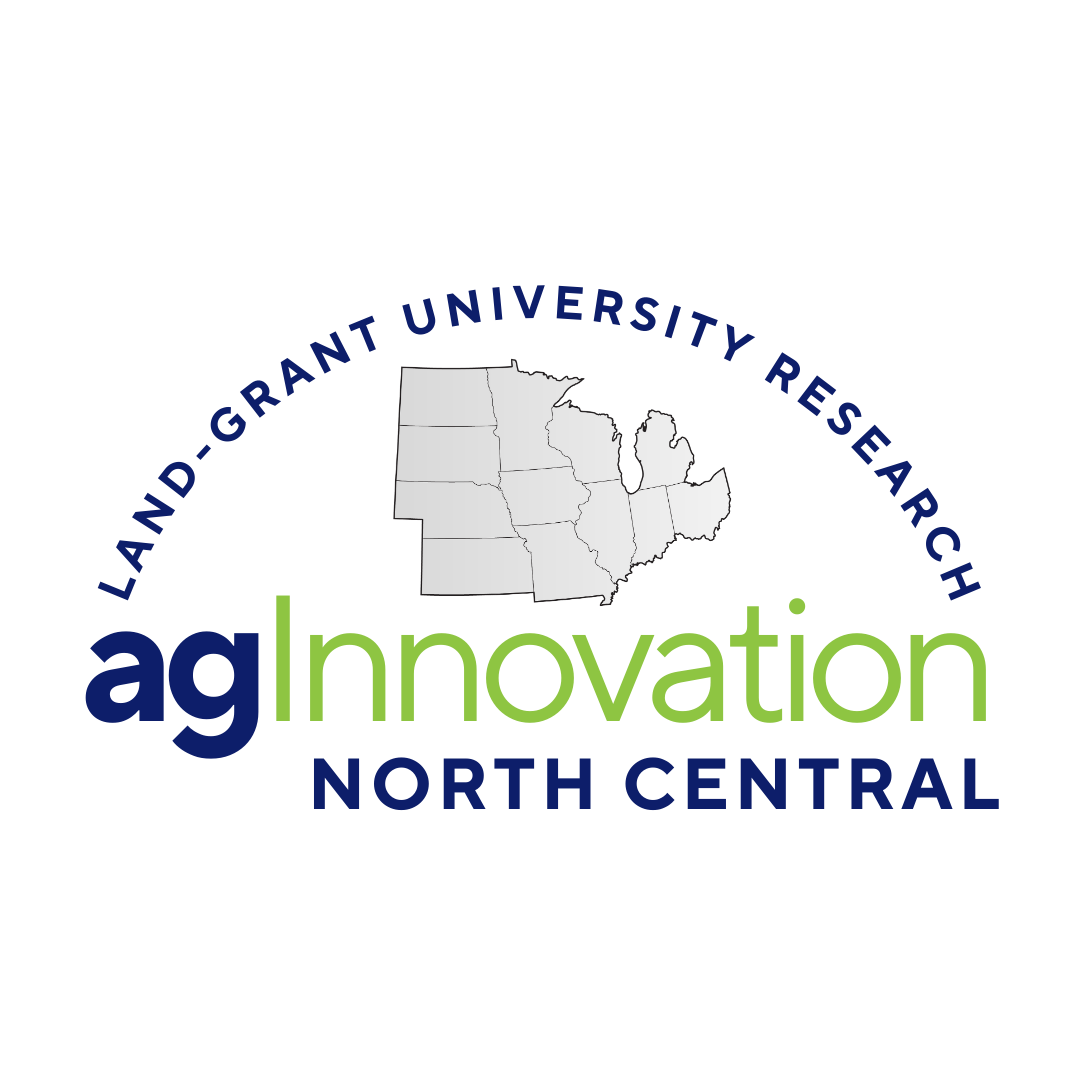
NC_temp1082: Biological Control of Plant Pathogens in the North Central Region (NC125)
(Multistate Research Project)
Status: Inactive/Terminating
NC_temp1082: Biological Control of Plant Pathogens in the North Central Region (NC125)
Duration: 10/01/2004 to 09/30/2005
Administrative Advisor(s):
NIFA Reps:
Non-Technical Summary
Statement of Issues and Justification
Plant pathogens cause diseases that dramatically impact the yield and quality of crops produced in the North Central region. Management of these diseases is limited due to a variety of environmental and economic constraints. Effective biological control strategies can provide useful options for integrated pest management. This project addresses the interactions of applied and resident microorganisms with plant pathogens and their host plants within the varied environments of the region. The ultimate objective is to develop biologically-based disease management strategies that maximize the benefits of applied and resident microbial agents.
