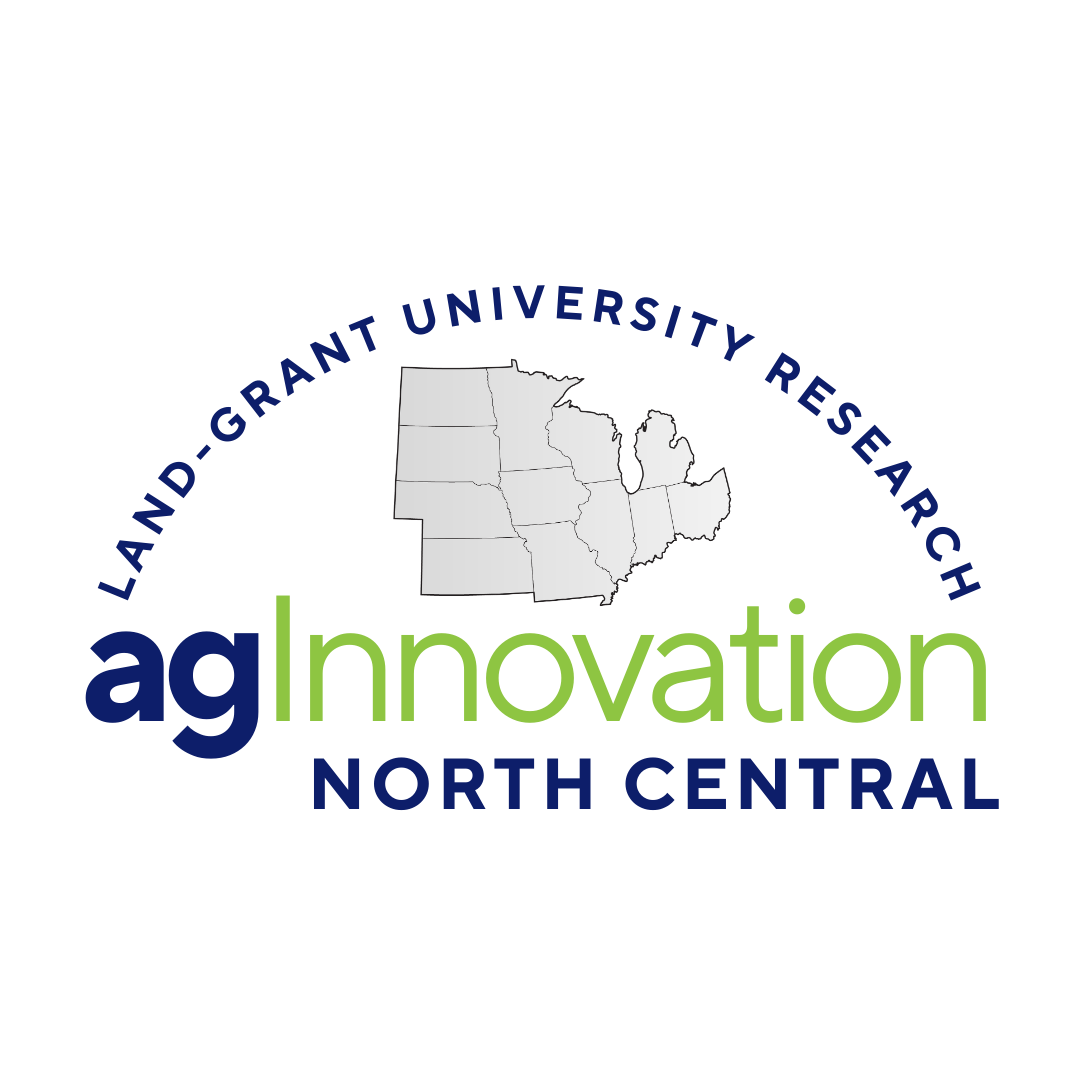
NC246: Ecology and Management of Arthropods in Corn
(Multistate Research Project)
Status: Approved Pending Start Date
NC246: Ecology and Management of Arthropods in Corn
Duration: 10/01/2025 to 09/30/2030
Administrative Advisor(s):
NIFA Reps:
Non-Technical Summary
The U.S. is one of the world’s leading corn producers, with annual grain production averaging 14.3 billion bushels and $73.3 billion annually from 2019-2023. Corn yields are negatively impacted by a variety of arthropod pests (most of which are insects) with variations in regional importance, including the western and northern corn rootworm, the European corn borer, corn earworm, fall armyworm, stink bugs, and others. Corn farmers face pest management challenges, including the development of resistance to insecticides and transgenic crops expressing Bt toxins for insect management, difficulties in assessing pest prevalence, and the emergence of new and invasive pest species. Because these issues are rarely confined within state lines, this multi-state committee coordinates research and extension efforts aimed at better understanding and managing arthropod pests in corn. Our objectives include the following: investigating corn arthropod biology; developing integrated pest management practices for arthropod pests; documenting, tracking, and mitigating insect resistance to control tactics; and developing and communicating best management practices for U.S. corn farmers to combat these pests. In addition to farmers, our target audience includes crop advisors, the seed, chemical, and biotechnology industries, policymakers, and others involved in using, developing, or deploying corn pest management technologies and management practices. Our project activities result in sound, research-based pest management recommendations, improved farmer profitability, identification of critical new issues in pest management (such as resistant populations or new invasive pests), and an improved understanding of pest biology and ecology.
Statement of Issues and Justification
The U.S. is one of the world’s top corn producers. In 2023, corn production in the U.S. reached a record of more than 15.3 billion bushels in a total harvested area of 86.5 million acres. In 2024, projections indicated around 14.8 billion bushels of corn, which is expected to account for approximately 35% of global corn production. Field corn accounts for 99% of the country’s acreage, with the remaining corn acreage cultivated with sweet corn, popcorn, and other specialty varieties for fresh or processed markets. The total production value of U.S. corn in 2022 was estimated at $97.2 billion.
