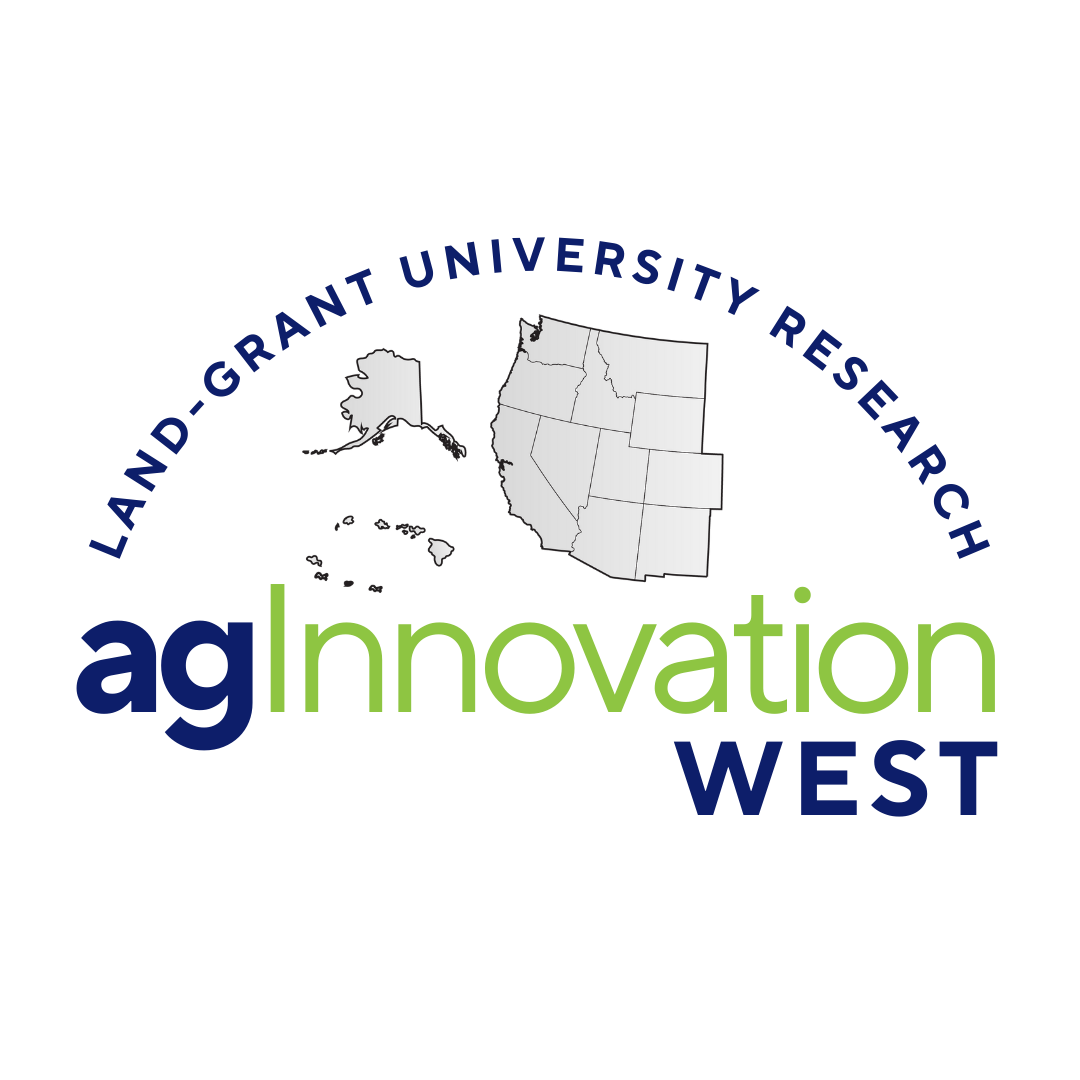
W_TEMP_4010: Integrated Approach to Enhance Efficiency of Feed Utilization in Beef Production Systems
(Multistate Research Project)
Status: Draft Project
W_TEMP_4010: Integrated Approach to Enhance Efficiency of Feed Utilization in Beef Production Systems
Duration: 10/01/2025 to 09/30/2030
Administrative Advisor(s):
NIFA Reps:
Non-Technical Summary
Beef cattle are well adapted to upcycle low quality feedstuffs into high quality protein for human consumption. However, the efficiency with which they convert that feed could be improved both for the benefit of the producer and the sustainability of the industry. The goals of this five-year project are to: to better understand biological sources of variation in the efficiency, to discover markers for the genetic improvement of nutrient utilization efficiency, to evaluate life-cycle efficiency to improve economic/environmental sustainability, to develop and decision-support tools to facilitate selection for improved nutrient utilization efficiency and to develop producer educational programs to enhance technology adoption by the beef industry. Stakeholders include other animal scientists that will benefit from the enhanced understanding of efficiency, industry professionals that will be able to incorporate new understanding into recommendations for producers and policymakers and primary producers that will have greater insight and tools to improve their operational efficiency.
Statement of Issues and Justification
The overall goal of this cooperative, multi-state, multidisciplinary, research and outreach project is to improve beef cattle nutrient utilization efficiency through a more complete understanding of the biological mechanisms that contribute to its variation. The scope of this project includes studies of molecular and systems physiology, genetics/genomics, nutritional and behavioral interactions that drive variation in nutrient utilization efficiency measured in terms of both Gain: Feed (G: F) and residual feed intake (RFI). With a strong integrated outreach program, this regional project will coordinate efforts across the nation to provide leadership and interact with industry to cooperatively disseminate this important information to US beef cattle producers.
This proposal to replace the soon to expire W-3010 project describes the collaborative effort of research from Agricultural Experiment Stations across the country to characterize specific aspects of the molecular, physiological, and genomic mechanisms that regulate beef cattle nutrient utilization efficiency. The proposed project addresses a major agricultural need. Improving the efficiency of meat production in beef cattle is essential to meet consumer demands for high-quality products, ensure profitability and minimize natural resource use.
In an era of increasing costs of feedstuffs and less available land for food production, the importance of finding new ways to maintain production with reduced demand for feed has a direct effect on rural prosperity and contributes to the sustainability of the beef industry. Reducing the demand for feedstuffs for similar production levels will also facilitate the distribution of feedstuffs for broader utilization. These technologies are readily transferable to beef production systems throughout the world and thus have the potential to contribute to both national and global food security. Realized reductions to the costs of producing beef translated to more competitively priced beef products in the global market.
- National importance of building this multi-state project: Across the nation, individual and outreach efforts with nutrient utilization efficiency are expanding at a rapid rate. Industry at multiple levels is embracing feed and nutrient utilization efficiency as a priority for research and development. In addition, the synergy that will be developed across this team of researchers will convert projects with local or regional impact to having a national and international impact.
- All projects outlined in the following methods section are technically sound and are in different stages of progress. Many of these have been peer-reviewed and are presently contributing impact at the state, regional, or local levels.
- This project is both multi-state and a multidisciplinary effort. It involves investigators with broad expertise across the nation and international collaborators in Australia and Canada. The Principal Investigators represent a variety of science disciplines and outreach expertise that complement each other and provide the skill-sets necessary to complete the objectives.
- The project involves strong cooperative efforts between the various units including the exchange of reagents, genomic data, a feed efficiency phenotype database as well as the exchange of knowledge and techniques, joint use of equipment and techniques available at particular experiment stations and joint publication of research results. A number of collaborative projects are described in the procedures for this proposal. The committee feels that as we are reaching out to encourage broader participation this will only increase. In addition, without a funded regional project, the collaborations would be substantially more difficult to initiate and maintain.
- The members of this committee have been successful in obtaining outside support to fund research. Funding from the NIFA and other granting agencies, foundations, and industry sources has been needed to carry out the work and to maintain the high level of productivity demonstrated by the previous group and this record of outside funding is expected to continue.
- This proposal describes a research and outreach approach to an increasingly important agricultural problem. Both the members of the previous project and the projected members of this project have demonstrated a high level of productivity and this is expected to continue with the new project.
- The impacts from the completion of this multi-state project will be national and international in scope. This project will contribute scientific rigor in elucidating the molecular mechanisms that underpin variation in beef cattle nutrient utilization efficiency and contribute to the development of biologically based predictors and improve the accuracy and reliability of mathematical models to predict feed intake and nutrient use in beef cattle, including grazing beef cattle. Producers will benefit from the development of molecular technologies that enhance conventional EPD estimates. The developed technologies will add value to conventional phenotyping for feed efficiency which is costly and time-consuming. Thus, the technologies are expected to lead to a long-term reduction in costs of overall FE testing and improve the nutrient utilization of beef cattle resulting in a lower impact on the environment and reducing costs to the producer for similar levels of beef production.
