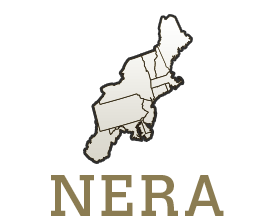
NE186: Genetic Maps of Aquaculture Species
(Multistate Research Project)
Status: Inactive/Terminating
NE186: Genetic Maps of Aquaculture Species
Duration: 01/01/1998 to 09/30/2002
Administrative Advisor(s):
NIFA Reps:
Non-Technical Summary
Statement of Issues and Justification
The ability to selective breed aquatic species would improve the quality and performance of these animals in commercial aquaculture. Genetic markers are important tools which allow the tracking of parentage and the performance of lines in breeding programs. The integration of these markers into linkage maps would further enhance their utility because it would allow more detailed analysis of the genetic variation affecting a trait. It would also facilitate the identification of the genetic alleles contributing to superior performance. Thus, the development of molecular markers and mapping of the genes determining economically important traits would speed the development of genetically improved strains. The enormity and complexity of this effort requires a collaborative effort among researchers with diverse disciplinary skills. Candidate species are: catfish, trout, tilapia, shrimp, and oyster.
Related, Current and Previous Work
Researchers are actively working to determine the complete sequence of several animal genomes as a toot for gene discovery and analysis of gene function (Hudson et al. 1995; Hodgkin et al. 1995; Rubin, 1996; Brenner et al, 1993). These efforts are expensive, and probably appropriate for only a handful of model systems. Fortunately, it is possible to exploit the sequences of these model systems to accelerate understanding of many closely related species, through the approach of comparative positional candidate gene analysis (Womack and Kata, 1995). Moderate density genetic maps have been developed for several species of agricultural importance (Barendse et al. 1997; Cheng, 1997; Rohrer et al. 1994). It is anticipated that identification of syntenic regions of the chromosomes will speed the identification of genes responsible for quantitative traits in the agricultural species. We recognize that for most aquaculture species there are still great gains to be made through traditional selective breeding. For many aquatic species, selective breeding programs have been difficult to implement because the juvenile forms are too small to be mechanically tagged, and so growth comparisons cannot be made in a common environment. The genetic markers we develop for mapping will find immediate application in tracking pedigrees in breeding programs. We are aimed at a longer term goal, to develop linkage maps which can be used to identify chromosomal regions, and ultimately the genes, responsible for quantitative variation. Our goal is to develop the tools required for accelerating breeding programs in the future. There are a series of logical steps that any genome project passes through. We take our lead from both the efforts of the human genome project, and our colleagues working on cows, sheep, pigs and chickens. The first steps are: 1. The development of a moderate density linkage map (1-2 cM spacing) using highly polymorphic DNA markers. 2. The mapping of genes controlling economically important quantitative traits using these markers. 3 The development of physical maps, linking of the physical map to the linkage map, and the identification of synteny with model organisms In the paragraphs below, we briefly review current technologies for these tasks. This is not meant to be an exhaustive list of current approaches. Furthermore, technologies for genome analysis are constantly changing and improving. We hope to take advantage of new approaches to linkage and physical mapping that may be developed over the next few years. Linkage mapping: Microsatellites (MS) - Tandem repeats of a short (1-6 bp) repeat of DNA are called micro satellites. These sequences are abundant in eucaryotic genomes, and tend to be highly polymorphic in length, because of variation in the number of tandem repeats (Tautz and Renz, 1984; Hudson et al, 1992). Their high level of polymorphism and ease of use have made them a preferred marker for linkage studies in many animal species (e.g. Dib et al. 1996; Dietrich et al., 1995). Amplified Fragment Length Polymorphisms (AFLPs) - Vos et al. (1996) described a technique for rapidly identifying anonymous fragment length polymorphisms. The basic technique involves digestion of genomic DNA with two restriction enzymes (e. a,. EcoRI and MseI) and ligation of specific adapters to the ends of the fragments. Successive rounds of PCR amplification with increasingly selective oligonucleotide primers reduces the number of observed fragments to a manageable number which can be scored on acrylamide gels. Quantitative trait mapping: Conceptually, quantitative trait mapping is simple. A hybrid cross is performed between two inbred lines differing in one or several traits of interest. The hybrid progeny are either intercrossed to one another, or backcrossed to one of the parental lines. The phenotypes of each F2 or BC progeny is recorded, along with their genotype for markers spaced at frequent intervals across the genome. At each locus a statistical test is performed to see if animals sorted by marker locus genotype differ significantly in phenotype. The presence of a quantitative trait locus (QTL) is suggested by rejection of the null hypothesis that the genotypic classes have identical phenotypes. Variations on this method are largely a search for greater statistical power. Simultaneous consideration of adjacent markers (interval mapping) can improve detection of QTL (Lander and Botstein, 1989). Composite interval mapping has been developed to control for the effects of unlinked QTL (Zeng, 1993, 1994). Maximum likelihood methods are popular, but require extensive computation and do not scale well when modelling multiple QTL for a given trait. Least squares methods may be more flexible in this regard (Haley and Knott, 199?; Jansen and Stam, 1994). Non-parametric methods have been developed to deal with traits which do not follow a normal distribution (Kruglyak and Lander, 1995). Another important new development is the candidate gene approach (Rothschild and Soller, 1997). Often genes known in other species can be reasonably hypothesized (on the basis of other knowledge on, for example, physiology or endocrinology) to affect a particular trait in the species under study. The effect of allelic variation at these so-called `candidate' genes on the quantitative trait can be directly studied. While this shortcut to gene identification is not without risk, experience has shown it has a relatively high probability of success. Physical mapping: Fluorescent in situ hybridization (FISH) - The direct hybridization of fluorescently-labelled DNA probes to chromosome preparations is an effective method for identifying the location and boundaries of synteny across species (Chowdhary et al. 1996; Ponce de Leon et al. 1996). FISH can also be used to physically map markers relative to one another, and to the ends of chromosomes (Smith et al. 1997). Radiation hybrids (RH) - This somatic cell method is an efficient route to physical mapping (Cox et al. 1990). Briefly, cells of the target species are irradiated to break the chromosomes into fragments 4-5Mb long. These cells are then fused to hamster cells, and a number of unique cells lines containing different combinations of target genome fragments are recovered. Presence/absence of particular genes in a panel of 100 such cell lines is used to determine the pattern and frequency of co-occurrence of markers, which is the basis of constructing a physical linkage map. An advantage of the RH approach is the ease with which type I markers can mapped to identify regions of synteny among genomes. Expressed sequence tags (EST) - An efficient approach to gene discovery and the characterization of sequence tagged sites (STS) is the partial sequencing of cDNA clones (Adams et al. 1993). The homology of ESTs among species can be readily assessed through sequence analysis, and PCR amplification can be used to map these markers in radiation hybrid panels (Banff et al., 1996; Foster et al., 1996). Bacterial artificial chromosome (BAC) libraries - Cloning vectors based on the E. coli Ffactor plasmid can stably maintain heterologous inserts as large as 300kb (Shizuyu et al. 1992). This system is being widely used in the analysis of both plant and animal genomes (Cai et al. 1995). These large cloned fragments are useful for physical mapping, and speed the construction of contigs (sets of overlapping clones) in the vicinity of mapped genes or QTL. Aquaculture species The prospects for genetic improvement of aquaculture species are good (Gjedrem, 1983; 1985) . Most species have large progenies, and short generation times, and both of these features allow strong selection to be imposed, accelerating progress in selective breeding programs. Still, progress in genetic improvement of these species has been slow. One reason is that reliable culture of the complete life cycle in captivity has only recently been achieved in some species. Second is the difficulty of physically marking offspring, and obtaining accurate measures of performance of juveniles. Finally, practical difficulties of hatchery management and product marketing have left growers little time to focus on breeding programs (Gall, 1991). The enormous potential of selective breeding in these species has yet to be realized. To date, genome mapping projects have focussed on mammals, and a few model organisms important to genetic