Corn yield is inconsistent across regions, and competition with a variety of insect pests contributes to this variability. Together with weather, planting conditions, and competition with weeds and diseases, arthropod pests are critical sources of economic yield losses. The European corn borer, Ostrinia nubilalis (Lepidoptera: Crambidae), and two species of corn rootworms, Diabrotica spp. (Coleoptera: Chrysomelidae), have been historical key pests of corn in the U.S., with each having an estimated impact of over $1 billion annually due to the management costs and yield losses. Several additional lepidopteran pests have been listed as economic pests of corn in the U.S. Helicoverpa zea (Lepidoptera: Noctuidae), commonly known as corn earworm, can be a serious pest to sweet corn (Olmstead et al. 2016), particularly when produced for the fresh market. Temperatures influence the population dynamics and survival of this species, and collaborative multistate work has proposed three overwintering zones in North America: the southern range (below 35oN), where H. zea survives winter temperatures; the transitional zone, where overwintering survival varies with yearly temperatures; and the northern limits, where winter temperatures are lethal to H. zea. In field corn, this pest is commonly present in southern states, and injury is mostly limited to kernel feeding on the tip of the ear, which usually does not cause an economic impact (Reay-Jones 2019). However, due to the highly polyphagous behavior of the species, corn serves as an important source of infestation for other economic crops which may facilitate migration and colonization of crops on a continental scale in the U.S. (Paula-Moraes et al. 2024). Fall armyworm, Spodoptera frugiperda (Lepidoptera: Noctuidae), also poses an economic threat to the U.S., especially to sweet corn production, with the potential to reduce yields by 20-40% in some cases, due to the feeding on leaves, stems, and kernels. This species is also a strong flier, being the southeast region hub for genetic flow (Paula-Moraes et al. 2024) and reaching northern latitudes every summer (Tessnow et al., 2023). Another economic pest associated with corn is western bean cutworm, Striacosta albicosta (Lepidoptera: Noctuidae). The first reports of this species come from the beginning of the last century, and it has since extended its geographical distribution northward and eastward, from the western Great Plains into the corn-producing regions of the Midwest and the eastern U.S. and Canada. A study found that the mean yield loss from a single larva per corn plant was 945.52 kg/ha (15.08 bu/acre) (Paula-Moraes et al. 2013).
Western corn rootworm, Diabrotica virgifera virgifera, and northern corn rootworm, D. barberi, make up the most economically important insect pest complex in corn, particularly in the western portion of the U.S. corn belt (Wechsler and Smith, 2018). While both species are capable of overcoming crop rotation as a control strategy (Gray et al. 2009; French et al. 2014), corn rootworm population densities and subsequent yield losses are greatest in areas where continuous corn production is prevalent. These species have a long history of overcoming pest control tactics such as insecticides, crop rotation, and transgenic traits through the development of resistant populations (Gray et al. 2009). The broad deployment of transgenic hybrids expressing multiple toxins of the bacteria Bacillus thuringiensis (Bt) for control of corn rootworms has contributed to large-scale reductions in rootworm population densities in much of the corn belt (Tinsley et al., 2018). However, the ongoing development of resistance to these transgenic hybrids in both western and northern corn rootworms poses a substantial pest management challenge to U.S. corn production.
Transgenic plants expressing Bt toxins have been broadly adopted in the U.S. to control O. nubilalis and other lepidopteran pests, in addition to rootworms. The Bt corn acreage has since increased, with more than 80% of all corn planted in the U.S. being genetically engineered in 2024, primarily with Bt traits for insect resistance (National Agricultural Statistics Service, 2024). Currently, several commercial traits are available to manage the aforementioned lepidopteran and coleopteran pests associated with corn, contributing to decreasing the use of insecticides and promoting IPM. However, several cases of increased resistant populations of target pests to Bt traits in corn and novel pest problems not targeted by Bt technology have underlined the need to continue research and Extension work. The overall contribution of supporting the diversification of options for pest management is the sustainability of corn production in the U.S.
In addition, other pest species are associated with corn production and are considered in IPM programs. For instance, a complex of stinkbug species (Hemiptera: Pentatomidae), including the brown marmorated stinkbug, Halyomorpha halys, and the brown stink bug, Euschistus servus, have been reported to affect corn crops across various regions in the U. S. The impact can vary year by year, but it is usually the top pest of corn in the southern U.S. (Reisig et al. 2024). One of the most identifiable symptoms of this pest is ear deformity, commonly named “banana-shaped” ear.
The NC246 committee addresses critical pest issues previously discussed and represents a collaborative effort between members working with corn on a continental scale in the U.S. The committee’s work has a long history of working with above and below-ground corn pests. Two committees were initially established. The initial NC205 (Ecology and Management of European Corn Borer and Other Lepidopteran Pests of Corn), established in 1953, and the NCCC46 (Development, Optimization and Delivery of Management Strategies for Corn Rootworms) started co-locating their annual meeting in the 1990s with an increasing interaction between members from the two committees. In 2016, the current NC246 committee was formed, merging the two committees and promoting research and Extension collaborations addressing the corn pest complex and emerging problems, such as insect resistance to Bt transgenic corn targeting corn rootworm and lepidopteran pests.
Related, Current and Previous Work
A CRIS search indicates 127 active projects related to ‘corn Lepidoptera,’ 126 for ‘corn Diabrotica,’ and 189 for ‘corn insects.’ Of these, 43 are directly associated with NC246, and many include participants who are NC246 members.
Currently, the members of NC246 include representatives from universities and USDA ARS units, primarily located in the Corn Belt and southeastern U.S. Our multi-state committee's activities supplement and enhance these members' work in multiple ways. During discussions at our annual meeting, NC246 often results in multi-state projects that grow out of existing projects from researchers in one or a few states. For instance, our sentinel Bt monitoring network for lepidopteran pests in corn, which by 2023 had grown to include 44 cooperators running 60 trial locations in 25 states and 3 Canadian provinces, began with five sites, all located in Maryland.
A NIMSS search disclosed three active Multistate Research Projects other than NC246 that include work on corn arthropod pests: NE-2332 Biological Control of Arthropod Pests and Weeds; S-1070 The Working Group on Improving Microbial Control of Arthropod Pests; and NE2001: Harnessing Chemical Ecology to Address Agricultural Pest and Pollinator Priorities. All of these projects are focused on a single aspect of IPM across multiple crops; therefore, their potential for overlap with our group is minimal. Another Multistate Research Project, S1080 Improving Soybean Arthropod Pest Management in the U.S., has a similar scope to NC246 and several members in common; however, the objectives for this group are focused on soybean. We have established cooperative interactions with members of these committees and will continue to coordinate efforts with other regional projects.