- The W-3010 Committee has been highly productive. Over 100 papers, book chapters/theses and abstracts have resulted from years 2020-2024 of the previous five-year project, and additional publications are expected through the 2025 termination date. In addition, substantial progress has been made under each of the specific objectives of the previous project.
Related, Current and Previous Work
Participating stations in this collaborative agreement are situated across several geographic regions, each with unique management challenges for optimizing livestock production in both feedlot and pasture environments. To address this, we employ a multidisciplinary research approach to enhance feed efficiency. This encompasses investigations into key areas such as feed intake and behavior, digestive physiology, fetal programming, genetics, microbiology, and precision livestock management.
Research conducted by the W4010 team builds upon a fundamental principle: applied research in animal agriculture originates from a robust foundation of prior basic research. Our work focuses on identifying the mechanisms that control feed efficiency in beef cattle and subsequently translating these discoveries into practical strategies for enhancing animal productivity. The collaborative efforts of the W4010 team have resulted in a substantial body of research achievements, evidenced by numerous publications and successful student theses and dissertations.
Animal agriculture faces significant challenges, with feed costs accounting for 50-70% of variable production expenses (Arthur and Herd, 2005). As a result, the industry has historically prioritized enhancing feed utilization efficiency (Ensminger, 1990). Various metrics for feed utilization have evolved over time, typically integrating total feed consumed with productivity indicators such as growth rates, offspring weight, and milk production (Koch et al., 1963; Koknaroglu et al., 2005; Ahlberg, 2017). In controlled environments like feedlots, average feed efficiency is calculated based on the total feed delivered to a group of animals. These data based on groups of animals, effectively drives improvements in sectors with shorter generation intervals, such as poultry and swine. However, for ruminant livestock (cattle, sheep, goats, etc.) with longer generation intervals and segmented production, producers often evaluate only specific phases of production (e.g., backgrounding, finishing), limiting their ability to assess overall feed efficiency.
Historically, the primary focus for improving feed efficiency has been on reducing input costs per unit of product or increasing growth and outputs per unit of input (Rowan, 2022). Recently, however, the animal agriculture industry has expanded its focus to include sustainability, aiming to reduce its carbon, water, and ecological footprints. These environmental footprints are largely driven by feed efficiency. For example, the beef industry has reduced its water footprint by 37% over the past 30 years by improving feed efficiency (Klopatek and Oltjen, 2022). Notably, only about 5% of the U.S. beef industry's water footprint is due to water consumed by the animals; 93% is related to water used for irrigating feedstuffs. A 5% improvement in water use efficiency across the U.S. beef herd would equate to roughly six weeks' worth of the water that flows over Niagara Falls.
Selection for more feed efficient cattle and stakeholder adoption of technologies to enhance genetic merit for feed efficiency is an effective strategy to meet future demands for animal protein in a sustainable manner. Feed efficiency is a complex trait regulated by numerous genes and biological processes (Cantalapiedra-Hijar et al., 2018). In a review of studies that examined the biological determinants of RFI in beef cattle, Herd and Arthur (2009) concluded that approximately one-third of the variance in RFI of beef calves could be explained by inter-animal differences in digestion, heat increment, composition of gain, activity and feeding behavior, and posited that the remaining two-thirds of variation in RFI may be linked to inter-animal differences in energy expenditures associated with biological processes like protein turnover, ion pumping and efficiency of mitochondria biogenesis. Characterizing the biological determinants associated with the variation in the efficiency of nutrient utilization provides insight into the regulatory mechanisms controlling feed efficiency and provides opportunities to identify candidate biomarkers associated with animals exhibiting more favorable feed efficiency phenotypes.
Feed intake and feeding behavior:
Feed intake is regulated by multiple complex physical and biological mechanisms that are not completely understood; however, consumption of feed is inextricably associated with feeding behavior (Allen, 2014). Previous studies have demonstrated a strong association between feed efficiency, measured as RFI, and feeding behavior traits (Lancaster et al., 2009; Hafla et al., 2013; Fitzsimons et al., 2014). Parsons et al. (2020) characterized feeding behavior patterns in steers consuming a high concentrate diet and found that low-RFI steers consumed 16% less dry matter and had fewer and shorter bunk visit and meal events than high-RFI steers. Additionally, low-RFI steers had less day-to-day variation in DMI and feeding behavior traits than high RFI steers, which may contribute to a more favorable rumen environment and greater efficiency of feed utilization. These authors reported that these differences in feeding behavior traits were minimally affected by covariate adjustment for DMI, indicating that steers with divergent RFI had distinctive feeding behavior patterns that were largely independent of differences in DMI (Parsons et al., 2020). Inter-animal variation in energy expenditure on feeding activity may explain a portion of the variation in feed efficiency. Understanding the relationship between feeding behavior patterns and feed efficiency metrics provides opportunities to identify indicators of complex mechanisms controlling the efficiency of feed utilization and offers potential biomarkers for selection of cattle with more favorable feed efficiency phenotypes.
Enteric methane emissions:
Use of dietary energy is the primary factor influencing overall efficiency of feed use (Ferrel and Jenkins, 1985; Trenkle et al., 1977; Rolfe et al., 1997). Matching energy supply to demand in livestock is key for achieving cost-efficient production systems. This is particularly the case in beef cattle where maintenance energy expenditure for the cow herd is one of the largest costs incurred for beef cattle producers and is also the largest single contributor to methane emissions related to beef production. Further, the beef cow herd (cows, replacement heifers, and bulls), which relies heavily on grazing systems, has been shown to require 82 and 64% of total feed energy inputs in a calf-fed and yearling-fed beef production systems, respectively (Basarab et al., 2012). Additionally, in calf-fed and yearling-fed beef production systems, the cow herd is responsible for about 85 and 70% of GHG emissions, respectively (Beauchemin et al., 2010; Basarab et al., 2013) Although most GHG mitigation strategies are currently focus on confined cattle, there is a need to assess mitigation strategies for grazing cattle.
Thus, optimizing dietary formulation to meet nutritional requirements has the potential to reduce production costs and the environmental footprint without compromising productivity. Wehrbein et al (2024; 2025) demonstrated that limit-feeding high concentrate diets (HCON) from 50 days pre-calving up to 84 days post-calving resulted in lower methane emissions and lower water intake compared to traditional high forage winter-feeding systems. Suggesting that HCON could minimize the carbon footprint and improve water usage efficiency of cow-calf systems. Further, winter-feeding HCON diets resulted in greater milk production, which may positively impact offspring performance.
Strategies that improve the efficiency of feed utilization will increase the economic viability of beef production systems, and reduce nutrient excretions (Waghorn and Hegarty, 2011). Multiple studies have demonstrated that more feed-efficient cattle consume less feed and have decreased total methane emissions and methane intensity than feed inefficient cattle (Hegarty et al., 2007; Fitzsimons et al., 2013; Manafiazer et al., 2020; O’Reilly et al., 2024). Hegarty et al. (2007) reported that beef steers selected to be more feed efficient produced 25% less methane than their inefficient counterparts. Moreover, several studies have demonstrated that heifers with low RFI as heifers, subsequently consumed 15 to 20% less hay as pregnant or lactating cows compared to cows that had high RFI as heifers (Black et al., 2013; Hafla et al., 2013). Additionally, studies have shown that cattle with low RFI phenotypes had more efficient grazing behavior patterns (Gregorini et al., 2015) and consumed less forage when grazing (Manafiazar et al., 2015; Manafiazar et al., 2020) and were better adapted to hot grazing conditions (Sprinkle et al., 2021) than cattle with high RFI phenotypes. While feeding management strategies and the selection of feed-efficient animals have shown promise in reducing methane emissions and enhancing environmental sustainability, the current rate of adoption by US producers remains limited.
Microbial biomarkers:
Identifying microbial biomarkers in beef cattle is crucial for advancing feed efficiency and sustainability in beef production. The rumen microbiome plays a central role in nutrient digestion and energy production, directly influencing feed efficiency phenotypes (Myer et al., 2015) . Such biomarkers can be used to design precision feeding programs, select for favorable host-microbiome interactions through breeding, and develop probiotics or dietary interventions to enhance nutrient utilization. By improving feed efficiency, these advancements can reduce feed costs and environmental impacts, such as methane emissions, while supporting sustainable beef production systems to meet growing global protein demands.
