for assessing gene expression of MS to develop improved control strategies. NEWCASTLE DISEASE VIRUS (NDV). ALABAMA (Giambrone and Wu) and UTAH (Frame) in collaboration with B. Nielsen (Brigham Young Univ.) will evaluate a transgenic edible plant vaccine against NDV. Specifically, they will develop a plant-specific expression construct containing the F (fusion) and Hemagglutinin-Neuraminidase (HN) protein genes; transform alfalfa for expression of recombinant subunit viral proteins, and determine the oral immunogenicity and immunoprotection of recombinant NDV proteins expressed in alfalfa. DELAWARE (Rosenberger, Cloud, Gelb, and Ladman) will evaluate the efficacy of NDV live and inactivated vaccines for chickens and turkeys using a velogenic NDV challenge. SEPRL will develop improved NDV DNA and viral vectored vaccines. EPIDEMIOLOGIC CASE STUDY. MARYLAND (Tablante) will perform an epidemiologic study of poultry on the Delmarva peninsula to determine risk factors contributing to respiratory disease for the purpose of developing better prevention and control strategies. The location of commercial and backyard poultry flocks will be identified and plotted on a Geographic Information System (GIS) database. Data on biosecurity and management practices will be collected during a farm visit that will include interviewing the owner or manager using a custom-designed questionnaire for backyard flocks and commercial farms. A case-control study comparing biosecurity and management practices on farms with flocks that test positive for any respiratory disease (through serology and/or virus isolation) compared to normal (respiratory disease-free) farms will be conducted to determine the risk factors that predispose flocks to respiratory disease. Data will be entered into a statistical database and analyzed using descriptive statistics and multivariate logistic regression. A program to prevent and control respiratory disease will be developed based on risk factors found to be statistically significant. The program will be supported by an educational program focusing on biosecurity and flock health management. CONTROL. CARCASS DISPOSAL BY COMPOSTING. IOWA (Reynolds) and MARYLAND (Tablante) will work with other stations in assessing biosecurity related to carcass disposal by composting. Experimental protocols to evaluate the ability of composting to inactivate poultry pathogens will be developed and employed. Measures to assess the biocontainment of composting infected carcasses will be implemented. It is anticipated that these assessment methods will be conducted on composting methods that are conducted in open areas and perhaps will also be used to assess the in-house methods similar to the one evaluated by Tablante et al (2003).Measurement of Progress and Results
Outputs
- Collect and maintain new isolates of respiratory disease agents for research.
- Characterize emerging disease agents in terms of their pathogenicity, antigenicity and genetic composition.
- Identify candidate microbial genes and antigens associated with the immune response.
- Develop, antisera, antigens, cloned genes, and primer sets for diagnostic test applications such as ELISA, PCR, and microarrays.
- Develop disease control strategies including vaccines and specific biosecurity measures.
- Disseminate information through refereed publications. Sponsor symposia, workshops, and informal discussions at national and international meetings. Continue to use our web site for dissemination of new information. http://www.wisc.edu/ncra/nc228index.htm
Outcomes or Projected Impacts
- Research will produce greater understanding of disease reservoirs, processes and control interventions.
- Reduced mortality and condemnation will result through use of improved vaccines and biosecurity measures.
- Consumers will enjoy safe, healthy, and competitively priced eggs and poultry meat products.