Objective 1: Investigate the ecology, biology, evolution, genetics, genomics, and behavior of corn arthropods.
NC246 members have developed genomic resources for western corn rootworm (WCR), including a large-insert bacterial artificial chromosome library, DvvBAC1 (Coates et al. 2012), SNP markers (Coates et al. 2009), and transcriptome assemblies (Siegfried et al. 2005; Knolhoff et al. 2010; Eyun et al. 2014; Wang et al. 2017; Adedipe et al. 2019; Zhao et al. 2019; Coates et al. 2021; Zhao et al. 2021). Additionally, the Diabrotica Genetics Consortium, which includes several NC246 scientists, developed a draft genome assembly for an inbred strain maintained at USDA-ARS in Brookings, SD (Coates et al. 2023). These resources predicted differential regulation of transcripts encoding putative detoxification genes among pyrethroid resistant beetles (Souza et al. 2020), cell stress- and repair-related genes among susceptible larvae exposed to Cry3Bb1 and Gpp34/Tpp35Ab1 transgenic corn (Coates et al. 2021), and among larvae in response to feeding on different host plants (Coates et al. 2023). Genome size estimates for six Diabrotica species showed that those for D. v. virgifera, D. v. zeae (Mexican corn rootworm; MCR), and D. barberi (northern corn rootworm; NCR) are between 2.26 and 2.58 Gbp, whereas the genome of D. undecimpunctata (southern corn rootworm; SCR) is about two-thirds as large at 1.64 Gbp (Lata et al. 2022). Genome sequencing data from NCR and SCR were additionally mined to identify integrated viral DNA (Liu et al. 2021; Liu et al. 2022), which provides insight into structural and functional genome evolution among rootworm species.
Genomic resources, including a genome assembly (Coates et al. unpublished; GenBank SWFO00000000.1), have been developed for European corn borer (ECB) to better understand its biology and the potential for resistance development to Bt proteins. In ECB, differential expression of midgut genes (Vellichirammal et al. 2015) and a single QTL were linked to Cry1F resistance (Coates et al. 2011) and associated with mutation in an ATP binding cassette (ABC) transporter subfamily C (ABCC) gene family member (Coates and Siegfried 2015; Farhan et al. 2023). Cry1Ab resistance in a lab-selected ECB strain with ~2500-fold increase in resistance (Coates et al. 2008) is controlled by two QTL that modify the expression of an unlinked aminopeptidase N1receptor (Coates et al. 2013b). These studies are pertinent given the recent documentation that ECB populations in Canada have developed practical resistance to transgenic corn hybrids that express Cry1F and Cry1Ab (Smith et al. 2019; Smith and Farhan 2023), and evidence of Cry1F resistance in Connecticut suggests it has spread to the northeast U.S. (Fisher unpublished). Given the migratory behavior of ECB populations (Sappington 2024), it is crucial to detect and estimate the frequency of ECB resistance alleles in U.S. populations that may be dispersing through migratory movement and to understand gene flow between different ecotypes of ECB. Genetic markers have been additionally used to study ECB population-specific differences in pheromone races and diapause ecotypes.
Field fall armyworm (FAW) resistance to corn producing the Cry1F protein first emerged in Puerto Rico (Storer et al. 2010) but was later found in Florida and North Carolina (Huang et al. 2014). This sequence of resistance detection matches migratory models for FAW in North America and suggests the dispersal of resistant FAW from the Caribbean into overwintering FAW populations in Florida and subsequent northward migration along the eastern U.S. seaboard (Tessnow et al. 2023; Westbrook et al. 2019). Development and extensive testing using multiplexed targeted sequencing for high throughput genotyping of field FAW samples failed to detect the allele linked with FAW resistance to Cry1F from Puerto Rico in the continental U.S. (Tandy et al. 2023; Banerjee et al. 2017; Banerjee et al. 2022; Schlum et al. 2021). Identifying a distinct resistance allele from Florida provided further evidence supporting that FAW resistance to Cry1F corn evolves locally and not due to migratory dispersal (Banerjee et al. 2022). This hypothesis is in line with lower migratory flight tendency in resistant FAW adults (De Bortoli et al. 2024), which can have relevant implications for IRM practices. More recently, reversed Cry1F susceptibility in FAW was identified when a multistate collaborative study performed bioassays on populations from seven southern states, including nine populations from the southeastern coastal region (Silva et al. 2024)
The estimated frequency for Cry1Ac and Cry2Aa resistance in the corn earworm (CEW) population was initially low (≤ 0.00042) (Burd et al. 2003), but prevalence of damage to Bt corn has increased (Dively et al., 2016; Reisig et al. 2018; Bilbo et al., 2019; Yang et al., 2019; Kaur et al., 2019; Dively et al. 2021; Dively et al. 2023; Huang et al. 2023, Reay-Jones et al. 2024). Inheritance of Cry1Ac resistance is non-recessive (Burd et al. 2003). Cry1A.105 and Cry2Ab2 resistance in CEW is autosomal and non-recessive, with resistance to Cry1A.105 controlled by a single locus (Yu et al. 2022) and Cry2Ab2 shown to be polygenic (Yang et al., 2020a; Yang et al. 2021a). In contrast, genetic analyses of pedigrees predict that at least two loci of major effect underlay Cry1Ab resistance in CEW, whereas inheritance of Cry1A.105 + Cry2Ab2 resistance is controlled by a single major locus (Taylor et al. 2021). The single QTL of major effect shared between Cry1Ab and Cry1A.105 + Cry2Ab2 resistance mapped to a region of the CEW genome encoding a tandem array of duplicated trypsin genes (Taylor et al. 2024). Cry1Ac resistance was linked to a premature gene stop codon in the kinesin gene for the field-derived Cry1Ab resistant in GA-R strain of CEW (Benowitz et al. 2022). Interestingly, although CRISPR/Cas-mediated knockout of CEW ABCA1, HaABCA1, resulted in significantly reduced Cry2Ab2 susceptibility in wildtype larvae and thus was suggested to function as a midgut receptor (Fabrick et al. 2022), the HzABCA1 gene has no known linkage to Cry2Ab2 resistance in field populations. CEW field populations remain susceptible to Vip3A transgenic crops (Niu et al. 2021), though recent work by NC246 has shown an increase in the phenotypic frequency of resistance (Dively et al. 2023). Alleles for Vip3A resistance were estimated at 0.0065 in a Texas CEW field population (Yang et al. 2020b) and caused reduced Vip3A binding in the midgut (Kerns et al. 2023). The alleles for this autosomal monogenic trait are recessive (Yang et al. 2021b). A chromosome-level assembly was generated for a Cry1Ac resistance strain of CEW (Stahlke et al. 2022) and will be a resource for future investigations on Bt resistance, and population and ecological genetic studies. In addition, the migratory capacity of this species has been investigated in populations from different latitudes of the continental U.S. in a collaborative effort, including NC246 members. Using a biogeochemical marker, evidence of a northward spring/summer migration of this species in North America was confirmed and underscored the significance of the southeastern U.S. as a hub for genetic flow. The results of hydrogen isotopic rations also indicated strong evidence of reverse (southward) migration of CEW from the continental U.S. to Puerto Rico (Paula-Moraes et al. 2024).