Current research highlights the potential of rumen microbial biomarkers, such as Flavobacteriia and pantothenate, in predicting feed efficiency traits like RFI in beef cattle. Notable correlations between pantothenate and feed efficiency were identified, linking, and predicting, the functional capacity of the rumen and its microbiome, specifically Flavobacteriia, through serum pantothenate offering the potential to use serum biochemistry as an indicator in identification of feed efficient cattle (Clemmons et al., 2019). Studies suggest that the relative abundance of specific microbial taxa and metabolic pathways, including those related to pantothenate biosynthesis, can serve as predictive markers of the microbial community structure and host feed efficiency. Indeed, ruminally supplementing microbes identified as important to feed efficiency traits, such as those contributing to optimizing the rumen microbial interactions (e.g., competition and cooperation) and those that could reduce the production of lactic acid and dCO2 to stabilize rumen pH, resulted in an improved average daily gain (ADG) of 29% and a tendency toward a 19% decrease in the feed efficiency metric, gain to feed ratio (G:F) Yang et al., 2025). Through discovery and by integrating these biomarkers into predictive models, producers can develop microbiome-driven selection tools and dietary interventions to enhance feed efficiency, reducing production costs and environmental impacts in the beef industry.
Fetal programming:
Evidence suggests that biological processes regulating normal growth, development, and nutrient utilization are programmed in utero, even during the earliest developmental stages (Caton et al., 2019; Caton et al., 2020). A research model developed at the North Dakota State University in collaboration with South Dakota State University and University of Auburn, examined the impacts of maternal vitamin and mineral supplementation (VTM, supplemented vs. NoVTM, not supplemented) during the periconceptual period or throughout gestation in beef heifers. A metabolomic analysis revealed that metabolites in the oxidative phosphorylation pathway were more abundant in the liver of fetuses from VTM than NoVTM dams (Crouse et al., 2022), suggesting that a greater supply of micronutrients during the periconceptual period and first trimester of pregnancy may positively modulate mitochondrial energy metabolism in offspring. These changes in the abundance of metabolites suggested physiological adaptations to meet fetal metabolic needs. Further, high-resolution respirometry analysis revealed greater efficiency of energy utilization in small intestinal samples of neonatal calves from VTM dams (Menezes et al., 2022). In fact, VTM offspring were heavier from weaning to breeding phase than NoVTM offspring, suggesting that maternal nutrition affects physiological mechanisms in utero that modulate offspring energetics and efficiency of nutrient utilization in the postnatal period.
Digestive physiology:
A functional gastrointestinal tract is crucial for nutrient absorption to supply energy and nutrients to the body. Also, the gastrointestinal tract and associated tissues, such as the liver and pancreas, utilize a disproportionate amount of energy compared to other tissues such as skeletal muscle (Rolfe et al., 1997; Caton et al., 2000; Swanson et al., 2008). Therefore, large improvements in energetic efficiency could be realized by developing approaches to reduce energy needs of the gastrointestinal tract and associated tissues.
One biological mechanism proposed to contribute to the inter-animal variation in feed efficiency is digestibility, with low RFI cattle exhibiting an improved digestibility pattern and subsequent increased nutrient utilization compared to high RFI cattle (Richardson and Herd, 2004). Studies have reported higher digestibilities in low- compared to high- RFI cattle (Johnson et al., 2019), while others have reported no differences in digestibility between cattle with divergent RFI (Fitzsimons et al., 2014). Results from a meta-analysis of literature studies revealed that the favorable dry matter digestibility in low-RFI cattle may actually be the consequence of DMI being negatively correlated with DM digestibility (Cantalapiedra-Hijar et al., 2018). However, Johnson et al. (2019), who reported that low RFI cattle had improved dry matter and nutrient digestibilities, found that DMI was not a significant covariate for dry matter digestibility, indicating that the reduction in dry matter digestibility of high-RFI animals was at least partially independent of DMI. Together, digestibility and fermentation account for approximately 18% of the inter-animal variation between cattle with divergent RFI (McCann et al., 2014), indicating the need to investigate the association between digestibility, rumen fermentation, and the rumen microbiota with cattle demonstrating more favorable feed efficiency phenotypes.
Genetic biomarkers:
There is a complex relationship among host genetics, rumen microbiome, and metabolome that drives variation in complex, polygenic traits. Including the effects of the microbiome and metabolome should improve phenotypic predictions, particularly for complex traits. Improving accuracy of phenotypic predictions will aid current strategies for genomic selection.
Several studies now support evidence of low-to-moderate host genetic control over rumen microbial features (Difford et al., 2018; Zhang et al., 2019; Sasson et al., 2017; Wallace et al., 2019; Abbas et al., 2020; Li et al., 2019). Across studies, host genetics on average appear to influence approximately 20% of heritable microbial feature variation, with some studies suggesting universal heritability of core microbial communities (Wang et al., 2024). While previously reported heritability estimates suggest reasonable control over the rumen microbiome, only a small fraction of the total rumen microbial composition appears to be heritable. However, heritable rumen microbial features are central members of co-occurrence networks (Sasson et al., 2017; Wallace et al., 2019, Li et al., 2019) and strongly correlate phenotypic traits such lactation performance (Zang et al., 2022), feed efficiency (Li et al., 2019), and methane production in comparison to their nonheritable counterparts. Heritable microbial communities contributed to 16 to 33% of variation in lactation traits in dairy cattle (Zang et al., 2022) and were strongly host genomically correlated to growth traits in beef cattle (Martínez-Álvaro et al., 2024). Highly heritable taxa contribute greater influence in metabolism in comparison to lowly heritable microbial communities (Sasson et al., 2017). Therefore, identifying heritable rumen microbial communities could reveal host-microbiome interactions underlying energy availability. Indeed, current work has also demonstrated using Angus steers (n = 343) enrolled in feed efficiency trials, out of the 532 bacterial and archaeal communities, 35 rumen bacteria were heritable (mean h2 = 0.24 ± 0.13). Heritable microbial communities displayed high connectivity in co-occurrence networks and contributed more to feed efficiency traits than their nonheritable counterparts (Henniger et al., 2025).
Multi-omics datasets have been proposed for integration into genomic predictions to improve selection for complex traits (Ross et al., 2013; Martinez-Alvaro et al., 2024; Xue et al., 2020). The inclusion of -omics data responds similarly to the effect of including correlated traits, where the accuracy of predictions is reliant on the heritability of the feature/trait and the magnitude of relationship between the feature/trait and phenotype (Christensen et al., 2021). As the rumen microbiome is demonstrated to be under low-to-moderate host genetic control and strongly genomically correlated to production-relevant traits in cattle, the rumen microbiome may be an informative feature to fit in genomic models. Therefore, rumen microbial features have been implemented across several studies in cattle with varying degrees of predictive ability (Martínez-Álvaro et al., 2022; Martínez-Álvaro et al., 2024). Current work has also demonstrated the holobiont effect, otherwise described as holobiability, to determine the host-microbiome influence on feed efficiency phenotypic traits and prediction (Henniger et al., 2025). To estimate heritability, microbiability, and holobiability of feed efficiency traits, variance components were calculated from a Bayesian reproducing kernel Hilbert space model where kernels were either the genomic effect, genomic and microbiome effects, or the genomic, microbiome, and genome-by-microbiome interaction effects. Holobiability was always greater than the heritability or direct heritability estimates, indicating the duality of host genetics and the rumen microbiome on feed efficiency (Henniger et al., 2025). Fitting the microbial effect improved the predictive ability of each model. Incorporating microbiome data into prediction models has the potential to improve predictive ability for complex traits.
Metabolomics has been used to discover novel metabolic pathways responsible for variation in growth and fat deposition in cattle (Weikard et al., 2010), and for discovery of biomarkers predictive of economically relevant traits in pigs (Rohart et al., 2012) and cattle (Karisa et al., 2014). Utilizing both targeted and non-targeted metabolomic approaches across various analytical platforms, studies have probed the associations of metabolites with RFI in beef cattle to identify metabolic networks regulating the biological pathways controlling this economically important phenotype (Karisa et al., 2014; Clemmons et al., 2017; Consolo et al., 2021). Karisa et al. (2014) examined plasma metabolites associations with RFI in 2 populations of growing cattle. The variance in RFI explained by metabolite profiles ranged from 36 to 98%, which was influenced by population and day of test that blood was sampled. Moreover, recent studies have demonstrated the potential of metabolomics to identify blood metabolite profiles as predictive biomarkers of feed efficiency in livestock (Foroutan et al., 2020; Goldansaz et al., 2020). There exists a continued need to identify metabolic mechanisms associated with inter-animal variation in RFI to prompt discovery of metabolite indicators that would enable accurate and more cost-effective prediction of feed-efficient animals early in life.