Pathogens that cause the most significant diseases on agronomic and horticultural crops are widely distributed across the North Central region. These pathogens are phylogenetically diverse (e.g. various prokaryotes, oomyctes, fungi, and nematodes) and predominantly opportunistic, attacking plants when conditions are conducive to disease development (Agrios, 1997). While the spectrum of destructive diseases varies annually, important genera of pathogens (e.g. Bipolaris, Colletotrichum, Fusarium, Heterodera, Phialophora, Puccinia, Pythium, Phytophthora, Rhizoctonia, and Sclerotinia) are endemic to the North Central region. There, and throughout the United States, these pathogens present a perennial problem that has to be managed properly for optimum productivity. In large fields, multiple diseases are often present, and this complex of diseases can result in substantial yield losses. For example, a recent study by Nutter et al. (2002) showed that the average yield loss in alfalfa due to foliar diseases could exceed 19% and that over 50% of the variance in yields could be attributed to plant diseases. In the North Central region, the yield losses caused by these plant pathogens is estimated to be worth several billion dollars, despite the current disease control efforts. For example, soybean farmers in the North Central region lose approximately 6.8 million tons each year in yield (valued at $1.475 billion) to soilborne plant diseases alone (Wrather et al., 2003). Effective disease management strategies are needed to minimize the economic damage to the major field crops of the North Central region.
The destructive activities of soilborne and residue-borne pathogens can be limited by a variety of farming practices, including crop rotation, tillage, and/or seed-treatment fungicides (Hoeft, 2000). These practices reduce disease by removing the host (rotation), damaging or displacing the pathogen (tillage), and avoiding the optimum growth conditions of the pathogen (based on planting date or planting density). Fundamentally, the management of crop residues and organic matter plays a key role in the control of root diseases (Bockus, 1998; Hoitink, 1999). Even so, a number of environmental variables, including climate and edaphic factors, can impact the rotation effect (Porter, 1997). While many root pathogens are generally crop-specific (e.g. for either corn or soybeans), some soilborne pathogens are virulent on multiple crops (e.g. Zhang and Yang, 2000). Chemical fungicides (e.g. mefonoxam and fludioxonil) can be used to suppress pathogen activity in the seedbed (Hoeft, 2000). Although seed treatment fungicides are used in the vast majority of corn and wheat plantings and are generally effective at promoting seedling emergence and vigor (especially in corn), chemical seed treatments do not provide season-long protection from soilborne pathogens and, therefore, yield responses are not consistently observed with their application (Lipps, 2001). Applications of chemical fungicides in urban landscapes (e.g. on golf courses and lawns) can be substantial but elevate concerns about negative impacts on water quality and human health (Vargas, 1994). Successful application of biological control organisms requires more knowledge-intensive management (BCWG, 1998), including a greater understanding of the environmental and economic issues influencing effective biopesticide use in a variety of agricultural systems.
Biological control agents, i.e. organisms antagonistic to plant pests and pathogens, can be used to reduce the incidence and severity of root diseases (Handelsman and Stabb, 1996; Cook and Baker, 1983), and foliar diseases (gb refs ). Phylogenetically diverse microorganisms have been used as biocontrol agents (EPA, 2003), but only a small fraction of isolates from named genera have significant biocontrol activity. The mechanisms by which these microbial agents suppress diseases include antibiosis, parasitism, competition, and induction of host resistance (Cook and Baker, 1983). Some agents may use multiple mechanisms to suppress disease. For example, Lysobacter enzymogenes has been shown to secrete chitinases, which may degrade fungal cell walls and subsequently induce plant host defenses (Kilic-Ekici, 2003; Zhang and Yuen, 2000). While sometimes perceived as less reliable or less effective than chemical alternatives, several microbial biocontrol agents have proven useful in multiple applications (Harman, 2000). This success is reflected in the fact that over two dozen biocontrol products are currently being marketed to producers (McSpadden Gardener and Fravel, 2002). Numerous studies have shown that biological controls can be used alone or in combination with chemicals to successfully increase stands and/or yields of a diversity of crops. However, many years of research have shown that microbial biological control agents do not perform consistently under all field conditions. The causes of inconsistent performance of biocontrol agents are not well understood, but likely are rooted in the complex interactions between microorganisms, plant hosts, and the abiotic environment that lead to biological control.
