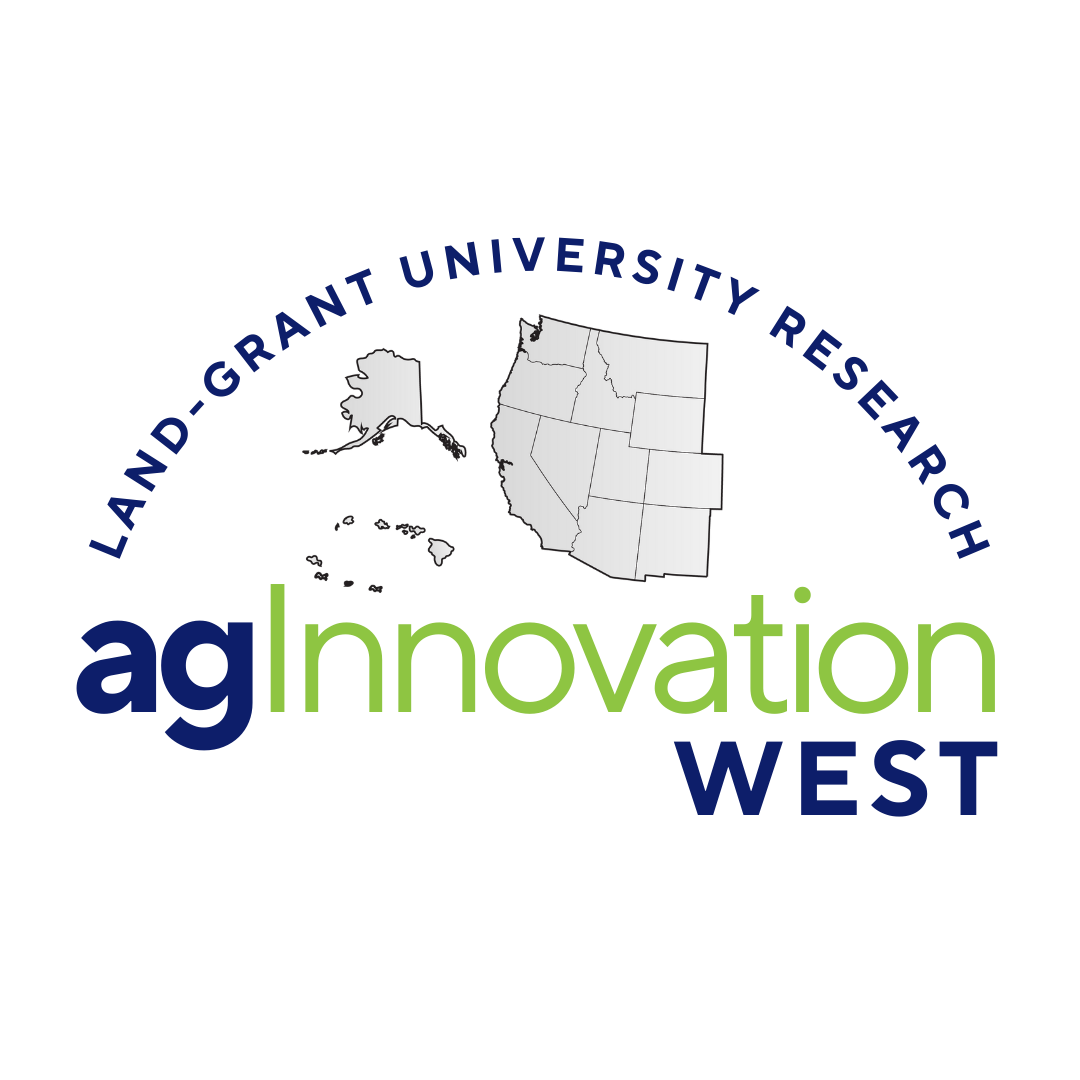
W171: Germ Cell and Embryo Development and Manipulation for the Improvement of Livestock
(Multistate Research Project)
Status: Inactive/Terminating
W171: Germ Cell and Embryo Development and Manipulation for the Improvement of Livestock
Duration: 10/01/1999 to 09/30/2004
Administrative Advisor(s):
NIFA Reps:
Non-Technical Summary
Statement of Issues and Justification
STATEMENT OF THE PROBLEM: Understanding of the underlying biological mechanisms and principles of methods used to produce genetically altered livestock is limited. Furthermore, most of these methods are very inefficient. These technologies will have to be substantially more efficient if we are to realize the advantages of transgenic farm animals for human food and fiber production. These advantages include the production of more desirable products, new products and increased efficiency of the utilization of natural resources. JUSTIFICATION: The development of transgenic animals used for food and fiber production has significant potential for consumers, animal producers, their communities and our environment. Potential examples of such transgenic animals are those producing a milk containing human proteins to make a more desirable human baby formula, those producing a leaner, more desirable meat, or those more efficient in growth, reproduction, wool production, or milk production including those with increased disease resistance (Wheeler and Choi, 1997). Increased efficiencies in production of animal products can be of economic benefit to both consumers and producers and have obvious advantages to the environment in terms of reduced use of natural resources. Current procedures for the production of experimental transgenic animals involve the use of in vitro oocyte maturation, in vitro fertilization, and in vitro culture before and after gene injection (Hasler et al., 1995). This is more practical than recovery of in vivo fertilized embryos but still extremely time and labor-consuming. Currently, more than 10 hours of labor are required to produce a single gene-injected bovine embryo for recipient transfer. When this is coupled with a 20% pregnancy rate and a 12% incorporation rate of the injected gene into the cells contributing to the offspring, an estimated 5,000 hours are required to produce a single transgenic offspring. Before transgenic animals can contribute significantly to production systems, their production will have to be far more efficient. The inefficiencies occur during oocyte maturation and fertilization, during embryo culture and during the incorporation and expression of the microinjected DNA. Short and long term storage of the transgenic embryos is necessary for efficient production of transgenic animals and needs improvement as well (Hurtt et al., 1999). The details of meiotic maturation of oocytes (particularly the details of nuclear maturation) are beginning to be understood. Much of this work has been done with mouse oocytes; yet differences are known to exist between murine oocytes and bovine, porcine, or ovine oocytes (Cran and Moor, 1989). This is just one of the areas where the proposed regional research will contribute to animal production. Although there have been recent advances in nuclear transfer technology in livestock and laboratory species (Wilmut et al., 1997; Schnieke et al., 1997; Wakayama et al., 1998), much is still to be learned regarding the biology and application of these methods to production of transgenic animals. This technology is very inefficient at present (Wilmut et al., 1997 ) and needs improvement before it can be widely used for livestock systems. Research to increase the practicality of making transgenic animals is directly in line with FAIR95 Goal 2 ""Meet consumer needs in domestic and international markets for competitive and high-quality products from animals. Objective l. Increase efficiencies of production livestock. Objective 2. Enhance the quality of products from animals"" (Anonymous, 1993). The economic significance of transgenic animals to U.S. animal agriculture in the future cannot be estimated with any confidence. However, the livestock and dairy industries generated over 68 billion dollars of on-farm receipts in 1992 (Anonymous, 1994). Even little effects on efficiency would repay research costs several times over. A regional research approach is an extremely advantageous means to efficiently approach these problems. Alternative approaches can be tested in multiple laboratories and the effective procedures further tested in the remaining laboratories. Oocyte and embryo procedures appear particularly laboratory dependent; for example, the optimal exposure time for vitrification of mouse oocytes and mouse blastocysts varied significantly among laboratories (Wood et al., 1993; Valdez et al., 1993; Zhu et al., 1993; Shaw et al., 1992). Improvements in nuclear transfer methods and the development of embryonic/somatic cell lines to serve as nuclei donors are other areas that would benefit from this multiple laboratory approach. The use of transgenic and nuclear transfer approaches are also very useful experimentally for obtaining a variety of information. Some examples are insight into the cell cycle, nuclear and cytoplasmic programing or re-programming, genomic imprinting, gene expression and developmental process to name a few. This information can be used in studies to examine basic biological questions, biomedical questions, genetic questions and evolutionary questions as well as applications for agriculture. This proposal will evaluate two areas critically important to the future success of animal biotechnology: 1) the understanding of the developmental biology and underlying biological mechanisms of fertilization and embryonic development and 2) the refinement of methods for production of genetically modified animals to improve livestock production efficiency.