Precision livestock:
The ongoing pursuit of improved efficiency has generated significant interest in developing accurate methods to evaluate individual feed intake in ruminants. Innovations in the early 2000s, such as GrowSafe (now Vytelle), C-Lock, Insentec, and Intergado (Basarab et al., 2003; Kolath et al., 2007), focused on continuously weighing feed disappearance from feed bunks that allow only one animal to feed at a time, using unique Radio Frequency ID tags for identification. Recent advancements have integrated automated weighing systems for continuous monitoring of individual animal body weight and water intake.
Despite these technological advancements, current automated intake systems face two major limitations: (a) their reliance on delivered feed, which restricts their use to controlled feeding facilities, and (b) the limited number of systems available. For instance, there are approximately 21,000 Vytelle bunks globally, each accommodating only eight animals, which means only about 600,000 animals are evaluated annually. Given that the U.S. beef cattle inventory exceeds 87 million (USDA NASS, 2024), this represents less than 1% of the population. Additionally, with only 11 million animals in feedlots (~12.6% of the U.S. beef herd), the majority of cattle are either grazing or consuming harvested forage. Specifically, about 37 million cows graze on rangelands west of the 97th meridian and pastures to the east. The western rangelands account for 21% of the U.S. surface area (~162 million hectares), and eastern pastures cover 6% (~49 million hectares; USDA Climate Hubs, 2024). Currently, few methods exist to quantify feed and water intakes of individual animals in these grazing systems.
Most research on grazing cattle relies on estimation methods derived from predictive equations (NASEM, 2016), highlighting the urgent need to develop methods to more accurately monitor feed intake of grazing animals. Various methods have been used to estimate forage intake of grazing animals ranging from manual measurement of herbage disappearance to use of external markers, and animal-based sensor technologies. Grazing sensors equipped with accelerometers and GPS devices enable real-time geolocation data to assess total distance traveled per day and grazing behaviors including time spent grazing, ruminating and walking per day, with data used to generate predictive algorithms to estimate forage intake of individual grazing animals.
Such tools are essential for identifying efficient replacement animals within herds, which would enhance farm and ranch profitability. Assessing individual feed intake is also crucial for estimating the ecological footprints of livestock enterprises. Methane emissions from ruminants, primarily resulting from anaerobic fermentation processes in the forestomach, are a significant concern (Galyean and Hales, 2022). As the industry increasingly focuses on reducing its ecological impact, our technology could play a vital role in helping producers evaluate and verify Scope 3 greenhouse gas emissions, calculate water footprints, measure ecosystem service credits, and participate in related commerce. Methane output, for example, is directly linked to feed intake, particularly in grazing animals on high-forage diets (Galyean and Hales, 2024).
A search for overlap with other multistate activities revealed a few projects with distinctly different objectives to the present proposal: NC1181 Sustaining Forage-based Beef Cattle Production in a Bioenergy Environment; NC1182 Nitrogen Cycling, Loading, and Use Efficiency in Forage-Based Livestock Production Systems; NC1184 Molecular Mechanisms Regulating Skeletal Muscle Growth and Differentiation; NCCC210 Regulation of Adipose Tissue Accretion in Meat-Producing Animals; NRSP009 National Animal Nutrition Program; S1045 Genetic Considerations for Beef Cattle Production in Challenging Environments; SERA041 Beef Cattle Production Utilizing Forages in the Southeast to Integrate Research and Extension Programs across State Boundaries; W1012 Improving ruminant use of forages in sustainable production systems for the western U.S.; and WERA001 Beef Cattle Breeding in the Western Region. However, many of the objectives of these other projects are complementary to those of the present multi-state project proposal. Whenever appropriate, committee members will maintain communication with these groups through collaborative research efforts and attendance at meetings of these groups. In addition, The NCCC308 group focusing on the Nutrition and Management of Feedlot Cattle has an overlapping objective as they seek to enhance productive efficiency and quality of feedlot cattle. This proposed group is complementary as it aims to look at life-cycle efficiency rather than just relative to feedlot performance.
Objectives
-
To understand biological sources of variation in the efficiency of nutrient utilization in beef cattle.
-
To discover and develop biomarkers and genetic markers for the genetic improvement of nutrient utilization efficiency.
-
To evaluate life-cycle efficiency of nutrient utilization in beef cattle in both confined pens and pastoral settings to improve economic/environmental sustainability
-
To develop and propagate EPDs, selection indices, and decision-support tools to facilitate selection for improved nutrient utilization efficiency.
-
To develop producer educational programs to enhance technology adoption by the beef industry.
Methods
By working cooperatively across this multi-state project, scientists in the participating Experiment Stations will build synergy and will expand their focus from the regional and state levels to have a national and international impact.
To understand biological sources of variation in the efficiency of nutrient utilization in beef cattle. Studies under this objective will focus primarily on the identification of the sources and quantification of the magnitude of the biological drivers of variation in feed efficiency and nutrient utilization. The following Experiment Stations will work in collaboration on this objective: California, Colorado, Illinois, Kentucky, Missouri, Montana, Nebraska, South Dakota, Tennessee, and Texas. Previous research has shown that differences in feed and nutrient use efficiency are related to variations in multiple mechanisms, including variations in microbial ruminal fermentation patterns and digestibility, as well as variation in maintenance energy requirement, which in turn is related to rates of body protein turnover. Collaboratively, we will determine the range in RFI that exists within the beef population. In Particular, we will identify biological mechanisms of RFI variation, determine genetic and environmental impacts on RFI, and quantify waste output and methane emissions in relation to RFI. Current research is focusing on:
- Investigating how feeding behavior, digestion, and methane production differ in cattle with varying feed efficiency in both confined pens dry lot and pastoral settings(Texas)
- Identifying biological factors that cause variations in feed efficiency and nutrient use (South Dakota State; Nebraska)
- Examining how feed efficiency affects the response of beef steers to dietary supplements, with the aim of reducing antibiotic use (West Virginia)
- Using machine learning approaches to develop tools to quantify individual grazing DMI at scale, from which grazing RFI can be determined (West Virginia)
- Studying the rumen microbiome to understand the mechanisms of efficient feed utilization in beef cows (Tennessee; Nebraska; Texas)
- Analyzing gene expression, metabolites, and physiological parameters to understand fattening in feedlot steers and improve carcass quality. Additionally, investigating metabolic rate and gene expression in grazing cows across seasons (Montana State)
To discover and develop biomarkers and genetic markers for the genetic improvement of nutrient utilization efficiency. Establishment of well-characterized populations of beef cattle across member experiment station herds and collaborating industry herds will provide the resource for this objective. In addition, outcomes from Objective 1, understanding the biological drivers of variation in RFI will contribute knowledge of candidate mechanistic pathways and candidate genes for study of potential physiological biomarkers and gene markers for RFI. The Colorado, Missouri, Ohio, Montana, Nebraska, Tennessee, Texas, and Wyoming Experiment Stations will collaborate to identify genetic, microbial, physiological, and behavioral biomarkers for ranking RFI phenotypes of cattle. The Colorado, Missouri, Montana, Nebraska, Ohio and Texas Experiment Stations will lead the studies to examine associations between RFI, and blood metabolites, hormonal profiles, and feeding behavior metrics. Virginia Tech has ongoing work focused on developing low-cost, low-power sensors to monitor critical production parameters in pasture-based production systems. Tennssee also has continuing work through the UT Precision Livestock Farming program utilizing real-time monitoring of biological, physiological, and environmental parameters to assess and improve production in pasture-based systems. The long-term goal of this pasture-based work is to identify detectable behaviors, attributes, or trends of calves that will be reliable predictors of whole-system efficiency, defined as the cumulative feed input needed to raise the calf to harvest weight. This form of digital phenotyping can enable more targeted investigation of factors influencing nutrient utilization in beef cattle and facilitate a more targeted assessment of genetic markers for genetic improvement of nutrient utilization efficiency.