research. As the techniques become more established and efficient, attention has begun to shift to additional species, particularly those of economic importance. These projects can be placed in a phylogenetic perspective It is interesting to note that the fish species to be studied are only slightly more divergent than the mammals. We may anticipate that these species will show large blocks of synteny with each other, and with emerging model systems such as the pufferfish (Brenner et al. 1993) and the zebrafish (Knapik et al. 1996). The aquaculture species also occupy an intermediate position on the tree, between the wellstudied genetic models of yeast, nematodes and Drosophila on the one hand, and chicken and mammals on the other. In this respect they are likely to be highly informative with respect to the evolution of genome structure and function. The status of current research on each species has been documented in the proceedings of a meeting held at U Mass/Dartmouth in May 1997 (Alcivar-Warren, 1997). A brief synopsis of progress toward genetic maps in each species is presented in the sections below. Catfish: Commercial production of catfish, Ictalurus punctatus is the largest single-species aquaculture enterprise in the United States. From 1980 to 1995, production grew from 46.5 million pounds to over 445 million pounds of fish processed (USDA, 1996). Commercial use of improved germplasm have dramatically increased production efficiency in many livestock species, and similar production increases should be possible through genetic improvement of catfish (Smith, 1991). Domestic catfish exhibit significant genetic variation and positive response to selection for economic traits such as growth, feed efficiency, disease resistance, carcass characteristics, environmental tolerance and reproduction (Dunham and Smitherman, 1987; Broussard and Stickney, 1981; Tomasso and Carmichael, 1991; Wolters and Johnson, 1995). Genetic linkage mapping in this species is at an early stage. The karyotype consists of 2N=58 chromosomes. Six linkage groups have been established using isozymes (Liu et al. 1992; Morizot et al. 1994), and several additional linkage groups have been identified recently in channel x blue(I. furcatus)hybrid crosses (Morizot et al, 1997). Recently, large numbers of polymorphic markers have been developed, including 400 randomly amplified polymorphic DNAs (RAPDs), 250 AFLP and 100 microsatellite markers (Liu, pets. comm., Waldbeiser and Bosworth, 1997), but are not yet mapped. Trout: The rainbow trout (Onchorhynchus my-kiss) is the leading salmonid species in U.S. aquaculture, and is the species with the largest biological database (Wolf and Rumsey, 1985), reflecting considerable study of the genetics, nutrition, immunology and physiology of this fish. Other species of 0?icorhynchus, including coho, Chinook and pink salmon, are also candidates for aquaculture. The Atlantic salmon (Salmo salary is the leading species for pen farming, and has been the object of considerable quantitative genetic study (Gjerde, 1993). The brook trout (Salvelinus fontinalis) is native to eastern North America and there is interest in culture of this species in the region. This species was the subject of early efforts to map protein coding genes (Johnson et al., 1987). Mapping of salmonids has focussed on allozyme polymorphisms, including the use of halftetrad analysis in meiotic gynogens (Thorgaard et al., 1983; Allendorf, 1986), which allows the mapping of genes with respect to the centromeres. Recent efforts have focussed on AFLPs (Thorgaard, pets. comet.) and the development of microsatellite markers (Olsen et al. 1996; Scribner et al. 1996). The karyotype of salmonids tends to be conserved for chromosome arm number (100104), but highly variable for chromosome number, which varies from 52 in pink salmon to 84 in brook trout. Atlantic salmon are distinct in having only 74 chromosome arms and a diploid number of 54-60. This distinctive karyotype suggests the linkage map for Atlantic salmon will be different than that for the other species. Because a well-funded effort to map the genome of Atlantic salmon already exists in Europe (the SALMAP collaboration), it is anticipated that efforts in the U.S. will focus on bow trout. These efforts should be complementary, as many of the genetic markers isolated in from one species can be incorporated in the map of the other. Tilapia: Tilapia (Oreochromis sp.) are a group of perch-like fishes (Family Cichlidae) native to the freshwaters of tropical Africa. They are one of the most important aquatic species in culture today. World-wide production of tilapia exceeds 1 billion pounds per year (ICLARM, 1991). U.S. production of tilapia is increasing rapidly, and was estimated at 19 million pounds in 1996. Businesses using recirculating aquaculture