In conventional agriculture, trends toward decreasing the frequency and types of rotations, reducing tillage, and reducing pesticide inputs all increase the potential for crop losses to plant diseases. Indeed, long-term research plots in the Midwest indicate that the omission of either tillage or rotation can reduce corn yields by 8% and soybean yields by up to 20% (Crookston et al., 1991; Hickman and Medlin, 2001, Porter et al., 1997). In addition, organic farmers need environmentally-friendly and socially-acceptable disease control practices. In all types of systems, augmentative biological control strategies hold promise for improving production efficiency. Indeed, biological controls of plant pests and pathogens have been targeted for development as part of integrated pest management (IPM) programs, and they are expected to play a key role in sustainable agriculture in the future (Jacobsen 1997). Microbial biological control agents are generally understood to be less toxic and, potentially, less costly than chemical fungicides, thus these agents can play a major role in the development of sustainable agricultural systems. There is a growing demand for sound, biologically-based pest management practices. Recent surveys of both conventional and organic growers indicate an interest in using biocontrol products (van Arsdall and Frantz, 2001; Rzewnicki, 2000), suggesting that the market potential for biocontrol products will increase in coming years.
Continued prospecting for new biological control agents is required to diversify the potential applications of biocontrol, identify more effective agents, and replace commercialized biocontrol products should resistance develop. The successful identification of candidate biocontrol agents requires effective screening procedures. Previous screening programs have yielded numerous candidate organisms for commercial development (Cook and Baker, 1983). The success of all subsequent stages of developing biocontrol strategies depends on the ability of a screening procedure to identify appropriate candidate agents. Conventional screens for new biocontrol microbes are still useful (e.g. Bull et al., 2002, Michaud et al. 2002); however, new techniques can enhance our ability to screen for effective biocontrol agents. For example, application of molecular markers for biocontrol activities will facilitate screening for locally adapted biocontrol strains (Landa et al., 2002, Raaijmakers and Weller, 2001).
Funding for basic and applied research continues to be provided by the USDA's Cooperative Research, Education, and Extension Service through a variety of programs (eg. the National Research Initiative (NRI), and the Pest Management Alternatives Program (PMAP)) as well as internally within the Agricultural Research Service. Such investment will ensure that innovations in biological control research will continue. An upswing in commercial interests has also developed in the past few years, and prospects for increased growth are positive. The Biopesticide Industry Alliance has formed, and it is now actively promoting the value and efficacy of biopesticides, including those that control plant pathogens. Clearly, the future success of the biological control industry will depend on innovative business management, product marketing, extension education, and research (BCWG, 1998; Mathre et al., 1999).
A variety of research questions remain to be answered about the nature of biological control and the means to most effectively manage it under production conditions. Advanced molecular techniques are now being used to characterize the diversity, abundance, and activities of microbes that live in and around plants, including those that significantly impact plant health. Such investigations include the characterization of the activities of biocontrol agents (Zhou, 2002; Kobayashi, 2002). Much remains to be learned about the microbial ecology of plant associated microorganisms in different agricultural systems (Weller et al., 2002). Recent advances in this area have led to a greater understanding of colonization of both roots and shoots of crop plants (Beattie and Marcell, 2002; McSpadden Gardener, 2002). Fundamental work is needed on characterizing the mechanisms by which organic amendments reduce plant disease (Nelson and Boehm, 2002). Studies are needed on the practical aspects of integrating biocontrol strategies with other farm practices (e.g. Dodds, 2002; Windels, 2002) and on how to make new biocontrol products stable, effective, safer and more cost-effective. Ecological models can be applied to identify the required characteristics of biocontrol agents and the stages in pathogen life cycles at which they are most readily managed via biological control (e.g., Garrett and Bowden, 2002). To better evaluate the costs and benefits of using biological control strategies, plant disease and associated biocontrol sub-models, akin to those developed for crop physiology (Gage, 2002), need to be developed. Computer models may be useful in determining the severity and occurrence of various plant diseases and the utility of various biocontrol strategies at expanded scales over large time frames (Safir, 2002). A greater understanding of the ecology, physiology and genetic variation of biocontrol agents, their methods of application, and their interactions with pathogens, hosts, and the environment is necessary before there is widespread commercial-scale use of biocontrol in U.S. agriculture.