Under the differential survival hypothesis, selection for resistance increases if individuals heterozygous for alleles at resistance loci survive in blended refuge (Mallet and Porter 1992; Gould 1994; Onstad et al. 2018). In support of this hypothesis, blended refuge increased the dominance of resistance to Cry toxins in both FAW (Yang et al. 2017) and CEW (Brévault et al. 2015). While CEW pupation depth, adult flight distance, and eclosion time were unaffected by blended refuge (Pezzini et al. 2023), the genomic signatures of Bt resistance were detected in a single generation of development on blended refuge ears (Pezzini et al. 2024). This suggests that blended refuge will accelerate the evolutionary rate of resistance for CEW. Blended refuge provides sublethal doses of insecticide that influence ear-feeding pests like CEW (Bilbo et al. 2018, Reay-Jones et al. 2020).
Helicoverpa armigera is native to Europe, Asia and Africa, and is invasive in South America (Arnemann et al. 2016; Arnemann et al. 2019) and the Caribbean (Smith 2014). Detection of a few adults in the U.S. occurred in Florida in 2014 (Hayden and Brambila 2015) and at the Chicago O’Hare Airport in 2019 and 2022 (USDA-APHIS, unpublished). Helicoverpa armigera is a closely related sister species of CEW, and interspecies mating produces reproductively viable hybrids. Gene flow has occurred between these closely related species, and a gene putatively conferring selective advantage may have risen in frequency in the CEW recipient genome (a process called adaptive introgression). Specifically, the H. armigera-specific locus CYP337B3, encoding a chimeric cytochrome P450 derived from an unequal crossover between Helicoverpa CYP337B1 and B2 genes and associated with elevated resistance to pyrethroid insecticides (Walsh et al. 2018), was detected among CEW in South America (Valencia-Montoya et al. 2020). The H. armigera-specific CYP337B3 locus was subsequently detected among CEW collected in Texas during 2019 (North et al. 2023) and from nearly 25% of moths collected in 2023 near a location in Colorado where pyrethroid spay applications failed to control CEW damage to sweet corn that season (Nufer et al. 2024). The level of introgression for CYP337B3 introgression, or other adaptive genes or alleles, across the CEW population remains unknown, and studies are in progress to document how this movement of resistance traits into CEW may impact the efficacy of current management practices.
Objective 2: Develop and assess IPM systems for the arthropod complex in corn.
A study of the spatial characterization patterns of species of stink bugs was performed with the objective of improving sampling plans in corn. Analysis of distance indices (SADIE) indicated aggregation patterns primarily in adult populations and less occurrence in nymphal populations. In addition, the spatial association of stink bug species and their injury varied with corn phenology stages. During the early vegetative stage, a lack of spatial association between stink bug populations suggested a need for intensive sampling, particularly in fields with increased residue from cover crops (Bryant et al. 2023). Overall, the work promotes improvement of management decision-making in IPM programs for this group of pests in corn, which is primarily based on insecticide use.
Objective 3: Characterize and monitor pest resistance and assess IRM and mitigation strategies.
Field-evolved resistance by WCR to insecticides is well documented. Resistance has been confirmed to insecticides in the cyclodiene, carbamate, and organophosphate classes (Ball and Weekman 1962, 1963; Meinke et al. 1998) and recently the pyrethroid class (Pereira et al. 2015). Data collected to date indicate that repeated exposure of adult WCR to foliar applications of pyrethroid insecticides led to resistance evolution. Adult resistance monitoring in the U.S. Corn Belt with a diagnostic dose of bifenthrin indicated that resistance was limited to the western Corn Belt (especially SW Nebraska and SW Kansas, Pereira et al. 2015). Follow-up work using simulated aerial application methodology confirmed that formulated bifenthrin was ineffective at the highest registered rate (Souza et al. 2019a). Results of replicated field trials also indicated that formulated soil-applied WCR pyrethroid insecticides were ineffective in areas where adult pyrethroid resistance had been documented (Souza et al. 2019b). Cross-resistance and synergism bioassays suggested that both detoxifying enzymes and target site insensitivity might be involved as resistance mechanisms (Pereira et al. 2017). Follow-up biochemical assays using model substrates as well as high-throughput RNA-sequencing revealed that insecticide-related metabolism in pyrethroid-resistant WCR populations was significantly enhanced compared to pyrethroid-susceptible populations (Souza 2019).
With the advent of pyramided Bt corn (corn with two or more Bt insecticidal proteins with different modes of action targeting one or closely related insect species), the refuge has evolved from a structured to an unstructured approach that uses a blend of Bt and non-Bt corn seed (i.e., refuge in a bag, RIB). Theoretically, pyramids require less refuge (Gould et al. 2006; Zhao et al. 2003), and EPA has allowed reduced refuge for some pyramided corn (Coons 2009). However, modeling suggests larval biology and behavior, such as dispersal between Bt and non-Bt corn, may compromise seed blends as a resistance management strategy (e.g., Goldstein et al. 2010; Razze et al. 2011; Yang et al. 2014). ECB neonate dispersal is greater on Bt corn compared with non-Bt corn (Razze and Mason 2012). Similarly, larvae of several lepidopteran species (e.g., ECB, WBC, FAW) (Paula-Moraes et al., 2012, Pannuti et al, 2016) exhibit more plant-to-plant movement than was expected, particularly in early instars.