systems to grow fish in close proximity to local markets have received premium prices for live fish and/or fresh fillets. Demand for the product exceeds U. S. production however, and the live weight equivalent of 60 million pounds were imported in 1996 (American Tilapia Association, 1997). Imports are growing at a rapid rate, because the mild white flesh of tilapia is readily accepted by U.S. consumers. Until recently, only a few genes had been mapped in tilapia. Hussain et al. (1994) used meiotic gynogens (diploid progeny produced by suppression of the 2nd meiotic division) to map gene-centromere distances for six allozyme loci and two color loci. Approximately 170 microsatellite loci have been characterized in tilapia (Lee and Kocher, 1996) and related cichlids. Approximately 200 cDNA clones from a tilapia brain cDNA library have been sequenced at Dalhousie University (J. Wright, pers. comet.). A number of sequences homologous to known genes were obtained, including several ribosomal proteins, a G-protein, fatty acid binding protein and collagens. The first relatively complete genetic map for a tilapia (Oreochromis niloticus) was recently completed (Kocher et al., 1997). The segregation of 62 Microsatellite and 112 AFLP polymorphisms was studied in 41 haploid embryos derived from a single female. Linkages were identified among 162 (93.1%) of these markers. 95% of the microsatellites and 92% of the AFLP polymorphisms were linked in the final map, which spans 704 Kosambi centimorgans in 30 linkage groups covering the 22 chromosomes of this species. Shrimp: Penaeids are the most economically important species of marine shrimp. The Pacific white shrimp, Penaeus vannamei, is the leading species fanned in the western hemisphere and will be the focus of our research. Other important species in the region are P. stylirostris, P. californiensis, P. setiferus, P. duorarum, P. a: tears and P. paulensis. In 1996, world-wide production of shrimp was estimated at 2.8 million metric tons, 25% of which was farmed. Future increases are expected to achieved through farming. Since the early 1980s, the shrimp fanning industry has been seriously affected by disease, including the Taura syndrome, White spot and Yellow head viruses. Taura syndrome virus (TSV) is the most important disease of P. vannamei, causing mortality in excess of 70% (Lightner et al. 1995; Brock, 1995). The susceptibility to TSV and other viruses is genetically determined (Alcivar-Warren et al. 1997a,b; Dhar et al. 1997). A gene map could be used to accelerate the rate of genetic improvement in cultured shrimp through marker-assisted selection. A few microsatellites and type I markers have been characterized from P. vannamei. Approximately 250 microsatellites have been isolated from P. monodon (Vanavichit, pers. comet.). A partial linkage map of the P. japonicas genome has been produced using AFLP markers (S. Moore, pers. comet.). Efforts to develop cytogenetic maps must contend with the large number of small chromosomes in these species (2n=88-92). Mapping efforts in P. vannamei can build on the large pedigrees developed in the US Marine Shrimp Farming Program. Oyster: The U. S. oyster industry relies on two closely related species: the Pacific oyster (Crassostrea gigas) and the eastern oyster (G virginica). Breeding efforts on the Pacific oyster have focussed on improving growth rate. Two protozoan parasites, MSX and Dermo, have decimated the eastern oyster industry. A primary research goal is the discovery of genetic markers for disease resistance which could be incorporated into a breeding program for this species. Foltz (1986) documented Mendelian inheritance of 11 allozyme markers in C. virginica, and identified two linkage groups, containing 4 and 3 markers, respectively. In a study of genecentromere recombination rates in C. gigas meiotic gynogens, Guo and Gaffney (1993) found recombination rates as high as 100%, as would be expected if some loci are distal to a single region of chiasma formation on each chromosome. McGoldrick and Hedgecock (1997) studied segregation at 14 allozyme loci in C. gigas and found that segregation ratios often deviated significantly from expectation. Segregation distortion is a dominant feature of genetic studies of this organism, presumably because of strong selection during early life stages. McGoldrick (1997) studied the segregation of 30 microsatellite markers in two C. gigas families. A number of reference families have been developed by individual investigators. An informal international collaborative effort to develop linkage maps for the commercially important cupped oysters Crassostrea gigas and C. virginica has recently begun. Pedigreed reference families from both species are being used to examine the inheritance of both protein polymorphisms and nuclear DNA markers.