Regional cooperation among scientists working on biocontrol can advance knowledge on how to use this important technology. Sharing of biocontrol agents and testing on various crops under a wide range of environments and soil types is one type of cooperation. Another is the development of techniques for producing, storing, and delivering biocontrol agents. The standardization of protocols for testing is an important result of cooperation. Regional cooperation also provides important a forum for discussions in which results and ideas can be exchanged and debated and cooperative efforts are enhanced and broadened.
In this five year project, we will build on the past success of cooperative efforts on biocontrol in the North Central Region. Working collaboratively, we will systematically compare the efficacy of biocontrol products on economically important plant species and characterize the mechanisms of biocontrol systems. Research across the North Central region will allow us to characterize the factors that influence the effectiveness of biocontrol systems and develop strategies for enhancing the activity of applied and indigenous biocontrol agents. Through such collaborative research and scientific exchange, we will foster the development of new ideas and approaches for biological control. The results and accomplishments of this project will have direct application to the use of biocontrol to protect economically important plants from a variety of plant pathogens.
Related, Current and Previous Work
The extensive body of literature reflects both the interest and potential of biocontrol in agriculture. Despite the interest, however, biocontrols have failed to be implemented as part of mainstream agricultural practices due to performance variability observed in field situations. Inconsistencies in biocontrol performance are often attributed to influences imposed on biocontrol agents by the plant host and environment. Both factors can vary widely between geographical regions and thus emphasize the need for specific biocontrol research in the North Central region. Approaches to overcome inconsistencies with biocontrols begin with understanding the mechanisms by which agents control disease, as well as understanding the diseases of specific cropping systems. Equally as important is the development of compatible management strategies to enhance biocontrol, both with indigenous microbial agents and introduced biocontrol agents.
NC-125 is comprised of a group of investigators with a broad range of expertise in both applied and basic biocontrol research. During the time span of the current project, the committee has focused on studies directed at disease control efficacy and understanding biocontrol mechanisms. Results from these studies have contributed significantly to the advancement of biological control of plant diseases, especially concerning cropping systems important to the North Central region. Some notable results are highlighted in the sections that follow.
When comparing the efficacy of biocontrol systems on economically important plants in the North Central region, committee members have taken a variety of direct and indirect approaches. These include testing various biocontrol agents for efficacy, developing predictive models for disease, and evaluating the effects of various amendments on cropping systems used in the North Central region. These approaches have proven useful in advancing our understanding of how to better utilize biocontrols and in reducing chemical pesticide inputs for disease control.
The assessment of new uses for biocontrol agents is an important, ongoing effort of the committee. Among the many biocontrols that have been assessed by the committee in the past, a notable example involves Lysobacter enzymogenes (formerly reported as Stenotrophomonas maltophilia) strain C3. Biocontrol efficacy has been reported for strain C3 on numerous diseases affecting a variety of crops, including brown patch, summer patch, stem rust, Bipolaris leaf spot and anthracnose on turfgrasses, Pythium damping off of sugarbeet, Fusarium head blight of wheat, and bean rust of dry bean (Yuen et al., 2003; Yuen et al., 2001; Zhang and Yuen, 1999; Yuen and Zhang, 2001; Kobayashi et al., 2003). Examples of other efforts directed at identifying new biocontrols for regional disease problems include investigations with mycoviruses associated with Fusarium solani f. sp. glycines, causal agent of sudden death syndrome of soybean disease (Marvelli et al, 2001). Similarly, greenhouse screens were developed in North Dakota for numerous root rot diseases of soybean, providing useful procedures for screening potential biocontrol agents and evaluating other management practices to enhance biocontrols.
Several researchers have made advances in developing predictive models as methods to augment and enhance biocontrol efficacy. For example, a simple population model of the Karnal bunt pathogen of wheat was developed in Kansas (Garrett and Bowden, 2002), and it was found to be useful for identifying susceptible stages of the pathogen life cycle that can be targeted for developing effective biocontrol. In Minnesota, a modified Weibull model was used to predict oospore survival and populations of Aphanomyces cochlioides exposed to high temperatures (Dyer and Windels, 2002). In this particular study, oospore mortality increased with increasing temperature. Indeed, by taking advantage of this knowledge, soil solarization was found to decrease Aphanomyces root rot while increasing both stand and yield of sugarbeet (Brantner et al 2002; Windels, et al. 2002). Efforts in Michigan have resulted in the development of a modeling applications systems framework (MASIF) (Gage et al., 2001) that is currently being used to analyze the effects of biocontrols and environmental parameters on crop yield at the county and regional level. Using crop simulation models within the framework, the economic feasibility of using biocontrols can be determined.