To evaluate life-cycle efficiency of nutrient utilization in beef cattle in both dry lot and pastoral settings to improve economic/environmental sustainability Studies under this objective will focus on a) measuring individual grazing DMI of grazing females to aid in selecting feed efficient replacement females and b) measuring postweaning feed efficiency in young female cattle and looking at the impact of feed efficiency measured in growing animals on feed efficiency measured later in life, productivity, and longevity. This data has been collected for the last six years at the Northern Agricultural Research Center within the Montana Agricultural Station and data collection will continue. The West Virginia Agricultural and Forestry Experiment Station has recently published a machine learning approach for predicting dry matter intake (DMI) using daily individual animal data and climatic conditions (Blake et al., 2023). The work focused on the initial group of bulls and steers utilizing the system. By employing gradient boosting models on the preliminary dataset, we developed a structured method to correct prediction errors through an ensemble of decision trees. Each tree refines the previous one, improving overall accuracy. Among the five boosting techniques evaluated, Gaussian Process Boosting (GPBoost) outperformed others regarding training-testing fit and prediction accuracy (ArunKumar et al., 2024). West Virginia has acquired three mobile IPW units for collecting daily individual body weight and water intake data essential for our DMI predictions. These units can operate in various grazing or dry lot systems and were successfully deployed at Purdue University, WVU – Reedsville, Ohio State University, and the University of Georgia from May to December 2024. Inert markers are used during deployments to obtain individual grazing DMI values. Additionally, multiple experimental stations involved in this project are measuring methane emissions and energy expenditure in beef cattle in both confined and pastoral settings using indirect respiration calorimetry, such as the GreenFeed system. Further, projects at South Dakota State University focus on measuring postweaning feed efficiency in young female cattle and examining how feed efficiency measured in growing animals impacts later-life feed efficiency, productivity, and longevity. Additionally, studies evaluating the relationship of feed efficiency measured in growing heifers with feeding behavior, supplement intake, and reproductive performance in the same cattle after maturity is ongoing at the Montana Agricultural Experiment Station. This work is ongoing and includes projects by established faculty in the Department of Animal and Range Sciences as well as our new Associate Professor. Dr. Thomson participates in the beef production research group and will represent this work to this multi-state committee. Virginia Tech has ongoing work leveraging cradle-to-farm-gate models of beef production systems to evaluate the effects of management decisions on carbon, water, and land footprints of beef production systems. These models can also be adjusted to identify optimal management practices to reduce these environmental footprints either individually or simultaneously. As economies shift and beef cattle feeding becomes increasingly more reactive to other industries (e.g., increased corn co-product feeding due to shifting ethanol and soy economies), these models enable efficient investigation of how management practices influence life-cycle nutrient utilization and related down-stream effects on economic viability and environmental impacts.
To develop and propagate EPDs, selection indices, and decision-support tools to facilitate selection for improved nutrient utilization efficiency. Studies under this objective will integrate molecular-level information in developing quantitative tools for the evaluation of FE and its implementation into the industry. A major objective of this project will be the integration of data from populations of beef cattle in the development of high accuracy EPDs for RFI and other production traits. Advanced concepts in mathematical modeling of RFI will also be studied under this objective. Specifically, the California and Texas Experiment Stations will evaluate and re-parameterize existing mechanistic nutrition models to explain more of the individual variation in nutrient requirements and intake to facilitate selection programs to identify animals that have divergent differences in the efficiency of feed utilization. Data from experiments conducted by participating Experiment Stations will be used to test and improve existing simulation models of cattle growth and body composition. Models will be modified by identifying and quantifying model parameters that reflect efficiency differences between animals, allowing estimation of system effects of selection or feeding animals of different efficiencies. Modeling analyses will also serve to evaluate hypotheses and extend the interpretation of results regarding RFI, and also as a tool for extending knowledge regarding RFI to the beef industry and the public at large. As the multi-state project database incorporating phenotypic and genotypic information is developed, new iterations of performance models will be developed to interface with this database. This will enable improvement of the existing models as well as the development of decision-aid tools to maximize the application of those data by industry
To develop producer educational programs to enhance technology adoption by the beef industry. To develop producer educational programs to enhance technology adoption by the beef industry. Faculty participating in this multi-state project have research, teaching and extension appointments. Outreach will be a strong thrust of our multi-state efforts. Participation of members in a BIF National Standards sub-committee for the development of feed efficiency testing protocols and interaction with state cattle associations and national breed societies will contribute to the integrative character of the project. This multi-state project will facilitate broader interaction of extension faculty bringing national impact to our outreach efforts involving California, Mississippi, Montana, Missouri, and Texas Experiment Stations.
Measurement of Progress and Results
Outputs
- Publications will be the primary tangible result expected from the project. The sharing of samples, DNA, and feed efficiency data will allow for additional publications and additional data derived from individual experiments.
- Extension publications derived from the primary scientific publications and other sources such as the project database will provide an important link that will lead to increased industry adoption nation-wide, and improved nutrient utilization efficiency in beef cattle.
- The Committee will sponsor a Symposium on Molecular Mechanisms underlying Nutrient Use Efficiency. This symposium will provide a mechanism to convey new research information and will increase the visibility of the project.
- Interaction of the committee with the seed-stock industry will enable development of multi-breed EPDs for feed and nutrient use efficiency.
- Increased integration and mechanisms for sharing of information between the scientific community engaged in the study of production efficiency and the beef industry sectors.
- Develop workshops and short courses designed to inform and aid producers in integrating selection for improved feed efficiency into current breeding programs and selection practices.
Outcomes or Projected Impacts
- Increased understanding of the role of growth factors and cell signaling pathways in regulating metabolic processes may lead to molecular- and cellular biology-based strategies to aid in selection of more feed-efficient beef cattle which will benefit both producers and consumers.Increased understanding of the role of growth factors and cell signaling pathways in regulating metabolic processes may lead to molecular- and cellular biology-based strategies to aid in selection of more feed-efficient beef cattle which will benefit both producers and consumers.
- Elucidation of specific genetic variation including SNPs responsible for variation in nutrient utilization efficiency may provide information necessary to utilize genetic selection or molecular- or cell biology-based methods to manipulate metabolic pathways in growing beef cattle. For example, it may be possible to reduce protein degradation in growing muscle, which appears to be one of the mechanisms underpinning variation in this trait. This will allow more rapid muscle growth without increasing energy requirements.
- Gene expression studies may also lead to identification of genes that may be used to produce transgenic animals that grow more efficiently. Additionally, because this project combines the skills of physiologists, nutritionists, muscle biologists, molecular biologists, geneticists, and outreach specialists, collaboration amongst committee members should result in integration of knowledge from the whole animal level to the level of the gene and/or cell in the elucidation of mechanisms that contribute to whole animal variation in nutrient utilization efficiency.
- Improved phenotypic predictions for feed efficiency traits in beef cattle by modeling the genomic, microbial, and genome-by-microbiome interaction effects. Estimating the holobiont effect, otherwise described as holobiability, to determine the host-microbiome influence on phenotypic traits will aid to further define the biological role of host genetics and the rumen microbiome on feed efficiency.
- Research supported by this project will result in training of new researchers equipped to integrate nutrient utilization research with molecular physiology and molecular genomics methods to study specific pathways and regulatory factors and the genes that contribute to variation in efficient use of nutrients for growth and production.
- Increased knowledge of the role of specific molecular physiological mechanisms, genes and gene variants in regulating nutrient utilization efficiency in beef cattle.
Milestones
(2025):Conduct and report on research activities (W3010 participants annual meeting will take place during the Beef Improvement Federation Symposium in Amarillo, TX, June 10th 2025) Publish peer-reviewed articles (including open-access publications) in scientific journals / Contribute to book chapters and review articles Foster collaborative research opportunities within and beyond the project. Disseminate research findings through presentations at conferences, seminars, and to relevant audiences (professionals and the general public): 2025 ASAS CSAS Annual Meeting, Hollywood, FL; July 6th to 10th 2025 ISEP meeting (International Symposium on Protein and Energy Metabolism; Rostock-Warnemünde - Germany; September 15-18)(2026):Conduct and report on research activities / Publish peer-reviewed articles (including open-access publications) in scientific journals / Contribute to book chapters and review articles / Disseminate research findings through presentations at conferences, seminars, and to relevant audiences (professionals and the general public) / Foster collaborative research opportunities within and beyond the project.
(2027):Conduct and report on research activities / Publish peer-reviewed articles (including open-access publications) in scientific journals / Contribute to book chapters and review articles / Disseminate research findings through presentations at conferences, seminars, and to relevant audiences (professionals and the general public) / Foster collaborative research opportunities within and beyond the project.
(2028):Assess and evaluate the achievements of collaborative efforts / Define new research goals and develop a proposal for continued collaborative work / Publish peer-reviewed articles (including open-access publications) in scientific journals / Contribute to book chapters and review articles / Disseminate research findings through presentations at conferences, seminars, and to relevant audiences (professionals and the general public) / Track and report on the success of student and postdoctoral training programs (e.g., career placements).
Projected Participation
View Appendix E: ParticipationOutreach Plan
Project participants have a history of information sharing activities, cooperation, and productivity. Many of the project scientists have been involved in similar areas previously (e.g. WCC-92, W-1010), some for decades. Their expertise and productivity are well documented by previous publications.