Annual assessments of the susceptibility of target pest species such as ECB and FAW has involved diagnostic bioassays (Marçon et al. 2000; Siegfried et al. 2007), F1 and F2 screening approaches (Vélez et al. 2013a,b; Siegfried et al. 2014), and more recently the use of DNA-based genotyping for known FAW resistance alleles (Banerjee et al. 2017, Tandy et al. 2023) to isolate resistance alleles and assess frequencies. In instances where resistance has been detected, specific research to identify inheritance patterns, fitness costs (Crespo et al. 2010; Pereira et al. 2011; Vélez et al. 2013a; Jakka et al. 2014; Petzold-Maxwell et al. 2014), and molecular mechanisms of resistance has been conducted (Crespo et al. 2011; Coates et al. 2011, 2013a; Coates and Siegfried 2015; Jakka et al. 2016; Banerjee et al. 2017, 2022; Abdelgaffar et al. 2019). Bioassays with Bt toxins using standard methods involving surface treatment of artificial diet with toxin preparations (Marçon et al. 1999) have provided consistent assessments of susceptibility for more than a decade (Siegfried et al. 2007; Siegfried and Hellmich 2012; Siegfried and Spencer 2012). Biochemical, molecular, and genomic tools have been developed to assess mechanisms of resistance (Coates et al. 2011; Crespo et al. 2011; Jakka et al. 2016) and identify resistance genes and alleles (Banerjee et al. 2017, 2022). Resistance to corn producing the Cry1F insecticidal protein has been linked to distinct mutations in the same gene, allowing the development of multiplexed targeted sequencing to detect known and new candidate resistance alleles in FAW populations (Tandy et al. 2023). Resistance to Cry1F was shown to inhibit the increased flight tendency of FAW in flight mill studies when exposed to an active Bt toxin (De Bortoli et al. 2024). Altered Vip3Aa binding to midgut receptors has been elucidated as a mechanism of resistance in CEW from Texas (Kerns et al. 2023), yet the altered receptor is not known yet. Both CEW (Dively et al. 2016; Bilbo et al. 2019) and FAW (Huang et al. 2014; Li et al. 2016; Jakka et al. 2014, 2015) show resistance to more than one Bt toxin, which reduces producer ability to control associated damage. WBC resistance to Cry1F toxin has been documented in Ontario, Canada (Smith et al. 2017). NCR is a perennial pest in much of the Corn Belt and has evolved resistance to multiple Bt toxins (Calles-Torrez et al. 2019).
Resistance to Cry3Bb1 corn was initially documented in Iowa (Gassmann et al. 2011, 2012). Cry3Bb1 resistant WCR were shown to be cross-resistant to mCry3A (Gassmann et al. 2014) and eCry3.1Ab (Jakka et al. 2016). More recently, WCR resistance to Cry34/35Ab1 was documented in Iowa (Gassmann et al. 2016) and Minnesota (Ludwick et al. 2017), which exhibits no cross-resistance to Cry3Bb1 (Zhao et al. 2016). Analysis of WCR collected from other regions confirmed resistance to “Cry3” (Cry3Bb1, mCry3A, and eCry3.1Ab) corn across the Midwest (Wangila et al. 2015; Zukoff et al. 2016; Schrader et al. 2017; Reinders et al. 2018). A WCR resistance management strategy based on the “high dose/refuge” concept has been shown to be ineffective for currently available rootworm Bt events which do not achieve a high dose of Bt toxin (Andow et al. 2016). Because commercially available rootworm events are not high dose, this allows for some larval survival and adult emergence (Hibbard et al. 2011; Frank et al. 2015; Hitchon et al. 2015; Keweshan et al. 2015; Head et al. 2017). Limited data have been collected on fitness of adults emerging from Bt events. To date, few WCR fitness costs have been associated with resistance to Bt events (Oswald et al. 2012; Meihls et al. 2012; Hoffmann et al. 2015; Ingber and Gassmann 2015). Cry3Bb1 resistance persists over time in the field (Gassmann et al. 2014; Wangila and Meinke 2017) and when frequency of Cry3Bb1-resistant individuals is high in a population, the average delay in adult emergence from Cry3Bb1 commonly reported in the literature (e.g., Hitchon et al. 2015; Keweshan et al. 2015) can be substantially reduced (Wangila and Meinke 2017). A single QTL on LG8 was linked to Cry3Bb1 resistance in WCR and screening of field populations with markers within the QTL were modestly predictive of resistance (Flagel et al. 2015).
A series of experiments have been conducted to develop an improved, standardized artificial diet for western corn rootworm that can be publicly available. An initial diet (WCRMO-1) that improved upon an older published WCR diet (Pleau et al. 2002) was published by Huynh et al (2017). WCR development and survival were significantly different when proprietary WCR diets, the older published diet (Pleau et al. 2002), and the new diet were compared (Meihls et al. 2018). Several improvements have been made to the WCRMO-1 diet (Huynh et al. 2019a) including the removal of corn root powder (WCRMO-2, Huynh et al. 2019b). An artificial diet for NCR has also recently been developed (Huynh et al. 2019c). The WCRMO-1 diet is compatible with rootworm Bt proteins and can differentiate resistant WCR colonies from susceptible colonies (Ludwick et al., 2018). These new diets will enable standardization of diet-overlay bioassays across labs to monitor for WCR or NCR resistance.
As noted above, questions have arisen concerning larval movement and the efficacy of seed blends in slowing the development of resistance. Associated with movement are the consequential effects of larval feeding on corn ears that may result in cross-pollination between Bt corn and non-Bt corn (e.g., Chilicutt and Tabashnik 2004; Burkness et al. 2011; Burkness and Hutchison 2012; Kang et al. 2012; Yang et al. 2014). Reduced mortality was observed by ECB larvae feeding on kernels of Bt corn expressing Cry1Ab toxin (Burkness et al. 2011), and recent studies have indicated that some species (e.g., CEW) may exhibit differential feeding on kernels expressing different or no Bt toxin. Differential expression of Bt toxins in corn ears has been suggested to affect the evolution of resistance in lepidopterans that feed primarily in the ear (e.g., CRW, WBC) (Burkness et al. 2011; Onstad et al. 2011). Using purple-seeded corn as a marker, studies (Dively et al. 2019) showed that outcrossing of pollen from the refuge plant in a seed blend averaged <1% in neighboring Bt ears but was much greater from Bt pollen in the opposite direction, averaging 18.6% in refuge ears. Laboratory bioassays further showed that consumption of a meridic diet containing 6% refuge kernel tissue collected from a 95:5% seed blend field caused significant larval growth inhibition of CEW and ECB larvae.