Related, Current and Previous Work
Related regional projects: Related regional research projects are NC-210 Mapping the Pig Genome, NCR-057 Reproductive Physiology, NCR-150 Application of Cellular and Molecular Biology to Animal Science Research, NEC-80 Biotechnology-Animal Development, and S-248 Improving Reproductive Efficiency of Cattle. However, the work is complementary, rather than duplication. The remaining description of current and previous work will be organized by objectives and references are typically to current reviews. 1). Understanding the developmental biology and underlying biological mechanisms of fertilization and embryonic development. Oocyte Maturation - Use of in vitro matured oocytes in combination with in vitro fertilization for the production of livestock embryos in the laboratory is rapidly increasing (Hasler et al.,1995).These techniques have not only tremendous research value in studying the basic biological events that occur during oocyte maturation, fertilization and early embryo development, but also provide an inexpensive and readily procurable source of preimplantation livestock embryos. Successful maturation, fertilization and subsequent embryonic development depend heavily on initial oocyte quality. Procedures that could accurately determine oocyte viability and its likelihood to fertilize and develop as an embryo following in vitro maturation would dramatically improve the efficiency of in vitro embryo production. Alternatively, reliable methods for assessment of viability of in vivo- or in vitro- derived embryos would permit screening of embryos prior to manipulation or transfer procedures. Linking these indicators of embryo viability and successful development with the expression and function of specific genes could also afford valuable insights into mechanisms contributing to embryonic mortality. An integral aspect of in vitro embryo production is successful oocyte maturation. Oocyte maturation is dependent on two separate events: nuclear maturation and cytoplasmic maturation (Fulka et al., 1998). Nuclear maturation refers to the progression of the oocyte from the dictyate stage in meiosis I to metaphase in a meiosis II and extrusion of the first polar body. The process of nuclear maturation occurs spontaneously upon removal of the oocyte-cumulus cell complex (OCC) from the follicle and does not represent a significant problem using established culture conditions. However, nuclear maturation alone is not necessarily indicative of the oocyte's ability to fertilize or sustain continued development (Izadyar et al., 1998). The process of cytoplasmic maturation appears to be the critical factor that determines the success of the subsequent steps to produce viable embryos. Determining and quantifying the indicators of cytoplasmic maturation in the oocyte would enable more efficient selection of oocytes for fertilization and embryo development in vitro. Involvement of cyclin and p34cdc2 kinase in nuclear maturation has been convincingly demonstrated in a number of species (Cran and Moor, 1989, Polanski et al., 1998). Other details of nuclear maturation are yet to be understood and exhibit some species variation. For example, protein synthesis of unknown molecules is required for appropriate nuclear maturation in ovine and porcine oocytes but is unnecessary in murine oocytes. The biology of cytoplasmic maturation is even less well understood (Krisher and Bavister, 1998). The anatomy of the oocyte changes includes the development of microtubule organizing centers and the migration of intracellular organelles (Mattson and Albertini, 1990). The ability of the oocyte plasma membrane to fuse with sperm increases with increasing maturity at least in the hamster (Zuccotti et al., 1991). There appears to be a lack of synchrony between nuclear and cytoplasmic maturation in vitro. Porcine oocytes may achieve nuclear maturation to metaphase II after as few as 24 hours of culture, but do not possess developmental competence until over 40 hours (Grupen et al., 1997). It is possible to prevent spontaneous nuclear maturation by addition of dibutyryl cAMP to the maturation medium (Nekola and Smith, 1975) and thus, allow more time for cytoplasmic maturation to occur while avoiding nuclear aging. Funahashi et al. (1997a) reported the addition of dibutyryl cAMP during the first 20 hours of maturation increased homogeneity of nuclear maturation among the maturing oocytes and resulted in higher pregnancy rates after fertilization and embryo transfer. Developmental competence might be improved further by delaying nuclear maturation for a longer period of time because oocyte maturation in vivo requires several days as compared with the 42 to 44 hour maturation period typically reported for porcine oocytes. Studies have indicated that morphological characteristics such as appearance of cumulus cells, oocyte size, location in the ovary and the time of polar body extrusion are related to the ability of oocytes to fertilize and develop into viable embryos (Younis al., 1989; Arlotto et al., 1990, 1992; Dominko and First, 1992). There are distinct morphological differences between in vivo and in vitro matured porcine oocytes (Wang et al., 1998). Events following fertilization such as sperm decondensation, pronucleus formation and completion of meiosis appear delayed for in vitro matured porcine oocytes (Laurincik et al., 1994). The slow or incomplete cortical granule release following sperm penetration (Wang et al., 1997) and uneven distribution of cortical granules within the oocyte (Wang et al., 1998) might be related to the high incidence of polyspermy reported for porcine oocytes matured and fertilized in vitro. Overall, the limited developmental competence of porcine oocytes matured in vitro appears to be related to failure of adequate cytoplasmic rather than nuclear maturation (Nagashima et al., 1996). Other reports suggested that activities of specific enzymes in OCC such as histone-1-kinase or quantities of biochemicals, such as prostaglandins, produced by OCC in culture are indicators of fertilization and potential embryo development (He et al., 1973). A multitude of studies have employed the addition of gonadotropins to maturation medium at the initiation of culture. However, there might be an advantage in either delaying or sequentially adding these hormones. Delaying the addition of gonadotropins by as little as 12 hours has been reported to improve developmental competence of porcine oocytes (Funahashi et al., 1997b). Studies investigating the long-term culture or preantral follicles have utilized FSH and estradiol in the culture medium (Hiro et al., 1994). Both estradiol (Eroglu, 1993) and FSH (Singh et al., 1993) have been shown to delay germinal vesicle breakdown (GVBD). Pre-culture of preantral follicles in FSH enhances GVBD and cumulus expansion in response to LH or hCG (Eppig, 1991). Therefore, sequential addition of FSH followed by LH might be effective in