It is the intention of the technical committee that new questions and information should flow freely between the laboratory and industry to ultimately benefit the consumer. . This project will have a multifaceted approach to transfer knowledge, skills, technologies to stakeholders. This Multistate Research Project will facilitate collaborations, manuscript reviews, and develop new approaches to understand the variation in nutrient use efficiency of beef cattle. Initial transfer of information to the general public will occur through Extension faculty programming efforts through a series of symposiums and producer meetings. A series of Extension fact sheets will be written based on the journal articles. Segments of the fact sheets or summaries will be placed in Extension newsletters and local newspapers and livestock and forage-related magazines. Web-based information will be prepared with links to the project. Additionally, development of venues that will disseminate the expertise of members within the group, as well as, nationally and internationally recognized leaders in beef cattle feed efficiency will be a priority. This Multistate Research Project will sponsor symposia or workshops on basic understanding of nutrient use efficiency in beef cattle and how that knowledge can be applied to select for productive and profitable cattle operations. This group also will work collaboratively with industry and government leaders to provide additional insight. This will be accomplished by inviting these individuals to annual meetings and requesting their input into research directions for the future and their critique of ongoing research.
It is intended that the preparation of peer-reviewed publications, presentations at professional meetings, and other means of disseminating project results to professional audiences be part of the ongoing project effort. This group also will work collaboratively with industry and government leaders to provide additional insight into solutions for many of the emerging challenges facing the livestock and meat industries. This will be accomplished by inviting these individuals to annual meetings and requesting their input into research directions for the future and their critique of ongoing research. When appropriate, published proceedings or other published work will be released to the appropriate audiences.
Further, we will extend our findings about RFI via models that will be integrated into producer software and outreach activities, such as beef performance projection and ration formulation computer programs. Thus, the economics of the use of animals with different RFI can be quantified. It is anticipated that the utilization of these published results will lead to improved efficiency of lean meat production in domestic livestock and poultry. Additionally, in 2026 the committee will organize a symposium on molecular mechanisms that underlie variation in FE for the annual meeting of ASAS that will convey recent research findings to the members of these organizations.
Organization/Governance
The Technical Committee will consist of at least one representative from each participating unit, appointed as described in the Manual for Cooperative Regional Research, with one representative from each unit designated as the voting member. The Western Regional Association of Directors will appoint one Director to serve as Administrative Advisor and a representative of the USDA/NIFA will also serve as an Advisor; both will be ex officio members of this committee. The Technical Committee will elect a Chair and a Secretary to serve for a period of one year, and these officers will continue to be voting members. In succeeding years, the Secretary will become Chair, and only a new Secretary will be elected. The Chair, the Secretary, the immediate Past Chair and the Administrative Advisor will serve as the Executive Committee, and they will have the authority to act on behalf of the Technical Committee during the periods between meetings.
The Chair, with approval of the Administrative Advisor, will call yearly meetings of the Technical Committee and interim meetings of the Executive Committee if needed. A report of research results from each unit will be presented orally and in writing at the Technical Committee meetings. The written report should include a list of publications resulting from research related to the project for the year. Reports will be critically reviewed by the Technical Committee and recommendations will be made for future research and coordination of research between units to maximize attainment of the Objectives. The Secretary will prepare minutes and an Annual Report for distribution to Technical Committee members, Directors and Department Chairs of participating State Agricultural Experiment Stations and USDA Laboratories, and the Regional Research Office, NIFA. Because submission of a yearly progress report is essential to assess progress, ensure participation, and prepare the Annual Report, any unit that does not submit a written progress report for two consecutive years will be considered not to be participating and will be dropped from the project.
Literature Cited
Abbas W, Howard JT, Paz HA, Hales KE, Wells JE, Kuehn LA, et al. Influence of host genetics in shaping the rumen bacterial community in beef cattle. Scientific Reports. 2020;10(1):15101.
Ahlberg, C. M., K. Allwardt, A. Broocks, K. Bruno, A. Taylor, L. McPhillips, C. R. Krehbiel, M. Calvo-Lorenzo, C. J. Richards, S. E. Place, U. DeSilva, D. L. VanOverbeke, R. G. Mateescu, L. A. Kuehn, R. Weaber, J. Bormann and M. M. Rolf. 2019. Characterization of water intake and water efficiency in beef cattle. Journal of Animal Science 97: 4770–4782.
Allen, M. S. 2014. Drives and limits to feed intake in ruminants. Anim. Prod. Sci. 54:1513–1524. doi:10.1071/AN14478.
Arthur P. F. and R. M. Herd. 2005. Efficiency of feed utilisation by livestock — Implications and benefits of genetic improvement. Canadian Journal of Animal Science. 85: 281-290. https://doi.org/10.4141/A04-062.
ArunKumar, K.E., N. E. Blake, M. Walker, D. Mata-Padrino, I. Holásková and M. E. Wilson. 2024. Boosting techniques for prediction of dry matter intake of cattle in confinement settings and SHAP analysis on the models’ output. Journal of Animal Science.
Baker SD, Szasz JI, Klein TA, Kuber PS, Hunt CW, Glaze JB, Jr., Falk D, Richard R, Miller JC, Battaglia RA, and Hill RA. Residual feed intake of purebred Angus steers: effects on meat quality and palatability. J Anim Sci 84: 938-945, 2006.
Basarab, J., V. Baron, Ó. López-Campos, J. Aalhus, K. Haugen-Kozyra, and E. Okine. 2012. Greenhouse Gas Emissions from Calf- and Yearling-Fed Beef Production Systems, With and Without the Use of Growth Promotants. Animals. 2:195-220. doi:10.3390/ani2020195
Basarab JA, Price MA, Aalhus JL, Okine EK, Snelling WM, and Lyle KL. Residual feed intake and body composition in young growing cattle. Canadian Journal of Animal Science 83: 189-204, 2003.
Basarab, J. A., K. A. Beauchemin, V. S. Baron, K. H. Ominski, L. L. Guan, S. P. Miller, and J. J. Crowley. 2013. Reducing GHG emissions through genetic improvement for feed efficiency: effects on economically important traits and enteric methane production. Anim. 7(s2):303-315. doi:10.1017/S1751731113000888.
Beauchemin, K. A., H. Henry Janzen, S. M. Little, T. A. McAllister, and S. M. McGinn. 2010. Life cycle assessment of greenhouse gas emissions from beef production in western Canada: A case study. Agric. Syst. 103:371-379. doi:10.1016/j.agsy.2010.03.008.
Black, T. E., K. M. Bischoff, V. R. G. Mercadante, G. H. L. Marquezini, N. DiLorenzo, C. C. Chase Jr, S. W. Coleman, T. D. Maddock, and G. C. Lamb. 2013. Relationships among performance, residual feed intake, and temperament assessed in growing beef heifers and subsequently as 3-year-old, lactating beef cows. J. Anim. Sci. 91:2254-2263. doi:10.2527/jas.2012-5242.
Blake, N. E., M. Walker, S. Plum, J. A. Hubbart, J. Hatton, D. Mata-Padrino, I. Holásková and M. E. Wilson 2023. Predicting dry matter intake in beef cattle. Journal of Animal Science: skad269.
Cantalapiedra-Hijar, G., M. Abo-Ismail, G. E. Carstens, L. L. Guan, R. Hegarty, D. A. Kenny, M. McGee, G. Plastow, A. Relling, and I. Ortigues-Marty. 2018. Review: Biological determinants of between-animal variation in feed efficiency of growing beef cattle. Animal. 12:s321–s335. doi:10.1017/S1751731118001489.
Caton JS, Bauer ML, Hidari H. Metabolic components of energy expenditure in growing beef cattle. Asian-Aus J Anim Sci. 2000;13:702-10.
Caton, J.S.; Crouse, M.S.; McLean, K.J.; Dahlen, C.R.;Ward, A.K.; Cushman, R.A.; Grazul-Bilska, A.T.; Neville, B.W.; Borowicz, P.P.; Reynolds, L.P. Maternal periconceptual nutrition, early pregnancy, and developmental outcomes in beef cattle. J. Anim. Sci. 2020, 98, skaa358.
Caton, J.S.; Crouse, M.S.; Reynolds, L.P.; Neville, T.L.; Dahlen, C.R.; Ward, A.K.; Swanson, K.C. Maternal nutrition and programming of offspring energy requirements. Transl. Anim. Sci. 2019, 3, 976–990.
Christensen OF, Börner V, Varona L, Legarra A. Genetic evaluation including intermediate omics features. Genetics. 2021;219(2).
Clemmons BA, Martino C, Powers JB, Campagna SR, Voy BH, Donohoe DR, Gaffney J, Embree MM, Myer PR. Rumen bacteria and serum metabolites predictive of feed efficiency phenotypes in beef cattle. Scientific Reports. 2019 Dec 17;9(1):19265.