Objectives
-
To develop moderate density genetic linkage maps for important aquaculture species.
-
To map genes for economically important traits in each species.
-
To integrate maps of individual species, and relate these maps to those of other organisms.
-
Methods
Objective l: Develop genetic linkage maps Step I: Identification and development of Type II markers. A few hundred highly polymorphic markers, such as microsatellite DNAs or AFLPs, are needed for each species. This is a large effort for an individual lab, but is fortunately a task which is easily distributed. We anticipate that a single library enriched for microsatellite sequences will be developed for each species. This library will be shared among laboratories for the sequencing of individual clones and characterization of the microsatellite sequences. Step 2: Development and distribution of reference family DNAs. Concentration of genotyping on a few reference families is the most efficient way to develop a map. For each species, 1-2 reference families containing approximately 500 individuals will be developed. DNA will be extracted from each animal, and aliquots of these DNAs distributed to cooperating laboratories for genotyping of particular markers. Step 3: Genotyping of reference families. Genotyping can be efficiently conducted in a distributed fashion among laboratories. Because of the decentralized nature of regional project funding, we expect that no single laboratory will be able to synthesize the oligonucleotide primers needed to genotype all of the loci. We anticipate that individual labs will characterize a particular set of microsatellite loci, and will contribute genotypes of the reference family to the common database. The steering committee for each species will coordinate this work to avoid duplication. Step -l: Development of databases for the mapping data. An important part of this project will be the integration of results from individual laboratories. This will be a primary responsibility of the species facilitators and the Technical committee. Management of genotype information for the reference families is relatively simple and can be accomplished in spreadsheets. As the data structures become more complex (multiple reference families, alternative mapping techniques), a more flexible database system will be required. It is anticipated that existing relational database interfaces (e.g. the Roslin Institute's Arkdb) can be used to organize and distribute results for the aquaculture species (A. Archibald, pers. comm.). Development of catfish molecular markers will occur at Auburn, Louisiana, Mississippi and Texas for catfish, California, Montana, Washington State, and Maine for trout, and New Hampshire, California, Connecticut, Maine, Virginia Polytechnic, Xavier and Swansea for tilapia. Shrimp markers will be developed and mapped at Tufts, Connecticut, and Worcester Polytechnic. Oyster markers will be developed and mapped by Delaware, Rhode Island, Rutgers, Virginia Institute for Marine Science, Louisiana, California, Philadelphia College of Pharmacy and Keio University School of Medicine. Reference families for catfish are under development at Auburn and ARS-Stoneville. Trout families have been developed at Washington State, and tilapia families ae being raised in Scotland and California. Shrimp families have been developed by Tufts and the Oceanic Institute. Oyster families have been developed by California and Rutgers. Objective 2: Mapping of quantitative trait loci Step I: Development of phenotypic assays for traits of economic importance. Perhaps the most critical step in any study which aims to map quantitative traits is the development of efficient protocols for characterizing the phenotype. This is particularly true for aquaculture species, because environmental variation is often difficult to control. The first step toward mapping QTL is the demonstration of an accurate and repeatable measure of the trait under study. Traits to be studied will vary among species, but will typically include growth rate and disease resistance. Step 2: Rearing and scoring of appropriate Fn and backcross families segregating for OTL. The development of appropriate family material is the second crucial step in mapping QTL. Depending on the trait, either an intercross or backcross design will be most efficient. Many molecular laboratories will not be equipped to develop such family material, which requires specialized