delaying nuclear maturation while promoting cytoplasmic maturation so that better maturation synchrony can be achieved. Also, sequential addition of FSH and LH should prevent premature luteinization of cumulus cells and a shift from estradiol to progesterone production. In vivo, the shift from estradiol to progesterone production by follicles occurs late in maturation, as the oocyte approaches metaphase-II (Xie et al., 1990). There also might be an advantage in the addition of insulin to oocyte maturation medium during the initial stages of culture because insulin decreases follicular atresia in vivo (Matamoros et al., 1991). Follicular fluid composition has also been shown to be different between oocytes which develop to embryos and those which did not. These reports have demonstrated differences in concentrations of several proteins in follicular fluid, including plasminogen activator, that differ between fertilized and nonfertilized oocytes (Cannon and Menino, 1998). It has been shown that equine oocytes require a longer in vitro maturation period than bovine oocytes (Shabpareh et al., 1993). Considerable research was conducted on the effects of follicular fluid on maturation of bovine oocytes; we concluded that preovulatory follicular fluid before the LH surge inhibits maturation, while by 20 hours after the LH surge, follicular fluid stimulates oocyte maturation, even in the presence of inhibitory factors (Romero and Seidel, 1994, 1996). Furthermore, 20-hour follicular fluid during maturation produces embryos that develop to blastocysts more readily than controls. The primary stimulatory factor may be EGF (Kato and Seidel, 1996), whereas, it has been shown that follicular walls (theca interna cells) are the source of the inhibitory factors (Kato and Seidel, 1998). Fertilization and Sperm Capacitation - Fertilization and initial cleavage stages occur in the mammalian oviduct. Although production and development of farm animal embryos have become routine, their development in vitro remains far below in vivo embryos. The inability to mimic in vivo conditions may contribute to sub-optimal development of in vitro-produced embryos. The secretion of several factor(s) from the mammalian oviduct may contribute to the superior development of in vivo produced embryos. A high molecular weight oviductal glycoprotein (OGP) is synthesized and secreted by the secretory cells of the oviduct. The presence of OGP is highest during the periovulatory period and declines thereafter, suggesting its potential role during in vivo fertilization and possibly during the initial cleavage stages (Malette et al., 1995; Verhage et al., 1998). In most IVF systems sperm concentrations are adjusted to maximize fertilization rates without regard to exposure of oocytes to high concentrations of spermatozoa. However, under in vivo conditions, oocytes are only exposed to a few spermatozoa. The production of oxygen radicals from high concentrations of spermatozoa (Aitken et al., 1996) may adversely affect embryo development and implantation. As part of the cytoplasmic maturation of oocytes, the protein composition of the oocyte plasma membrane changes including an increased ability to bind and/or fuse with sperm (Zuccotti et al, 1994; Cohen et al., 1996). Oocyte receptors for the sperm plasma membrane are either described/ hypothesized as the complementary receptors for putative ligands or in one case as integrin heterodimers. Integrins are exposed on the surface of many mammalian oocytes and are complementary to one of the apparent sperm ligands in rodents. Hence, integrin molecules are likely to be one of the sperm receptors on rodent oocytes; integrin heterodimers do not meet the presumed criteria for tissue and species specificity. Continued synthesis/export of sperm receptors to the plasma membrane following ovulation was demonstrated previously in mice (Kellom et al., 1992). Wheeler et al. (1996) has verified that bovine oocytes increase their ability to bind sperm plasma membrane during the 22 hours of in vitro maturation and that this increase is dependent upon protein synthesis. There has previously been an evaluation of plasma membrane protein components in individual oocytes. Recently, a putative oocyte plasma membrane protein (POMP) receptor for sperm was identified based on western ligand blotting, affinity chromatography, localization on oocyte surface, and inhibition of sperm-oocyte binding by FAB to a MAP peptide of this protein (Berger et al., 1998; 1999; Yen and Berger, 1999). Sperm gain the ability to bind and fuse with the oocyte plasma membrane in the equatorial segment during capacitation. Modification of one or more of the ligands or exposure of one or more of the ligands for the oocyte plasma membrane would appear to be an essential component of capacitation. A number of potential sperm ligands have been proposed to interact with the oocyte in different species; species specificity (at least among mammalian orders) would be expected. Ash et al. (1995) identified four predominant binders of the oocyte plasma membrane as potential porcine sperm ligands for the porcine oocyte plasma membrane.Using an antibody to the DRKD N-terminal peptide, antigenic cross-reactivity between this porcine sperm plasma membrane recognizing porcine oocyte plasma membrane and a bovine sperm plasma membrane recognizing bovine oocyte plasma membrane was demonstrated. Ligand/receptor similarity might be anticipated among species within a mammalian order since whole gametes demonstrate some cross species interaction. Assisted Fertilization - It has been shown that capacitation status of bovine sperm for intracytoplasmic sperm injection (ICSI) is irrelevant, and that there is a benefit of activation of oocytes with the calcium ionophore A23187 (Chen and Seidel, 1997). Further, a beneficial effect has been shown by activating equine oocytes with ICSI (Kato et al., 1997). A novel method of increasing fertilization rates in vivo via a sperm-oocyte adhesive peptide (Amann et al., 1999) may also be promising in vitro. GnRH was also shown to increase the success rate of bovine IVF (Funston and Seidel, 1995). Sex Determination - The ability to pre-select the sex of offspring would have tremendous economic benefit to beef and dairy producers alike. Numerous efforts have been directed toward development of procedures for pre-selecting the sex of offspring. One approach has been to attempt to physically separate X- from Y-bearing spermatozoa (Amann, 1989). To date, modified flow cytometry (Johnson et al., 1994) is the only separation procedure that has been successful in sorting X- from Y-bearing spermatozoa. However, this procedure does not have widespread application at the present time, due to the cost of the equipment and limitations on sorting rate. It may be possible to significantly alter the sex ratio by screening and then selecting, ejaculates with a naturally altered ratio of X- to Y-bearing spermatozoa. Chandler et