Objective 4: Employ traditional and innovative delivery methods to disseminate information related to sustainable management of corn arthropod pests to farmers, industry, colleagues, agricultural professionals, and policy makers.
NC246 members have been active in translating their research into actionable management recommendations for corn farmers throughout North America. Some multi-state highlights include a multi-region virtual scout school hosted online by Crop Protection Network, and regional survey maps of moth and corn rootworm trapping networks hosted by IPM-Pipe and Iowa State University Extension, respectively. A group of corn entomologists (consisting mostly of NC246 members) has published annual estimates of invertebrate losses in corn since 2021. (Reisig et al. 2022, 2023, 2024).
Objectives
-
Investigate the ecology, biology, evolution, genetics, genomics, and behavior of corn arthropods.
Comments: 1 A. Characterize the dispersal of arthropod corn pests and assess its implications for Integrated Pest Management (IPM) and Insect Resistance Management (IRM). 1B. Improve rearing and bioassay methodologies, including artificial diets. 1C. Investigate ecology and gene flow among pest species/races/strains/biotypes. 1D. Assess the effects of Bt seed blend refuge on lepidopteran pest species. 1E. Develop genomics tools for key corn pests, including genome and transcriptome sequences, genetic markers, and quantitative trait locus (QTL) maps of important traits. -
Develop and assess IPM systems for the arthropod complex in corn.
Comments: 2A. Coordinate early detection and mitigation networks for invasive species. 2B. Document the performance of chemical insecticides, Bt toxins, RNA-interference molecules, biological control agents, and conservation of natural enemies. 2C. Document the need and effects of management tools on non-target organisms. 2D. Evaluate the adoption of IPM/IRM management practices by corn farmers. -
Characterize and monitor pest resistance and assess IRM and mitigation strategies.
Comments: 3A. Establish resistance monitoring networks for corn pests. 3B. Characterize the geographic range, demographics, and nature of arthropod pest resistance to insecticidal agents. 3C. Identify genes, genome regions, and cellular and biochemical mechanisms involved in resistance to insecticidal agents. 3D. Develop region-specific bioeconomic models to assess refuge and IPM strategies for managing pest resistance to Bt corn. 3E. Use data generated in the above efforts to develop and evaluate IPM and IRM strategies. -
Employ traditional and innovative delivery methods to disseminate information related to sustainable management of corn arthropod pests to farmers, industry, colleagues, agricultural professionals, and policymakers
Methods
Obj. 1. Ecology, biology, evolution, genetics, genomics, and behavior of corn arthropods.
1A) Characterize dispersal of arthropod corn pests and assess its implications for IPM and IRM.
A multi-state study led by FL will map the seasonal migration of lepidopterans, such as FAW, into the central and eastern U.S. using pheromone traps and molecular genetic markers, including Bt-resistance genes. Several states () and six Canadian provinces will collaborate in the Great Lakes and Maritimes Pest Monitoring Network where pheromone traps will monitor shifts in the distribution and phenology of important lepidopteran corn pests. The network will be used in conjunction with sentinel plots and pest collections for bioassays to determine resistance development. Several states (DE, MD, and PA) will conduct pheromone monitoring to assess the spread of WBC into the mid-Atlantic region and persistence in endemic regions of NE. In MD and many participating states, CEW and FAW will also be monitored at multiple sites, with data entered into the EDDMaps system. In addition, FL will also lead studies of migration of CEW, including southward reverse migration at the end of the crop season. To better understand the distribution of ECB strains as well as their phenology, pheromone traps will be deployed in numerous states (CT, DE, IA, MD, MN, MO, NC, OH, SC, SD, and WI) and Canadian provinces . Long-range movement of ECB ecotypes will be investigated with adult flights mills. ECB larval movement among Bt and non-Bt plants will be investigated to understand resistance development potential and management considerations. Western and northern corn rootworm adult population densities will be monitored through a multi-state/province (IA, IL, IN, KS, MB, MN, ND, NE, OH, ON, SD, and WI ) network of farmer and crop advisor volunteers.
1B) Improve rearing and bioassay methodologies, including artificial diets.
USDA-ARS (MO) will collaborate with Frontier Scientific to refine commercially available diet formulations for WCR and NCR larvae. Analysis of metabolites contained within corn roots at different time points will aid in understanding factors important for growth and development of WCR and NCR. Using this information, an improved artificial diet will be developed, and the potential for continuous rearing using artificial diets will be investigated. IA, NE, and Canada will investigate WBC diet and rearing conditions to improve laboratory fecundity. Corn tissue-incorporated diets will be developed for use in bioassays diagnostic of field-evolved resistance and to determine the effects of tissue-specific feeding on larval growth, development, and adult fecundity. The use of non-commercial single-batch Bt insecticidal proteins will be explored in efforts to standardize bioassays.
1C) Investigate ecology and gene flow among pest species/races/strains/biotypes.
IA and TN will lead studies, in collaboration with other states, on the characterization of pheromone and diapause variants of ECB. Temporal genetic variation will be assessed in geographic regions with sympatric ECB voltinism ecotypes using SNP markers (Coates et al. 2013b), including those in genes linked to variation in Pdd (Kozak et al. 2019), female pheromone production (Lassance et al. 2010), and corresponding male response (Unbehend et al. 2021). Estimates of genetic differentiation among previously genotyped samples of these strains (Coates et al. 2019) will be re-evaluated along with additional field-collected samples. The structure of an inversion on the Z chromosome of ECB will be characterized by generating and aligning chromosome level genome assemblies for ECB with and without the inversion. The role this structural change has on the evolution and expression of genes will be assessed, as will the degree to which structural genome change might reinforce population divergence. A project led by TN will develop next generation sequencing to determine dispersal among FAW populations of Bt resistance alleles and their frequency. Genomic resources generated from FAW collections by multiple states will be used to determine migratory movement of overwintering populations. This information will elucidate gene flow between FAW populations and corresponding spread of Bt resistance alleles and facilitate the development of predictive models for the movement of resistant FAW. The frequency of the H. armigera-specific CYP337B3 in CEW will be monitored by use of a genetic marker to screen annual collections made across North America. Additionally, the time of introduction will be retrospectively estimated by screening historical samples. The level of larval CEW pyrethroid resistance conferred by CYP337B3 will also be assessed using dose-response bioassays from field-collected insects in IA and CO.