facilities, and frequently involves close collaboration with industry. Step 3: Genotyping of these progenies for type II markers and linkage mapping of O TL. The final phase of QTL mapping is the genotyping of progeny for a set of markers evenly spaced at roughly 20cM intervals. These genotypes are most efficiently obtained using semi-automated techniques in molecular laboratories dedicated to such studies. Quantitative genetic studies of catfish will be conducted at Auburn and Mississippi, of trout at Western Carolina, Montana and Washington State, and of tilapia at California, Virginia Polytech, and New Hampshire. Quantitative genetics of shrimp will be studied at Tufts, Mississippi, The Oceanic Institute, and of oyster at Delaware, Rutgers, Virginia Institute for Marine Science, Louisiana and California. Objective 3: Comparative genome mapping Step l: Development of physical maps. Physical maps are the most efficient approach to developing the comparative mapping information used in positional candidate gene approaches. Using the technology of radiation hybrids, and FISH mapping, we will begin to relate the genetic and physical maps. The focus in this stage of the research would be on Type I markers (coding genes), for which homologies across species are easier to identify. The set of evolutionarily conserved, functional genes identified by O'Brien et al. (1993) should be especially useful in this regard. Lyons et al. (1997) describe primer sequences for 410 loci useful for such comparative mapping in mammals, and extension of this method to aquaculture species will facilitate construction of synteny maps among our more distantly related species. Step 2: Identification of synteny. Relating the genetic and physical maps of these species to each other, and to those of model organisms, will allow the rich information in genome sequence databases to be brought to bear on the identification of candidate genes for particular QTL (Rothschild and Soller, 1997). In this stage of the research, alignment of the aquaculture species linkage maps will be attempted, and syntenies with model systems will be identified. Step 3: Develop large-insert libraries. Libraries of large-insert clones (e.g. BACs) area resource for physical mapping and positional cloning. An initial goal is the development of libraries which provide 95% coverage of the genome. This requires libraries which contain approximately three haploid equivalents. Since most of these aquaculture species have genomes approximately Ipg in size, a library of 20,000 clones should provide 3-fold coverage. Step -l: Probe these libraries for previously characterized markers. To begin the process of relating clones to the physical and genetic maps, the clones will be analyzed for the presence of STS markers. Briefly, the BAC library will be arrayed in microtiter plates and screened by PCR of the row and column DNA pools. The clone address of each positively amplified BAC will be confirmed by a second amplification. Comparative mapping of catfish will be conducted at Auburn, Louisiana and Texas, of trout at Wisconsin (Milwaukee), Washington State and Montana, and of tilapia at New Hampshire and Institute of Aquaculture (Scotland). Comparative mapping of shrimp will be conducted at Tufts and of oyster at Rutgers, Louisiana, California and Keio University.Measurement of Progress and Results
Outputs
- Develop moderate density linkage maps of highly polymorphic DNA markers for commercially important aquaculture species.
- Use linkage maps to identify and select genes controlling commercially important traits.
- Identify syntenic relationships with species which are being completely sequenced (e.g., the human genome project).
- Develop computer-mediated DNA sequence databases for identifying promising candidate genes.
- In the longer term, develop genetically superior strains for commercial production.
Outcomes or Projected Impacts
- Improved genetic markers for managing aquaculture broodstock.
- Improved breeding programs to enhance production traits.
- Development of faster growing, disease resistant strains of major aquaculture species through marker assisted selection.
- Elucidation of genetic mechanisms underlying disease resistance, and improved animal growth.
- For the consumer, a more plentiful supply of high quality seafood produced using sustainable production technologies.
- For the environment, reduced fishing pressure on key aquaculture species.