al. (1998) reported that within sires, the ratio of X- to Y-bearing spermatozoa from different ejaculates of semen can be skewed significantly toward one sex and this skewed ratio appears to translate into altered sex ratio of offspring. The ratio of X- to Y-bearing spermatozoa of ejaculates within bulls was found to be significantly skewed (~84%) in about 20% of the ejaculates evaluated. A number of physiological and environmental factors have been reported to be related to a male effect on sex ratio (Krackow, 1995). Studies are needed to confirm that the ratio of X- to Y bearing spermatozoa is skewed in some ejaculates of semen and to identify factors influencing or related to the skewed ratio. Embryo Development - Although the methodology for maintaining embryos from livestock in culture has existed for many years, the ability of the present systems to support normal development is limited. This is especially true of early embryos that often exhibit developmental blocks in vitro (Beckmann and Day, 1993, Krisher and Bavister, 1998). These blocks are thought to be the result of inadequate culture systems since similar blocks are not found in vivo. In addition, numerous studies have shown that there is a decline in embryo viability corresponding to the length of time they are held in culture (Davis, 1985). More recently, co-culture systems have been developed to at least partially overcome the deficiencies associated with earlier systems (Trounson et al., 1994). Although a variety of cell types have been examined for use in co-culture systems, the greatest success has been achieved using oviduct cells. The benefits of co-culture systems in promoting in vitro development are well established, but the mechanisms by which these benefits are achieved have yet to be elucidated. Despite the increase of their use, co-culture systems are not without their limitations. First, by their very nature, co-culture systems are undefined. This makes comparisons of results difficult between laboratories as well as within laboratories when experiments are conducted over an extended period of time using different batches of oviduct cells. Second, co-culture systems require that researchers maintain cell lines or continually establish primary cell cultures to maintain the culture system. Third, it is difficult, if not impossible, to conduct basic studies in areas such as embryo metabolism, embryonic protein secretion and/or protein binding to embryos in co-culture systems due to the involvement by the oviduct cells. Evidence is accumulating that growth factors have an autocrine and paracrine role in embryo development (Gandolfi, 1994; Heyner et al., 1994, Glover and Seidel, 1998).Tremendous effort will be required to define these effects but secretion of growth factors by the co-cultured cells may be at least one of the mechanisms for the beneficial effect of co-culture (Winger et al., 1997). An extensive series of experiments confirmed the detrimental effects of high oxygen and beneficial effects of certain antioxidants on in vitro development of bovine embryos (Kato et al., 1998; Olson and Seidel, 1999). Optimal ways have been determined to condition media with buffalo rat liver cells for culturing bovine embryos (Funston et al., 1997). Studying gene expression in elongating bovine embryos has shown the IGF system appears to have important regulatory roles (Keller et al., 1998). One of our stations (Colorado) has identified several genes with increased expression as embryos elongate (Glover and Seidel, 1998). Extracellular matrix (ECM)-degrading proteinases are believed to play pivotal roles in early embryo development and embryo-uterine interactions (Cross et al., 1994). ECM-degrading proteinases include the plasminogen activator (PA)-plasmin system and the family of matrix metalloproteinases (MMP) (Vassalli and Pepper, 1994). To date, the laboratory of one of our contributing stations (Oregon) has demonstrated that endodermal cells express PA during migration in vitro in sheep and cattle. During the periods of endodermal cell migration and trophoblastic expansion and elongation, ovine embryos express PA, MMP-9 and three MMP inhibitors (TIMPs-1, -2 and -3). Blastocyst production by in vitro methods has plateaued at around 40% despite various attempts to improve culture conditions. The 40% development rate falls short of the 85 to 95% that occurs in vivo. Various attempts have been made by using co-culture systems in conjunction with adding or varying media components or culture conditions (i.e. media, co-culture cell type, medium supplements, gas atmosphere, various environmental factors etc); however, in vitro production of blastocysts still hovers at or below 40% , with recent reports ranging from 20 - 30% (Eyestone and First 1989, Thibodeaux et al. 1992, Freeman et al. 1993, Choi et al. 1998, Krisher and Bavister 1998 and Lim et al. 1998). Furthermore, in vitro-produced blastocysts are usually developmentally retarded with fewer inner cell mass cells compared with embryos developed in vivo. The primary problem associated with current culture systems is that they do not mimic the changing oviductal/uterine environment. This appears to be supported by the fact that blastocyst development is influenced by the stage-specific composition of culture medium. The apparent lack of biologic culture conditions necessitates further innovation in tissue culture methodology and the pursuance of further research in this area. 2). Refinement of methods for production of genetically modified animals to improve livestock production efficiency. Nuclear Transfer - Nuclear transfer, which involves the transfer of each nucleus from a multicellular embryo into an enucleated metaphase II oocyte, has been developed in several species (Willadsen, 1986; Prather et al., 1987; Stice and Robl, 1988.). Nuclear transfer has become an active field of research over the last decade, culminating in reports over the past two years of live offspring from differentiated cells of sheep, cattle, and mice (Schnieke et al., 1997; Wells et al., 1997; Cibelli et al., 1998; Kato et al., 1998; Wakayama et al., 1998). Dolly was not a fluke. Dozens of laboratories are producing calves and lambs whose genetic parents are somatic cells, often derived from adults. This technology has three broad applications: 1) applied animal breeding to copy outstanding animals (e.g., proven bulls), 2) a tool for basic research, (e.g., mechanisms of differentiation), and 3) a biotechnological tool (e.g., vastly simpler approaches to making transgenic farm animals). Although offspring have been produced in livestock using nuclear transplantation, the efficiency rate has been low (Wilmut et al., 1997).In each of the species reported to date, the efficiency is !