Clemmons BA, Mihelic RI, Beckford RC, Powers JB, Melchior EA, McFarlane ZD, Cope ER, Embree MM, Mulliniks JT, Campagna SR, Voy BH. 2017. Serum metabolites associated with feed efficiency in black angus steers. Metabolomics. 13:147. doi:10.1007/s11306-017-1282-z
Consolo, N. R. B., V. L. M. Buarque, J. Silva, M. D. Poleti, L. C. G. S. Barbosa, A. Higuera- Padilla, J. F. M. Gomez, L. A. Colnago, D. E. Gerrard, N. A. Saran, and S. L. Silva. 2021. Muscle and liver metabolomic signatures associated with residual feed intake in Nellore cattle. Anim. Feed. Sci. Tech. 271:114757. doi:10.1016/j.anifeedsci.2020.114757
Crouse, M.S.; McCarthy, K.L.; Menezes, A.C.B.; Kassetas, C.J.; Baumgaertner, F.; Kirsch, J.D.; Dorsam, S.; Neville, T.L.; Ward, A.K.; Borowicz, P.P.; et al. Vitamin and Mineral Supplementation and Rate of Weight Gain during the First Trimester of Gestation in Beef Heifers Alters the Fetal Liver Amino Acid, Carbohydrate, and Energy Profile at Day 83 of Gestation. Metabolites 2022, 12, 696.
Difford GF, Plichta DR, Løvendahl P, Lassen J, Noel SJ, Højberg O, et al. Host genetics and the rumen microbiome jointly associate with methane emissions in dairy cows. PLOS Genetics. 2018;14(10):e1007580.
Ensminger, M. E. 1990. Animal Science. Pearson. ISBN 0813428874.
Ferrell, C.L.; Jenkins, T.G. Cow type and the nutritional environment: nutritional aspects. J. Anim. Sci. 1985. 61(3):725-741. doi: 10.2527/jas1985.613725x.
Fitzsimons, C., D. A. Kenny, M. H. Deighton, A. G. Fahey, and M. McGee. 2013. Methane emissions, body composition, and rumen fermentation traits of beef heifers. J. Anim. Sci. 91:5789–5800. doi:10.2527/jas.2013-6956.
Fitzsimons, C., D. A. Kenny, A. G. Fahey, and M. McGee. 2014. Feeding behavior, ruminal fermentation, and performance of pregnant beef cows differing in phenotypic residual feed intake offered grass silage. J. Anim. Sci. 92:2170-2181. doi:10.2527/jas.2013-7438.
Foroutan, A., C. Fitzsimmons, R. Mandal, M. V. Berjanskii, and D. S. Wishart. 2020. Serum Metabolite Biomarkers for Predicting Residual Feed Intake (RFI) of Young Angus Bulls. Metabolites. 10:491. doi:10.3390/metabo10120491
Galyean, M. L. and K. E Hales. 2022. Prediction of methane per unit of dry matter intake in growing and finishing cattle from the ratio of dietary concentrations of starch to neutral detergent fiber alone or in combination with dietary concentration of ether extract. Journal of Animal Science 100 skac243, https://doi.org/10.1093/jas/skac243.
Galyean, M.L.; Hales, K.E. 2024. Relationships between Dietary Chemical Components and Enteric Methane Production and Application to Diet Formulation in Beef Cattle. Methane 3:1-11. https://doi.org/10.3390/methane3010001
Goldansaz, S. A., S. Markus, M. Berjanskii, M. Rout, A. C. Guo, Z. Wang, G. Plastow, and D. S. Wishart. 2020. Candidate serum metabolite biomarkers of residual feed intake and carcass merit in sheep. J. Anim. Sci. 98:1-15. doi:10.1093/jas/skaa298.
Gregorini, P., G. C. Waghorn, B. Kuhn-Sherlock, A. J. Romera, and K. A. Macdonald. 2015. Grazing pattern of dairy cows that were selected for divergent residual feed intake as calves. J. Dairy. Sci. 98:6486-6491. doi:10.3168/jds.2015-9614.
Hafla AN, GE Carstens, TDA Forbes, LO Tedeschi, JC Bailey, and JT Walter, JR Johnson. Relationships between postweaning residual feed intake in heifers and forage utilization, body composition, feeding behavior, physical activity and heart rate of pregnant beef females. Journal Animal Science, 91:2013-6423, 2013.
Hegarty, R. S., J. P. Goopy, R. M. Herd, and B. McCorkell. 2007. Cattle selected for lower residual feed intake have reduced daily methane production. J. Anim. Sci. 85:1479–1486. doi:10.2527/jas.2006-236.
Henniger, MT, Beever, J, Rowan TN, Voy BH, Wells, JE, Kuehn, LA, and Myer PR. 2025. Host Genetics, the Rumen Microbiome, and Holobiont Effects Jointly Contribute to Feed Efficiency in Beef Cattle. Dissertation. Accessed 1/2025. Trace, University of Tennessee.
Herd, R. M., and P. F. Arthur. 2009. Physiological basis for residual feed intake. J. Anim. Sci. 87(14 Suppl):E64-E71. doi:10.2527/jas.2008-1345.
Johnson, J. R., G. E. Carstens, W. K. Krueger, P. A. Lancaster, E. G. Brown, L. O. Tedeschi, R. C. Anderson, K. A. Johnson, and A. Brosh. 2019a. Associations between residual feed intake and apparent nutrient digestibility, in vitro methane-producing activity, and volatile fatty acid concentrations in growing beef cattle. J. Anim. Sci. 8:3550-3561. doi:10.1093/jas/skz195.
Karisa, B. K., J. Thomson, Z. Wang, C. Li, Y. R. Montanholi, S. P. Miller, S. S. Moore, and G. S. Plastow. 2014. Plasma metabolites associated with residual feed intake and other productivity performance traits in beef cattle. Livest. Sci. 165:200-211. doi:10.1016/j.livsci.2014.03.002.
Klopatek, S. C. and J. W. Oltjen. 2022. How advances in animal efficiency and management have affected beef cattle’s water intensity in the United States: 1991 compared to 2019. Journal of Animal Science 100 skac297 https://doi.org/10.1093/jas/skac297.
Koch, R.M.; Swiger, L.A.; Chambers, D. et al. Efficiency of feed use in beef cattle. Journal of Animal Science, v.22, p.486-494, 1963.
Koknaroglu, H., D.D. Loy, D.E. Wilson, M.P. Hoffman, J.D. Lawrence. 2005. Factors Affecting Beef Cattle Performance and Profitability, The Professional Animal Scientist 21:286-296 https://doi.org/10.15232/S1080-7446(15)31220-1
Kolath, W. H., C. Huisma and M. S. Kerley. 2007. CASE STUDY: An Evaluation of the Potential to Measure Real-Time Body Weight of Feedlot Cattle. The Professional Animal Scientist 23:295-299.
Lancaster, P. A., G. E. Carstens, D. H. Crews, T. H. Welsh, T. D. A. Forbes, D. W. Forrest, L. O. Tedeschi, R. D. Randel, and F. M. Rouquette. 2009. Phenotypic and genetic relationships of residual feed intake with performance and ultrasound carcass traits in Brangus heifers. J. Anim. Sci. 87:3887–3896. doi:10.2527/jas.2009-2041.
Li F, Li C, Chen Y, Liu J, Zhang C, Irving B, et al. Host genetics influence the rumen microbiota and heritable rumen microbial features associate with feed efficiency in cattle. Microbiome. 2019;7(1):92.
Manafiazar, G., J. A. Basarab, V. S. Baron, L. McKeown, R. R. Doce, M. Swift, M. Undi, K.
Wittenberg, and K. Ominski. 2015. Effect of post-weaning residual feed intake classification
on grazed grass intake and performance in pregnant beef heifers. Can. J. Anim. Sci. 95:369-
- doi:10.4141/cjas-2014-184.
Manafiazar, G., T. K. Flesch, V. S. Baron, L. McKeown, B. Byron, H. Block, K. Ominski, G.
Plastow, and J. A. Basarab. 2020. Methane and carbon dioxide emissions and grazed forage
intake from pregnant beef heifers previously classified for residual feed intake under drylot
conditions. Can. J. Anim. Sci. 101:71-84. doi:10.1139/cjas-2019-0182.
Martínez-Álvaro M, Auffret MD, Duthie C-A, Dewhurst RJ, Cleveland MA, Watson M, Roehe R. Bovine host genome acts on rumen microbiome function linked to methane emissions. Communications Biology. 2022;5(1):350.