Committee members have evaluated the use of various soil amendments as a means to enhance biocontrol. For example, in Minnesota, addition of green manure to soils reduced Verticillium wilt of potato and Phytophthora on alfalfa, and also reduced pathogen survival rate in the soil. Green manure amendments were correlated with increases in antibiotic production within soil microbial communities (Kinkel et al. 2001). In a second example, soil amendments of composted and raw dairy manure in Ohio showed promise in increasing antibiotic-producing fluorescent pseudomonads in the rhizospheres of field-grown tomato and cabbage (McSpadden Gardener et al, 2002). These two examples highlight some common goals of committee members, in which amendments are not only being evaluated for improving regional cropping systems, but also for their effect on beneficial microbial populations.
Other management strategies investigated by committee members have also shown promise as potential nonchemical methods that can be used in combination with biocontrols. As mentioned in the previous section, soil solarization showed significant promise as a practice for improving sugarbeet yield in Minnesota. In Kansas, studies indicated that integrated management practices were important for durability of plant genetic resistance in three different pathosystems (Mundt et al., 2002). Ongoing studies in Indiana have associated uptake of the reduced form of manganese in soil by plants with resistance to disease. Evidence was generated that glyphosate resistance in corn and soybean is linked to a reduction in manganese uptake efficiency in soils, which in turn can detrimentally influence plant resistance to disease (Dodds et al., 2002). In related studies, application of the biocontrol fungus, Trichoderma virens increased corn yield in low manganese soils. The T. virens strain used for this study is a strong manganese reducer, suggesting its presence in the soil facilitated uptake of the element by plants.
Significant research efforts by the committee have been directed at understanding some of the host and environmental influences on biocontrol efficacy and consistency. Two highly relevant examples of such research by committee members involve work conducted in Iowa and New York. Experiments in Iowa have focused on microbial colonization of plant surfaces, which is often a prerequisite for biocontrol. For example, using a series of mutant hosts altered in their leaf surface cuticle, the cuticle layer on plants was shown to significantly influence the amount of bacterial inoculum retained on plant surfaces after application, but to have a relatively minor effect on subsequent bacterial colonization (Beattie and Marcell, 2002; Marcell and Beattie, 2002). Given the importance of water availability to the activity of pathogenic and antagonistic microbes, a sensitive, in situ method was developed in Iowa to quantify the availability of water to microbes in planta and thus to better predict environmental influences on microbial activity (Axtell and Beattie, 2002). In New York, the influence of seed exudation on stimulation of Pythium spore germination was investigated. These studies have demonstrated that certain fatty acids released in host plant exudates function as signals for pathogen spore germination. Metabolism of these fatty acids by microbial agents can effectively block signaling of Pythium spore germination, resulting in prevention of infection and disease (van Dijk and Nelson, 1998; van Dijk and Nelson, 2000). Such activity is dependent upon the rate of exudation from seeds with respect to the stimulation of pathogen spore germination and the effects of other exudates (Kageyama and Nelson, 2003). Strong evidence supported catabolite repression of the biocontrol mechanism as the reason for the failure of biocontrol on some hosts but not on others (Kageyama and Nelson, 2003). The findings from research conducted in Iowa and New York have global significant impact in the field of biocontrol, especially with respect to developing approaches to overcome performance inconsistencies.
Many research programs (e.g., those in Iowa, Nebraska, New Jersey, New York and Ohio) have focused on understanding mechanisms of biocontrol and microbial traits associated with biocontrol efficacy. Information generated from these studies should enhance the efforts of applied biocontrol studies and improve biocontrol consistency. In New York, as an extension of the previous mentioned studies, microbial activity found in conducive composts was compared with that in suppressive composts; suppressive composts supported growth of microbes that both degraded Pythium germination signals and that colonized plant host seeds in high populations. Additional studies indicated that microbial populations arising from soils amended from the different composts differed according to specific metabolic capabilities. This metabolic difference was linked with biocontrol of Pythium damping off (McKellar and Nelson, 2003). An extension of these studies indicated that differences in the quantity and content of carbon sources in seed exudates among plant species were linked to biocontrol efficacy of Enterobacter cloacae.