\ 10% (1-6% in sheep, 1-4% in cattle, 1% in pigs, and 10% in rabbits). The factors affecting the efficiency of nuclear transplantation are: enucleation of the recipient oocyte, fusion of the transplanted nucleus to the enucleated oocyte cytosol, activation of the oocyte, and ""re-programming"" of the transferred nucleus. In each of the studies previously indicated, if we evaluate the efficiency of each of these steps, activation may be the factor responsible for the greatest loss in efficiency (Collas and Robl, 1990). However, the general consensus of those in the field is that nuclear transfer efficiency is much too low to be economically viable except in very limited applications. The low efficiency also makes it an expensive research tool. Any increase in efficiency will greatly enhance the value of this technology and contribute to our understanding of changes that must occur in chromosomes to allow embryonic gene expression patterns. It is also critical to begin to understand how potentially subtle modifications in the nuclear structure impact the ability of a cell to contribute to production of offspring and yet another to fail. An important aspect of this field in the past and the future concerns understanding the abnormalities of calves cloned by nuclear transplantation (Seidel et al., 1997; Garry et al., 1998). Such calves and lambs have high rates of fetal and neonatal death. At birth, they are hypoxic, hypoglycemic, hypothermic, and readily die (Garry et al., 1998). It has been hypothesized that these animals have a genetically or epigenetically abnormal placenta, since the calves themselves appear normal genetically (Garry et al., 1996). Transgenic Methodologies - The ability to produce novel proteins in the mammary gland of domestic animals will have an important impact on agriculture. With recent success in the ability to use somatic cells as nuclear donors which result in the production of offspring, this objective appears finally to be attainable at rates which will allow use of domestic animals. The ability to routinely introduce novel genes into tissue culture cells will greatly increase the types of genes utilized for production of transgenic animals, other than mice. Several years ago one of our contributing stations (Colorado) combined transgenics with in vitro-produced bovine embryos (Thomas et al., 1993), and produced the first transgenic calf demonstrated to, in fact, express a transgene (Bowen et al., 1994). Additional previous work has included the production and study of transgenic mice incorporating a variety of transgene constructs to determine optimum promoter elements (Gutierrez-Adan et al., in press) or fusion gene constructs for use in dairy goats and cattle. Targeted milk properties include processing functionality (Maga et al., 1995; Gutierrez-Adan et al. 1996), anti-microbial properties (Maga et al., 1997), and the application of antisense constructs to obtain efficient down-regulation of protein secretion into the milk (Sokol et al., 1998). Transgenic animals have great potential to livestock production but the potential is still unrealized. The low efficiency in their production is a primary reason (Ebert and Schindler, 1993; Halter et al, 1994). These inefficiencies occur at all stages. An aspect of great importance in this regard is the isolation and manipulation of undifferentiated, pluripotent embryonic cells. There are two types of pluripotent/totipotent embryonic cells, embryonic stem (ES) cells and embryonic germ (EG) cells. ES cells are isolated in culture from blastocyst-stage embryos, and EG cells are isolated from cultured primordial germ cell (PGC). Development of a stem cell line for a species that could be used as a recipient of the desired DNA and subsequent nuclear transfer of the stem cell nuclei or the incorporation of the stem cells into an embryo with the resulting birth of a chimeric animal are potential solutions to the inefficient incorporation of the injected DNA. ES cells first were isolated from mouse embryos (Evans and Kaufman, 1981; Martin, 1981 ) and since have been used as a model for mammalian embryogenesis and more recently for genetic manipulation. Due to their ability to integrate foreign DNA and thereafter differentiate into any and all tissues of a normal individual, ES cells are effective vehicles for genetic engineering and for creating laboratory animal models of human diseases. Despite considerable effort, progress toward isolation of ES cells from agricultural species has been slow. Some of the more promising results have been achieved with pigs (Wheeler, 1994). To date, production of embryonic stem cell lines has been much more difficult in livestock species than in mice (Sims and First, 1994; Wheeler, 1994, Wheeler et al., 1995). In the early 1990s, several laboratories reported isolation of ES-like cells from cultures of primordial germ cells (PGC!/s)(Matsui et al., 1992; Resnick et al., 1992). These cells were called EG cells to distinguish them from blastocyst-derived ES cells, but EG and ES cells appear to share many characteristics, including the ability to differentiate into numerous cell types. One of our contributing stations (California) successfully isolated EG cells from porcine PGC (Shim et al., 1997, Shim and Anderson, 1998;), the first example in a species other than the mouse. Recent successes with isolation of ES and EG cells from agricultural species have been exciting, but generally these ES and EG cells have a low efficiency of incorporation into normal, developing embryos. Moreover, in no instance have livestock ES or EG cells been shown to develop into gametes, a prerequisite to their use in genetic engineering. Several laboratories involved in the W-171 project have a history of productive research with ES and EG cells. By combining the resources, ingenuity and collaborative efforts from these laboratories, this Regional Research Project could yield undifferentiated cell lines of practical value to animal agriculture, a phenomenal contribution to animal agriculture. Cryopreservation - Cryopreservation of in vivo produced bovine embryos is quite successful and very limited success has recently been achieved with other species such as late-stage porcine embryos (Pollard and Leibo, 1994; Nagashima et al., 1994; Dobrinsky, 1997). Initially, in vitro matured and fertilized embryos appeared more susceptible to damage during freezing and thawing (Leibo and Loskutoff, 1993, Arau et al., 1996). Alterations in in vitro maturation and culture can affect the susceptibility of the in vitro-produced oocytes to cryodamage. Recently, some of our group have had an emphasis on equine oocytes and embryos. The large equine embryo routinely collected nonsurgically is particularly challenging to cryopreserve. Recently, a new concept was developed, step-down equilibration, to cryopreserve such embryos successfully (Young et al., 1997). This evolved from basic research of permeability of equine embryos to cryoprotectants (Pfaff et al., 1994). Recently, equine and bovine oocytes have successfully been vitrified (Hurtt et al., 1999). They showed that matured oocytes survived vitrification better than immature ones.