1D) Assess the effects of Bt seed blend refuge on lepidopteran pest species.
A study led by NC along with NC246 members will assess the impacts of refuge structure on male and female fitness and mating success. If mating is impacted by seed-blend versus structured refuge, a stochastic, spatially explicit model of resistance in CEW to Bt toxins in the southern U.S. will be developed. WBC larval movement and survival in blended refuges with Vip3A corn will be evaluated in field trials in NE and Canada and laboratory exposures conducted in IA using differing amounts of Vip3A, non-Bt and cross-pollinated kernels incorporated into artificial diets. Additionally, diet bioassays with incorporated corn tissues will be used to determine the effects of different tissues on WBC larval feeding, growth, and development.
1E) Develop genomics tools for key corn pests, including genome and transcriptome sequences, genetic markers, and QTL maps of important traits.
The WCR genome will be used to investigate the genetic basis of Bt resistance. Based on QTL mapping of Cry3Bb1 and Gpp34/Tpp35Ab1 resistance in WCR and mapping of trait loci in populations, candidate resistance genes will be validated using RNAi as performed previously for ABC transporters (Adedipe et al. 2019) and/or by CRISPR knockouts. These candidate genes will be investigated in mCry3A and eCry3.1Ab-resistant strains maintained at USDA-ARS, and a Cry3Bb1-resistant strain at Iowa State. Genome and transcriptome sequence assemblies will be generated for SCR and NCR, and comparison of chemosensory gene repertoire made with those in the WCR genome to understand the evolution of host recognition. ECB genomes will be assembled for ECB ecotypes differing in presence of an inversion on the Z chromosome (Kozak et al. 2017) and used to define breakpoints and development of genetic markers to identify prevalence in field populations. ECB population genomic and pedigree studies will be used to investigate roles of per and pdfr genes in determining Pdd (Kozak et al. 2019) and refine interactions with pheromone strains at the population level (Coates et al. 2019). Markers will be added to two ECB QTL previously linked to Cry1Ab resistance (Coates et al. 2013b), and a single QTL containing the ABCC2 gene linked to Cry1F resistance (Coates and Siegfried 2015; Farhan et al. 2023). These genetic markers will be developed to estimate gene flow and track putative Bt resistance genes in field populations. In CEW, genomic studies will be conducted to better understand the genes involved in resistance to Cry1 and Cry2Ab2 and develop markers to screen field populations for resistance. Field-collected FAW, CEW, and ECB samples from multiple states will be used for isolation of genomic DNA, which will then be used for targeted and/or whole-genome sequencing at TN as described elsewhere (Tandy et al. 2023; Schlum et al. 2021). Samples from susceptible and resistant strains of FAW, CEW, and ECB from LA, MD, MN, OH, and TX will be sent to TN for mechanistic analysis of resistance to Cry1, Cry2, and Vip3Aa proteins in Bt corn. Analyses include testing processing of the proteins by midgut fluids and binding of the purified proteins to the midgut epithelium using established methods (Kerns et al. 2023; Abdelgaffar et al. 2019). Genomic and transcriptome profiling and bulk segregant analysis of genetic variation associated with the resistant phenotypes allow the identification of genetic loci and candidate resistance genes. Information from these studies will be used to develop multiplexed targeted sequencing tools for high throughput monitoring for resistance to Bt corn in FAW, ECB, and CEW. Continued genotyping using available targeted sequencing tools (Tandy et al. 2023) will allow the estimation of resistance allele frequency and subsequent risks of resistance evolution.
Objective 2: Develop and assess IPM systems for the arthropod complex in corn.
2A) Coordinate early detection and mitigation networks for invasive species.
Multistate pheromone trapping will continue in collaboration with USDA APHIS for early detection of invasive H. armigera and its introgression with the native H. zea will be examined using a genetic marker for CYP337B3. The performance and possible efficacy decline of management tools in IPM programs will also be documented.
2B) Document the performance of chemical insecticides, Bt toxins, RNA interference molecules, biological control agents, and conservation of natural enemies.
Collaborative work across many states will continue to document the annual performance of Bt commercial traits and insecticides broadly adopted to manage pests (particularly NCR, WCR,ECB, and CEW) in corn production systems. This documentation will be conducted with the multistate collaboration of pest population collections, the performance of toxicological bioassays in designated laboratories, and the annual establishment of sentinel plots in different corn-producing regions. Innovative technologies such as biopesticides will also continue to be explored as components to be adopted in IPM programs. Field efficacy evaluations of Bt traits for corn earworm control will be combined across southeastern states (GA, NC, and SC) to detect changes in performance over time. The study of entomopathogenic nematodes applied as persistent biological control agents will continue to examine their persistence and lethality to corn rootworms and other pest/beneficial species, including a multi-state field assessment in IA, IL, and NE. A multi-state effort (DE, MD, OH, PA, TN, and VA) will assess slug populations in agricultural fields. NC246 members will contribute to a nationwide estimate of losses in corn production due to invertebrate pests published annually through Crop Protection Network (many states; coordinated by Dominic Reisig, North Carolina State University).
2C) Document the need and effects of management tools on non-target organisms.
The effect of seed treatment on pollinators, natural enemies, and detritivores will continue to be studied by survey, field studies, and dose-response studies in the laboratory to characterize susceptibility, routes of exposure, and sublethal effects. A multi-state (IL, NE, and TX) project will examine the non-target impacts of entomopathogenic nematodes applied for rootworm control. A second multi-state project (IN and PA) will examine the effects of cover cropping in no-till fields (practices that are increasing in prevalence within the Corn Belt) on the abundance of and ecosystem services provided by, a key understudied group of natural enemies (ants) that can potentially prey upon a wide range of corn pests but are relatively ignored in the published literature.