In Ohio, a rapid PCR-based method was developed to monitor antibiotic-producing Pseudomonas spp. populations in the plant rhizosphere (McSpadden Gardener et al., 2001). Using this method, notable differences in the populations of antibiotic-producing pseudomonads were observed on different crops, at different locations, and under different management regimes (McSpadden Gardener and Lutton, 2002, McSpadden Gardener et al 2002). The relationships between these biocontrol populations and other environmental variables are the subject of ongoing investigations. Such work has revealed how crop and soil type may influence the biogeography and activities of DAPG-producing Pseudomonas strains in Ohio.
Taxonomic characterization was conducted on strain C3, formerly reported as Stenotrophomonas maltophilia, in New Jersey. The relevance of repositioning of strain C3 as Lysobacter enzymogenes (Sullivan et al., 2003) is two-fold: 1) the effective biocontrol strain C3 is removed from taxonomic association with clinical strains (Stenotrophomonas maltophilia); and 2) it establishes a little recognized group of bacteria (Lysobacter spp.) as a potential mining source for biocontrol agents.
A number of studies investigated biocontrol mechanisms at the molecular and biochemical levels. Combined efforts between Nebraska and New Jersey indicate several traits expressed by L. enzymogenes C3 are associated with biocontrol activity. Both induced resistance and antibiosis via a small molecular weight compound were reported as potential biocontrol mechanisms by L. enzymogenes C3 in Nebraska for Bipolaris leaf spot of tall fescue and for Fusarium head blight in wheat (Yuen and Kilic, 2001; Kilic-Ekici and Yuen, 2003; Li et al., 2003). These two newly identified mechanisms expressed by C3 complement other studies on biocontrol mechanisms expressed by L. enzymogenes. To date, three glucanase genes (Palumbo et al.2003), one of at least 2 chitinase genes that encode for at least five isozymes (Zhang et al. 2001; Zhang and Yuen, 2000a; Zhang and Yuen, 2000b; Bick et al., 2001), and a type III secretion system have been identified and molecularly characterized (Reedy and Kobayashi, 2001; 2003). Their roles in biocontrol are currently being determined by mutational analysis. In addition, a global regulatory region that controls extracellular enzyme production, and is associated with fungal antagonism was identified in L. enzymogenes. The region encodes a catabolite-activator-like protein (clp) homologue and a putative acetyltransferase on a single RNA transcript that is differentially regulated based on specific carbon sources (Palumbo et al. 2000; Zhou et al., 2002; Kobayashi et al., 2003). Complete loss of control efficacy of Bipolaris leaf spot of tall fescue and Pythium damping off of sugarbeet was observed with a strain mutated at the locus, indicating the regulatory locus globally controls traits associated with biocontrol in these pathosystems (Palumbo et al., 2000; Kobayashi et al., 2003).
Related Regional Research Projects: The goals of NC-125 and the currently proposed committee share some similarities with those of W-147 and S-302. However, the major distinctions of this proposal include: i) focus on the economically important crops grown in the North Central region, ii) examination of foliar as well as root pathosystems, iii) use of models for defining the parameters required for effective biological control at multiple scales, and iv) characterization and application of a distinct set of biological control agents.
Objectives
-
Evaluate biocontrol strategies for the management of diverse pathogen systems
-
Determine the influences of host, environment, and microbial populations on biocontrol at multiple spatial and temporal scales
-
Elucidate the molecular and cellular basis of traits affecting biological control
-
Methods
Objective 1: Evaluate biocontrol strategies for management of diverse pathogen systems. Experiments involving applied microorganisms will be conducted using legumes such as soybean, common bean, and alfalfa (IA, IL, IN, ND, NE, OH), tomatoes (OH), wheat, and turfgrass (NE), cucumber and cotton (NY). Both soilborne and foliar pathogens will be targeted. These include Fusarium solani, Phytophthora sojae, Pythium spp., Sclerotinia sclerotiorum, and Heterodera glycines in legumes (IL, IN, ND, NE, NY, OH), Phoma medicaginis in alfalfa (IA), Fusarium graminearum in wheat (NE), Rhizoctonia solani in turfgrass (NE), and Colletotrichum coccodes in tomato (OH). Most studies will involve, but not be limited to, experimental strains of bacteria (e.g., Bacillus, Enterobacter, Lysobacter, Pantoea, and Pseudomonas) and fungi (e.g., Gliocladium and Trichoderma) that are non-specific antagonists. Some will involve pathogen-specific mycoparasites (e.g. Sporidesmium sclerotivorum against Sclerotinia [ND] and pyrenomycetous fungi against Fusarium spp. [NE]). For Sporidesmium, methods for mass production of the mycoparasite will be investigated (ND). Biocontrol agents isolated by committee members will be exchanged with other members for initial evaluation in greenhouse model systems. Promising agents will then be tested in field trials in which they will be applied as seed treatments, soil amendments, or foliar sprays. Committee members (IA, IL, IN, ND, NE) will conduct systematic evaluations of commercially produced biocontrol agents on different pathosystems. In addition to applied agents being