Objectives
-
Understanding the developmental biology and underlying biological mechanisms of fertilization and embryonic development
-
Refine methods for production of genetically modified animals to improve livestock production efficiency
-
Methods
Objective 1: The overall aim of the research to be conducted under this objective is to gain a more precise understanding of the biological requirements for successful oocyte maturation, fertilization, and subsequent embryonic development. The production of live offspring is dependent upon all of these events occurring in a well-orchestrated fashion. Collaboration in the area of porcine oocyte maturation will occur. California will examine the presence of a putative oocyte plasma membrane protein (POMP) receptor before and after partial in vitro maturation and during incubation under maturation conditions with the presence of cycloheximide to inhibit protein synthesis. Oocytes will be examined using a confocal microscope, and the intensity of fluorescence in ellipses spaced around the oocyte plasma membrane will be measured and analyzed. In a related study, porcine ovaries will be fixed in Bouin's solution, embedded in paraffin, and sections subjected to immunohistochemistry. Alternate sections will be incubated with pre-immune IgG or stained with hematoxylin and eosin. Presence of POMP at the oocyte surface during oocyte maturation in vivo will be examined. Arkansas, Iowa and Illinois will investigate the effects of maturation time and media supplementation on the developmental competence of porcine oocytes matured and fertilized in vitro. Porcine oocyte-cumulus complexes (OCC) will be recovered from the ovaries of gilts at slaughter and will be matured for 24, 36 or 48 hours in M-199 supplemented with various combinations of serum, FSH, estradiol, insulin and dibutyryl cAMP. After the initial culture, a portion of the OCC will be removed for evaluation of germinal vesicle breakdown. The remaining OCC will be matured for an additional 24 to 48 hours in M-199 supplemented with serum, LH and cysteine. Following final maturation, oocytes will be evaluated for nuclear maturation and electrically activated to assess parthenogenic development. After the maturation treatments have been assessed, the best treatment/time combination will be used in conjunction with IVF to evaluate oocyte developmental competence. The rate of polyspermy will be compared after pre-exposure of oocytes and/or spermatozoa to oviductal cell secretions. Colorado will use bovine and equine oocytes from slaughterhouse ovaries for studies on in vitro maturation. Connecticut will use bovine oocytes from pre-pubertal ovaries for studies on in vitro maturation in collaboration with Louisiana. Oocytes will be matured in vitro using standard procedures (Romero and Seidel, 1996), except that chemically defined media will be used for most studies (Olson and Seidel, 1999). The dose-response of gonadotropins; dose-responses of sulfhydryl-donating agents such as glutathione, cysteamine, and _-mercaptoethanol; addition of amino acids present in oviductal and follicular fluid; benefits of the growth factors EGF and IGF-1; and dose responses of decreasing sodium ion will be the main topics studied. Colorado also will evaluate success via metabolic rates, cortical granule distribution, and zona hardening, as well as the more conventional fertilization and embryonic developmental rates. Louisiana, Colorado and Illinois will collaborate on methods to assist fertilization of equine oocytes. Intracytoplasmic sperm injection (ICSI) seems to be the most probable method to produce live foals via in vitro embryo production techniques (Squires et al., 1996; Cochran et al., 1998). Joint efforts are planned to continue development of ICSI technology for in-field use in the cow (Colorado, Oklahoma, Louisiana), small ruminants (Louisiana, Iowa) and swine (Illinois). Colorado will use the new piezo-injection technology for ICSI in cattle and horses. An important key to the success of ICSI is proper oocyte activation. Colorado will research injection of the sperm activating protein that causes oscillations of free intracellular Ca++ in oocytes (Fissore et al., 1998). This protein will be isolated from bull and stallion semen, and dose responses will be generated by injection at different times relative to ICSI (Chen and Seidel, 1997). Colorado also will study other methods of attaining physiological oocyte activation including injecting IP3 and other agents affecting this pathway. This work will coincide with studies conducted by Utah where mechanisms associated with activation of bovine oocytes will be evaluated. The potential role of integrins in the interactions between the sperm and oocyte; any association between integrin associated intracellular calcium transients and either IP3 or ryanodine receptors and their possible link with specific integrin subunits found on the surface of bovine oocytes will be the focus of these investigations. Studies will be conducted on in vitro sperm capacitation because capacitation is needed for optimal in vitro production of embryos. California will determine, using flow cytometry, if bovine sperm with varying abilities to interact with oocytes vary in the binding of the DRKD antibody to the sperm surface, either initially or after normal in vitro capacitation. Initial comparisons will be made among samples used for in vitro fertilization within a single laboratory, but subsequent studies will focus on semen samples obtained from the collaborating stations (Colorado, Louisiana, Utah) that use slightly different in vitro embryo production conditions. Fresh and frozen epididymal sperm, and techniques for capacitation, will be studied by several stations (California, Colorado, Iowa, Illinois, Louisiana) for use in IVF procedures in various farm animal species. California and Illinois will investigate porcine sperm, California and Colorado will study bovine sperm, and Colorado, Iowa, and Louisiana will examine equine sperm. Prior research (Louisiana) has identified differences among species, and it has been noted that epididymal stallion sperm, for example, does not require capacitation in order to produce equine embryos in vitro. A major collaborative effort among stations will focus on fertilization and subsequent development of in vitro matured bovine oocytes. All oocytes will originate from a single source (Wisconsin) and will be shipped to participating stations (Colorado, Connecticut, Iowa, Louisiana, Oklahoma, and Utah) for subsequent investigations. To the best of our knowledge, this will be the first time that oocytes from a common source will be utilized in such a manner. This uniformity in pre-fertilization conditions will enable a more clear-cut interpretation of experimental results obtained across stations. Likewise, this approach affords the opportunity to investigate various methods of oocyte maturation on subsequent embryonic development using standardized protocols for fertilization and culture. Preimplantation embryo development in vivo depends on the interaction between the embryo and the oviductal/uterine environment. Interactions occur primarily between proteins (e.g. changes in type, quantity and location of specific proteins such as receptors, enzymes etc.) and the embryo and/or oviductal and uterine epithelial cells. Colorado, Illinois, Louisiana, Oklahoma, and Utah will investigate the role of various glycoproteins on embryonic development. Oklahoma will focus on bovine oviduct-specific glycoprotein (Thibodeaux et al., 1995), while Colorado will study the secreted oviduct glycoprotein that binds to oocytes (Verhage et al., 1998) and a seminal plasma peptide, Fert+, that recently was shown to improve fertility of subfertile bulls with artificial insemination (Amann et al., 1999). Utah will study changes in protein profiles (i.e., type, amount and distribution) of OVEP and UEC co-cultures, which will provide a window through which external processes (epigenetic) of embryonic development can be studied. They will use electrophoresis (i.e. 2-D and capillary IEF and standard electrophoresis procedures) to investigate kinetic