Martínez-Álvaro M, Mattock J, González-Recio Ó, Saborío-Montero A, Weng Z, Lima J, et al. Including microbiome information in a multi-trait genomic evaluation: a case study on longitudinal growth performance in beef cattle. Genetics Selection Evolution. 2024;56(1):19.
McCann, J. C., T. A. Wickersham, and J. J. Loor. 2014a. High-throughput methods redefine the rumen microbiome and its relationship with nutrition and metabolism. Bioinform. Biol. Insights. 8:109-125. doi:10.4137/BBI.S15389.
Menezes, A.C.B.; Baumgaertner, F.; Hurlbert, J.L.; Bochantin, K.A.; Kirsch, J.D.; Dorsam, S.; Sedivec, K.K.; Swanson, K.C.; Dahlen, C.R. Feeding a Se-Containing Trace Mineral Supplement during Pregnancy: Effects on Mitochondrial Oxygen Consumption in Liver and Small Intestine of Neonatal Calves. In Proceedings of the 12th International Symposium on Selenium in Biology and Medicine, Honolulu, HI, USA, 16–20 February 2022.
Myer PR, Smith TP, Wells JE, Kuehn LA, Freetly HC. Rumen microbiome from steers differing in feed efficiency. PloS one. 2015 Jun 1;10(6):e0129174.
National Academies of Sciences, Engineering, and Medicine. 2016. Nutrient Requirements of Beef Cattle: Eighth Revised Edition. Washington, DC: The National Academies Press. https://doi.org/10.17226/19014.
O’Reilly, K., G. E. Carstens, J. R. Johnson, N. Deeb, and P. Ross. 2024. Association of genomically enhanced residual feed intake with performance, feed efficiency, feeding behavior, gas flux, and nutrient digestibility in growing Holstein heifers. J. Anim. Sci. skae289.
Parsons, I. L., J. R. Johnson, W. C. Kayser, L. O. Tedeschi, and G. E. Carstens. 2020.
Characterization of feeding behavior traits in steers with divergent residual feed intake
consuming a high-concentrate diet. J. Anim. Sci. 98:1-9. doi:10.1093/jas/skaa189. doi:10.1093/jas/skae289.
Richardson EC, and Herd RM. Biological basis for variation in residual feed intake in beef cattle. 2. Synthesis of results following divergent selection. Australian Journal of Experimental Agriculture 44: 431-440, 2004.
Rohart, F., A. Paris, B. Laurent, C. Canlet, J. Molina, M. J. Mercat, T. Tribout, N. Muller, N. Iannuccelli, N. Villa-Vialaneix, L. Liaubet, D. Milan, and M. San Cristobal. 2012. Phenotypic prediction based on metabolomic data for growing pigs from three main European breeds, J. Anim. Sci. 90: 4729–4740. doi:10.2527/jas.2012-5338.
Rolfe DFS, Brown GC. Cellular energy utilization and molecular origin of standard metabolic rate in mammals. Physiol Rev. 1997;77:731-58.
Ross EM, Moate PJ, Marett LC, Cocks BG, Hayes BJ. Metagenomic Predictions: From Microbiome to Complex Health and Environmental Phenotypes in Humans and Cattle. PLoS One. 2013;8(9):e73056.
Rowan, T. N. 2022. Invited Review: Genetic decision tools for increasing cow efficiency and sustainability in forage-based beef systems. Applied Animal Science 38:660-670 https://doi.org/10.15232/aas.2022-02306.
Sasson G, Ben-Shabat SK, Seroussi E, Doron-Faigenboim A, Shterzer N, Yaacoby S, et al. Heritable Bovine Rumen Bacteria Are Phylogenetically Related and Correlated with the Cow’s Capacity To Harvest Energy from Its Feed. mBio. 2017;8(4):e00703-17.
Sprinkle, J. E., M. J. Ellison, J. B. Hall, J. V. Yelich, C. M. Willmore, and J. R. Brennan.
- Grazing behavior and production for lactating cows differing in residual feed intake
while grazing spring and summer rangeland. Transl. Anim. Sci. 5:txab063.
doi:10.1093/tas/txab063.
Swanson K, Miller S. Factors regulating feed efficiency and nutrient utilization in beef cattle. In: France J, Kebreab E, editors. Mathematical Modelling in Animal Nutrition. Cambridge, MA: CAB International; 2008. p. 419-41.
Trenkle, A.; Willham, R.L. Beef Production Efficiency: The efficiency of beef production can be improved by applying knowledge of nutrition and breeding. Sci. 1977. 198, 1009-1015. Doi: 10.1126/science.198.4321.1009.
USDA NASS, Agricultural Statistics Board. Cattle Inventory Released January 31,2024. ISSN: 1948-9099. https://downloads.usda.library.cornell.edu/usda-esmis/files/h702q636h/6108x003v/kk91h696g/catl0124.pdf.
USDA Climate Hubs. Grazing lands in a changing climate. Accessed 8/16/2024. https://www.climatehubs.usda.gov/grazing-lands-changing-climate.
Waghorn, G. C., and R. S. Hegarty. 2011. Lowering ruminant methane emissions through
improved feed conversion efficiency. Anim. Feed. Sci. Tech. 166:291-301.
doi:10.1016/j.anifeedsci.2011.04.019.
Wallace RJ, Sasson G, Garnsworthy PC, Tapio I, Gregson E, Bani P, et al. A heritable subset of the core rumen microbiome dictates dairy cow productivity and emissions. Science Advances. 2019;5(7):eaav8391.
Wang W, Wei Z, Li Z, Ren J, Song Y, Xu J, et al. Integrating genome- and transcriptome-wide association studies to uncover the host–microbiome interactions in bovine rumen methanogenesis. iMeta. 2024;3(5):e234.
Wehrbein, M.A.; Velasquez-Moreno, E.; Menendez, H.M.; Rusche, W.C.; Smith, Z.K.; Menezes, A.C.B. PSVII-30 Winter-feeding high concentrate diets reduces enteric methane emissions pre-calving in beef cows, Journal of Animal Science, Volume 102, Issue Supplement_3, September 2024, Pages 679–680, doi:10.1093/jas/skae234.768 . Data presented at the National ASAS Meeting (Calgary, Canada, July 2024)
Wehrbein, M.A.; Jardon, G.H.; Menendez, H.M.; Rusche, W.C.; Smith, Z.K.; Menezes, A.C.B. Winter-feeding a high concentrate corn-based diet pre- and post-calving improves milk production in beef cows. Submitted and to be presented at the 2025 ASAS Midwest meeting (March 9 – 12th, Omaha, NE)
Wehrbein, M.A.; Jardon, G.H.; Menendez, H.M.; Rusche, W.C.; Smith, Z.K.; Menezes, A.C.B. Winter-feeding high concentrate diets minimizes the carbon footprint and improves water usage efficiency in cow/calf systems. Submitted and to be presented at the 2025 ASAS Midwest meeting (March 9 – 12th, Omaha, NE)
Weikard, R., E. Altmaier, K. Suhre, K. M. Weinberger, H. M. Hammon, E. Albrecht, K. Setoguchi, A. Takasuga, and C. Khun. 2010. Metabolomic profiles indicate distinct physiological pathways affected by two loci with major divergent effect on Bos taurus growth and lipid deposition. Physiol. Genomics 42A:79 –88. doi:10.1152/physiolgenomics.00120.2010.
Xue M-Y, Sun H-Z, Wu X-H, Liu J-X, Guan LL. Multi-omics reveals that the rumen microbiome and its metabolome together with the host metabolome contribute to individualized dairy cow performance. Microbiome. 2020;8(1):64.
Yang F, Henniger MT, Izzo AS, Melchior EA, Clemmons BA, Oliver MA, Gaffney JR, Martino C, Ault-Seay TB, Striluk ML, Embree JJ, Cordero JF, Mulon PY, Anderson DE, Embree MM, and Myer PR. 2025. Performance improvements and increased ruminal microbial interactions in Angus heifers via supplementation with native rumen bacteria during high-grain challenge. Scientific Reports. doi: 10.1038/s41598-025-86331-1
Zang X-W, Sun H-Z, Xue M-Y, Zhang Z, Plastow G, Yang T, et al. Heritable and Nonheritable Rumen Bacteria Are Associated with Different Characters of Lactation Performance of Dairy Cows. mSystems. 2022;7(5).
Zhang Q, Difford G, Sahana G, Løvendahl P, Lassen J, Lund MS, et al. Bayesian modeling reveals host genetics associated with rumen microbiota jointly influence methane emission in dairy cows. The ISME Journal. 2020;14(8):2019-33.