evaluated on the basis of disease inhibition and growth/yield enhancement, microbial populations on appropriate plant parts will be quantified by cultural and molecular procedures (e.g. PCR) so that colonization ability across crops and diverse environments can be determined. Methodology for measuring populations of specific agents or species will be shared among committee members. Efforts will be made standardize experimental designs for biocontrol agent evaluations, and data will be pooled for joint publication. We will also investigate the enhancement of the biocontrol activity of indigenous microorganisms using soil amendments (IL, MN, NY). The effects of incorporating materials, such as cover crops and composts, on root disease development will be evaluated. Population levels of the pathogens and other soil-microorganisms will be monitored. Soil-soil amendment combinations identified to be pathogen suppressive will be investigated further in the following objectives. Objective 2 . Determine the influences of host, environment, and microbial populations on biocontrol at multiple spatial and temporal scales. Several studies will be conducted to assess the influences of abiotic factors on biocontrol agent activity. Leaf surface water availability will be examined for its role in the growth, survival and behavior of bacterial populations on leaves (IA). Water stress tolerance in Pantoea agglomerans and Pseudomonas syringae will be evaluated using molecular approaches. Measured water availability conditions and water stress tolerance levels will be related to population dynamics of these bacteria on leaves of bean and corn. Analysis of water stress tolerance will be extended as well to other organisms contributed by committee members to evaluate the effects of these factors on leaf colonization. The influence of carbon compound type and quantity occurring in seed and root exudates on control of Pythium damping off by bacterial agents will be investigated in NJ and NY to answer the question as to why biological control is more effective in some soil environments as opposed to others. Metabolic activity of P. fluorescens Pf-5 will be monitored in different speromosphere and rhizosphere environments using the luxAB reporter gene system or using oxygen microelectrodes, and then correlated with expression of select biocontrol traits, as well as biocontrol activity (NJ). Because fatty acid degradation is essential for biological control activity of Enterobacter cloacae and sugars block the process, the qualitative and quantitative fatty acid and sugar profiles from plant species that support different levels of E. cloacae biological control activity and the general timing of fatty acid and sugar release will be studied (NY). The availability of sugars and fatty acids to bacteria in the spermospheres of the various plant species will be monitored using a sucrose biosensor strain of Pantoea agglomerans and an E. cloacae fatty acid biosensor. Research efforts also will focus on characterizing the biotic components that influence soil suppressiveness. Microbial community composition will be investigated by culturing methods (IN, MN, ND, NY, OH) and via collaborative efforts by culture-independent procedures such as DNA sequencing, T-RFLP analyses (OH) and DGGE analyses of amplified DNA (NY), and fluorescent in situ hybridization (FISH) (NY). While community profiles will include soil and rhizosphere bacteria and fungi (OH), particular attention will be paid to seed-colonizing bacteria (NY) and Streptomyces spp. in soil (MN). Specific microbial populations contributing to pathogen suppression will be identified (IN, ND, NE, NJ, OH). In OH, examinations of species and sub-species level diversity of several biocontrol genera (e.g. Pseudomonas and Bacillus) will be conducted. In NE and NJ, strains of Lysobacter enzymogenes from different soil, organic matter, and plant surface environments will compared for ecological (e.g. rhizosphere and phyllosphere competence), physiological, (e.g. lytic enzyme activity, antibiotic production, induction of plant resistance), and genetic (e.g. regulatory systems for extracellular metabolite production and secretion) traits. The relationship of various traits to effectiveness in biological control then will be determined. It is recognized that populations of any given bacterial species in the environments are comprised of cultural cells and viable but non-culturable cells. In IA, the potential contribution of viable but non-culturable bacterial cells to the total population colonizing leaves, and subsequently to biological control, will be determined using P. agglomerans on bean and corn. Further studies will be conducted with P. syringae and P. fluorescens to determine how this factor differs among bacterial species. In IL, a unique group of potential biocontrol agents, dsRNA mycoviruses, will be examined for genetic diversity and evaluated for effects on Fusarium solani f. sp. glycines, the causal agent of sudden death syndrome in soybean. This approach will be extended to mycoviruses in other fungal soybean pathogens (Rhizoctonia, Phialophora, and Sclerotinia) as well. Committee members (IL, IN, MN, NY, OH) will explore field scale factors (i.e. cropping systems, crop rotations, soil amendments, composts and tillage practices) in relations to pathogen suppression by indigenous microoganisms or by specific antagonists, e.g. Streptomyces