changes of protein patterns, and ICC (immunocytochemistry) and FISH techniques will be used to detect changes of specific proteins (receptors). Colorado and Iowa will investigate the role sulfhydryl-donating agents such as glutathione, cysteamine, and _-mercaptoethanol in embryonic development using a chemically defined medium (Romero and Seidel, 1996; Olson and Seidel, 1999; Caamano et al., 1996; Caamano et al., 1998). Colorado will continue their dose- response studies with various antioxidants, particularly Vitamin E and _-mercaptoethanol (Olson and Seidel, 1999) and carbohydrate sources (e.g., fructose; Chung et al., 1996. Oregon plans to evaluate expression of components of the PA-plasmin system and the MMP during the periods of endodermal cell migration from the inner cell mass, formation of the bilayered trophoblast, and trophoblastic expansion and elongation in sheep and cattle embryos. They intend to determine the complete phenotypic expression pattern and the cellular localizations of the ECM-degrading proteinases and their associated inhibitors during these periods of development. Understanding these systems during specific developmental events may afford valuable insights into embryonic mortality as well as provide targets for investigation of abnormal placenta formation in pregnancies produced by nuclear-transferred embryos. Novel approaches to in vitro culture will be investigated by Colorado, Iowa, Illinois, and Louisiana. Colorado has confirmed data of others (Vajta et al., 1997) that submarine culture (using a water bath) appears to improve embryonic development greatly compared to standard incubators, and they will attempt to determine the reasons for this enhanced development. Illinois has devised a new micro-fluidic embryo culture device, and this will be tested at other stations (Iowa, Louisiana, ) Oregon, Utah, Illinois and Colorado will investigate gene expression in developing preimplantation embryos. Colorado will characterize the genomic organization and functions of genes that are expressed in greater amounts in day-17 than in day-15 bovine embryos, and gene expression between in vitro and in vivo embryos will be compared using differential display (Glover and Seidel, 1998). Illinois will characterize the gene expression of porcine embryos from the 1-cell to blastocyst stage using, RT-PCR, differential display PCR and capillary electrophoresis. Oregon will collect embryos from superovulated donor cows and ewes, and RT-PCR will be used for determining the phenotypic expression pattern of the genes of interest. Localization of the cells expressing the specific genes will be conducted using in situ hybridization. For experiments involving analysis of secreted proteins by cultured embryos, isolated inner cell masses or trophoblast, conditioned medium will be recovered and analyzed by immunoblotting and casein and gelatin zymography. Utah will also use RT-PCR to trace sequential gene expression during embryo development. Recently, great interest has been expressed in the ability to control the sex of offspring. Arkansas, Colorado, and Iowa will be involved with studies on offspring sex ratios. Arkansas will investigate if a naturally occurring skews occurs in the ratio of X- to Y-bearing spermatozoa among ejaculates, and, if so, will study factors that potentially influence the altered ratio of or X- to Y-bearing spermatozoa. Semen will be collected from bulls at varying intervals, and the ratio of X- to Y- bearing spermatozoa of each ejaculate will be determined using X- and Y-specific DNA probes in conjunction with fluorescence in situ hybridization (Hassanane et al., 1998). Seminal plasma will be evaluated for components that might be related to altered sex ratio on semen. Ejaculates identified as having the sex ratio skewed (70% or greater X- or Y-bearing spermatozoa) will be used in conjunction with in vitro fertilization to confirm that altered sex ratio of semen translates into altered gender ratio in vivo. Embryos will be sexed using commercially available male-specific DNA probes. If possible, arrangements will be made to confirm through artificial insemination and fetal sexing, that altered sex ratio of semen translates into altered sex ratio of offspring. Colorado will continue its studies on non-surgical deep intrauterine insemination using sperm sorted with the aid of a modified flow cytometer, while Iowa will continue its studies on the use of a vaginal conductivity probe to pinpoint an insemination time that may lead to an altered calf sex ratio. Understanding ovarian follicular dynamics is important for optimizing the production of oocytes for in vitro embryo production and for in vivo embryo production via conventional superovulation. In an effort to produce excellent quality oocytes for IVF, collaborative efforts are planned to evaluate protocols to maximize follicular growth and oocyte collection by transvaginal ultrasound-guided oocyte recovery (Louisiana, Iowa, Connecticut). These studies will focus primarily on prepuberal, pregnant, and postpartum females. Preliminary studies have indicated that transvaginal ultrasound oocyte recovery is possible in goats (Han et al., 1996; Graff et al., 1999) and horses (Bruck et al., 1992; Cook et al., 1992; Meintjes et al., 1994), as well as cows (Pieterse et al., 1988, 1991a,b; Looney et al.,1994. One station (Louisiana) has offered to train personnel from other stations to enhance collaborative efforts among the contributing stations. An electronic heat detection system (Heat Watch) has recently become available to livestock producers in the United States, and a joint effort is planned to evaluate this system in different regions (Louisiana, Illinois, Iowa, Utah, Connecticut) using dairy and beef females. The initial focus of this research will be to evaluate differences in the onset and duration of behavioral estrus in similar genotypes during different climates/seasons of the year. Ultimately, these data will be used to predict the best time for transvaginal oocyte collection that will optimize the number of oocytes collected from each donor female. Harvesting the best quality oocytes is important not only for IVF, but also for nuclear transfer or transgenic research programs. Objective 2: The overall aim of the research to be conducted under this objective is to enhance the success of each step in the embryo manipulation process that is required for successful production of genetically modified embryos and offspring. Continual advances in gene transfer techniques and nuclear transfer methodology have led to a crucial need for more research that will lead to increased incorporation of these methods into livestock production systems. Colorado, Louisiana, Connecticut, Illinois and Utah will conduct studies on nuclear transfer. Utah will investigate methods for increasing activation rates of bovine nuclear transfer embryos. They also will conduct experiments designed to evaluate the ability of the early process of fetal and embryonic development to ""reset"" the developmental clock of adult bovine fibroblasts. These studies will evaluate subtle modifications in nuclear components that result in some cells having the developmental competence to produce offspring and others to result in failure (Blackburn, 1991; Harley et al., 1990; Allsopp et al., 1992; Vaziri et al., 1993; Bodnar et al., 1998). Utah also will continue to study the developmental potential of hybrid (sheep-cow) nuclear transfer embryos to produce viable offspring. Utah, Louisiana, Illinois, Connecticut and Colorado will investigate the use of somatic cells for nuclear transfer. Louisiana and Illinois will develop methodology to establish caprine, ovine, equine, and bovine somatic cell lines that are useful for nuclear transfer, while Colorado will optimize methods of transplanting nuclei from fetal fibroblasts and cumulus cells into activated enucleated oocytes. Connecticut will test the nuclear transfer efficiency of different donor cell types for nuclear transfer and explore the possibility of transfecting cultured cells for transgenesis. Utah will investigate the use of somatic cell lines (embryonic, fetal and