spp. (MN), Coniothyrium minitans and Sporidesmium sclerotivorum (ND). Crop-pathogen systems to be studied will include those described for objective I. Disease levels, pathogen populations, antagonist communities, and edaphic conditions under each treatment will be analyzed. Finally, a modeling approach will be used to predict the impact of biological control, and efforts will be made to incorporate the results of field studies in objective I. One set of models to be developed in KS will use the life history characteristics of wheat and of its pathogens to determine when and where biological control measures will be effective and the characteristics of successful biological control agents or mixtures. Ecological theory developed in the context of invasive species will be used to model the requirements for dispersal of biological control agents through agroecosystems to effectively manage pathogens. Efforts will be made to link these models to another set to be developed in MI. MI efforts will incorporate existing regional environmental databases and physiological crop models for corn, soybean and wheat developed for field level simulations into a modeling applications framework (MASIF) that will expand field-specific models to multicounty and regional use. By incorporating plant disease and associated biocontrol submodels, the potential occurrence and severity of certain plant diseases and the utility of various biocontrol options can be determined at spatial scales ranging from the field to the region over large time frames. Objective 3: Elucidate the molecular and cellular basis of traits affecting biological control. Characterization of traits influencing biocontrol will continue. Mechanisms expressed by Lysobacter enzymogenes strain C3 will be intensified (NE, NJ). To date, 3 glucanase genes, 1 of at least 2 chitinase genes, a 2-gene global regulatory system consisting of a CAP-like protein (clp) regulator and a putative acetyltransferase locus, and a type III protein secretion system (TTPSS) consisting of at least 11 identified genes suspected to be involved in L. enzymogenes pathogenesis of fungi have been characterized. Strain C3 also induces host resistance in monocot plants and produces a heat stable antifungal factor, or HSA. In NJ, the characterization of extracellular enzymes will continue. In addition, more in depth molecular characterization, including mutational analyses, will be conducted for all genes characterized to date. In NE, HSA will be characterized as to its chemical structure and mode of action, the latter accomplished using a genetic approach with Aspergillus nidulans as the model system. Host genes associated with induced resistance activated by strain C3 will be identified; then using gene activation as markers for induced resistance, the elicitor for induced resistance by C3 will be characterized. The involvement of HSA and induced resistance in biological control by strain C3 will be determined through comparison of mutants of strain C3 deficient in these and other traits with the wild type. Molecular characterization of traits influencing epiphytic colonization of Pseudomonas syringae will be performed in relation to the genes and regulons contributing to environmental stress tolerance (IA). The genetic basis for biocontrol activity of commercialized B. subtilis strains will be investigated (OH). Mechanisms of action will be examined for selected biological control agents after they are identified by committee members as described under objectives 1 and 2.Measurement of Progress and Results
Outputs
- Highly significant advances in the understanding of biological control systems will result from this project. These will include but are not limited to the identification of new biological control strategies, the elucidation of influences of environmental variables (biotic and abiotic) on biocontrol systems, and the characterization of biocontrol mechanisms of select agents.
- Based on the productivity of the current project with 11 active members, we anticipate that we will generate at least 50 research publications, several of which will be of joint authorship among committee.
- Committee members will cooperate in the development of outreach materials. For example, we will develop general information publications on concepts and practices of biological control, which incorporate the outputs of this and previous committees, for the North Central Region. Drafts of these publications will be made available on the American Phytopathological Society Biological Control committees web site to encourage input, and will be submitted for publication in the APSnet Education Center, for a student audience, and the Plant Management Network, for stakeholder audiences (e. g., farmers, crop consultants, horticulturalists, extension advisors).
Outcomes or Projected Impacts
- The advances in understanding of biological control systems will contribute to the development of integrated strategies that will improve the management of diseases that are difficult to control and will reduce dependence on synthetic pesticides.
- We will increase the awareness of stakeholders as to the availability and effectiveness of biorational plant disease control strategies.
- Greater acceptance of biological control by the agricultural community will be achieved through an improved understanding of the molecular, physiological, and ecological bases for biological control of plant pathogens.