adult) from domestic animal species as vectors for the production of transgenic animals using nuclear transfer. Louisiana and Colorado also will study the normalcy of embryonic and fetal development in vitro and in vivo because it has been well documented that a portion of the nuclear transfer offspring have been abnormally large at birth (Willadsen et al., 1991). Efforts are needed to identify the cause of this developmental problem for the success of this new technology to be accepted by the livestock community. Colorado will continue to search for genetic or epigenetic changes in cloned embryos vs. control embryos. They will particularly examine the placenta, by using differential display (Glover and Seidel, 1998) to compare differences in mRNA content of various genes in the cotyledons of cloned vs IVF vs control day-45 pregnancies (collected by a novel, nonsurgical procedure). Differential expression of candidate genes will be confirmed by Northern blots; genes will be cloned, sequenced, and identified and/or characterized. California, Colorado, Connecticut, Illinois, Iowa, Louisiana, and Utah will investigate techniques for the efficient production of transgenic livestock. California and Illinois will test in vivo differentiation of porcine ES and EG cells after being combined with embryos of various developmental stages. In addition, Illinois will test in vivo differentiation of porcine ES cells in the SCIDs mouse tumor system. Porcine cell lines that have been screened for markers of undifferentiated cells and that have been shown using tests for in vitro differentiation to be pluripotent will be tested for in vivo differentiation. California will share cell lines among participating laboratories in order to test these cell lines under the varying conditions found in the various laboratories. Iowa will investigate methods for more efficient isolation of porcine primordial germ cells. Colorado, Connecticut, Utah, Illinois and Louisiana will conduct studies with transfected cell lines. Colorado will conduct experiments with cattle and sheep and will use GFP (green fluorescent protein) as a reporter gene to study placental expression driven by the regulatory regions of molecules such as placental lactogens, interferon tau, and molecules from other species such as hCG and eCG subunits. Illinois will conduct transfection experiments with the bovine alpha-lactalbumin gene and promoter to drive mammary expression of IGF-1 and beta 1,4 glactosyltransferase. Somatic cells will be transfected in vitro, characterized, and frozen in aliquots for later nuclear transplantation. Similarly, Utah will transfect somatic cell lines with genes designed for expression of foreign proteins in the mammary gland and for enhancement of the resistance of animals to disease of economic importance to agriculture. Louisiana will conduct transfection studies using embryo-derived stem cell lines from goats and cattle. Colorado, Iowa, and Louisiana will investigate methods for cryopreservation of oocytes and embryos. Colorado, Louisiana, and Iowa will investigate the potential use of vitrification for the freezing of mammalian oocytes and embryos. Louisiana will compare conventional and vitrification methodologies for the cryopreservation of oocytes from the mouse, goat and cow, while Colorado will study vitrified oocytes (cattle and horses) by evaluating fixed and stained specimens (Hurtt et al., 1999). Louisiana and Iowa will evaluate various non-conventional apparatuses (e.g., electron microscope grids) for their ability to enhance the cooling rate during the cryopreservation process. Having a supply of excellent quality oocytes and embryos available at any time would certainly enhance research on nuclear transfer, ICSI, and/or transgenesis. Much remains to be learned concerning concentration, timing of addition, and timing of removal of cryoprotectants for both vitrification and conventional cryopreservation. Colorado and Iowa will investigate cryopreservation of equine and porcine blastocyst stage embryos. Colorado will expand step-down equilibration studies with large equine blastocysts (Young et al., 1997), compare concentrations of glycerol and ethylene glycol (4 vs 6 M), investigate whether the step-down procedure (decreasing cryoprotectant) before freezing is, in fact, beneficial. Iowa will examine the biophysical properties of porcine blastocysts in an attempt to develop a suitable method for cryopreservation of intact, nonmanipulated porcine blastocysts.Measurement of Progress and Results
Outputs
- Results from the research are expected to have tremendous impacts on scientists, persons engaged in animal production, and consumers. Gaining insights into the biological mechanisms underlying early embryonic development will enable researchers to devise better systems for production of embryos genetically capable of more efficient production of food and fiber. Some of the immediate tangible benefits from this research will include the generation of scientific publications that reflect the advancement of science, development of new methodologies that can be utilized in the private sector, and education of society through extension reports and popular press publications. Once the new techniques have been incorporated into biomedical research and production agriculture, consumers will see new products emerging onto the marketplace as well as traditional products being sold at a reduced price because of their more efficient production. It will also offer the opportunity to decrease the number of animals needed to produce products that reach market, lessening animal waste production and reducing potential environmental concerns.
- Studies on oocyte maturation, fertilization, and embryonic development are crucial to enhance livestock reproductive efficiency. Once the underlying biological mechanisms are better understood, problems with infertility that arise in the field may be more easily overcome. It will also enable a more prolific generation of embryos for research purposes, which ultimately will lead to further advances in many fields. Information generated from this research may be used to increase fertility of livestock species, decrease embryonic death, and ameliorate the declining fertility of older animals. There also will be more direct benefits for farmers who use assisted reproductive technologies such as superovulation, in vitro fertilization, and embryo transfer. These technologies increase reproductive rates of valuable females, even enabling generation of in vitro produced embryos from cows that are pregnant. These techniques also enable older, infertile females to continue to reproduce and provide products to market in the form of pregnant recipients and frozen embryos. The latter can be marketed easily internationally without a concern for transmitting diseases.
- Studies on nuclear transfer, transgenesis, and cryopreservation are important to the future of society. It is likely that the research on cell cycle control, conducted as a part of research on somatic cell nuclear transfer, may lead to cures for cancer, treatments to reduce the aging process, and methods to generate organs for transplant. When nuclear transfer is combined with transgenesis, even greater possibilities exist for both animal agriculture and biomedicine.
- Combining technologies such as in vitro fertilization, nuclear transfer, and cryopreservation provides phenomenal opportunities for increased efficiency of livestock production. Efficacious cryopreservation of oocytes enables storage of female gametes. Although embryos from ruminant species can be frozen reasonably well, the ability to cryopreserve oocytes will increase flexibility in animal production and in research. In vitro embryo production could be timed to use naturally cycling recipients. Oocytes from genetically elite females could be better utilized, leading to a faster rate of genetic improvement due to an increased female selection intensity. Cryopreservation of routinely collected embryos would provide many benefits to the livestock industry, such as not needing recipients in "real time" and being able to export genetic material.