2D) Evaluate the adoption of IPM/IRM management practices by corn farmers.
The impact of the availability of management tools in farmers’ choice in IPM/IRM program adoption will continue by surveying seed dealers/cooperators and comparing prices of Bt corn hybrids and corresponding pest incidence in different regions. This will be combined with economic analyses and measurements of willingness to plant non-Bt refuge hybrids before and after a presentation of the economic analysis.
Objective 3: Characterize and monitor pest resistance and assess IRM and mitigation strategies.
3A) Establish resistance monitoring networks for corn pests.
Diagnostic bioassays of collections from across the Corn Belt will be conducted annually to assess the susceptibility of target pest species (e.g. WCR, ECB, FAW, and WBC) to Bt toxins and insecticides using established methods (Marçon et al. 1999, 2000; Siegfried et al. 2007). Field visits will be conducted to identify suspected cases of resistance in response to concerns by farmers and/or crop consultants. A gene check strip will be used to confirm the type of Bt corn planted in the field, feeding injury evaluated, and arthropods collected to generate populations for bioassays. Multiplexed targeted sequencing (Tandy et al. 2023) will be adapted to detect multiple resistance genes as a high throughput tool for resistance screening. For corn rootworm, single-plant bioassays, following Gassmann et al. (2014), will be applied to measure the level of resistance to Bt events. Continuation of a multistate network (coordinated by MD) of sweet corn sentinel plots will monitor for further lepidopteran resistance development to the Bt toxins and determine whether regional control efficacy differences exist among the toxins expressed in Bt sweet corn. The major focus of the monitoring network will be to detect changes in CEW susceptibility to the Vip3Aa toxin. Sentinel plots will also be used to monitor for resistance in ECB in the U.S. and Canada beginning in 2024. The findings will be used to investigate the utility of the sentinel plot monitoring approach and improve the design of resistance monitoring for Bt crops.
3B) Characterize the geographic range, demographics, and nature of arthropod pest resistance to insecticidal agents.
Inheritance experiments will involve reciprocal crosses of resistant and susceptible parental populations to establish an F1 generation and assess dominance based on the response curve of heterozygous individuals. Backcrosses of the F1 heterozygotes to one of the parental strains will be used to estimate the number of genetic factors that contribute to resistance. A variety of biochemical, molecular, and genomic tools will be used to assess mechanisms of resistance (Coates et al. 2011, 2013a; Crespo et al. 2011; Jakka et al. 2016; Banerjee et al. 2017, 2022).
3C) Identify genes, genome regions, and cellular and biochemical mechanisms involved in resistance to insecticidal agents.
Genetic markers will be added to ECB genetic maps previously identifying a QTL for Cry1F resistance (Coates and Siegfried 2015). Differential transcript expression studies will be conducted between Bt resistant and susceptible ECB, or Bt exposed and unexposed WCR and WBC. MD will use genomic approaches to determine the number, location, effect sizes, and dominance of quantitative trait loci (QTL) associated with CEW resistance to Cry1Ab as well as Cry1A.105+Cry2Ab2 using field-collected populations. In TN, assays with radiolabeled Cry and Vip3Aa proteins will be used to test for reduced binding in resistant populations. Candidate resistance genes will be identified from bulk segregant analyses (BSA) and transcriptome profiling by RNAseq. Mutations and indels linked with resistance will be used to develop a target panel for multiplexed targeted sequencing to examine the frequency of resistance alleles. Gene editing by CRISPR/Cas9 and expression in heterologous systems will be used as functional tools to establish resistance genes.
3D) Develop region-specific bioeconomic models to assess strategies for managing pest resistance to Bt corn.
Small plot experiments will be conducted (CT, IL, MO, ON, and SD) to evaluate the yield impacts of ECB injury to modern corn hybrids. These data will be used to update economic models for ECB IRM. Existing bioeconomic models will be extended to incorporate multiple insect targets with multiple modes of actions for each target and to examine both IRM and mitigation policies. Model extensions will include assessing the impacts of pyramiding and stacking of Bt corn traits for below-ground and above-ground pests and more sophisticated modeling of hybrid selection decision-making behavior using agent-based modeling. Key topics to examine will include mitigation policies to respond to the development of resistance (e.g., localized Bt seed bans, areawide spray applications, Bt seed taxes, increased refuge), and impacts of using homogeneous trait packages when pressure from the target pests varies regionally. MN and WI will lead model development, working with committee members to parameterize the yield loss and pest population dynamics models with regionally appropriate values.
3E) Use data generated in the above efforts to develop and evaluate IPM and IRM strategies.
The results for the above models will include economic assessments to project the social surplus generated by the alternative IRM strategies for resistance management and mitigation. These measures will largely be based on farmer returns, accounting for major cost categories related to insect management, including technology fees for seed and insecticide costs, as well as taxes or other revenue collected. The purpose of these social surplus metrics is to provide a measure for comparing and ranking alternative management and mitigation strategies. MN and WI will lead efforts in these analyses.
Obj. 4. Employ traditional and innovative delivery methods to disseminate information related to the sustainable management of corn arthropod pests to farmers, industry, colleagues, agricultural professionals, and policymakers.
Traditional in-person and virtual extension meetings and educational programs will be held with producers, industry, consultants, and regulators to discuss findings and share information and ideas to enhance policy and regulatory decisions compatible with the economic, environmental, health, and social needs of both the agricultural and non-agricultural communities. Newsletters, traditional extension materials, web pages, blogs, AgTwitter and other social media, videos, interactive training modules, scientific publications, surveys and focus groups, and position statements will be used to disseminate information to the agricultural and public sectors. (DE, FL, GA, IA, IL, IN, KS, KY, MD, MI, MN, MO, NC, ND, NE, OH, ON, PA, SC, SD, TX).
Measurement of Progress and Results
Outputs
- 1.Data and refereed publications identifying new instances of resistance and/or resistance mechanisms to biotech traits and insecticides in NCR , and WCR, and CEW, ECB, FAW, and WBC.
- 2.Regional best management practice recommendations for key pests of corn published in journals and/or extension bulletins.
- 3.Data and refereed publications characterizing the migration patterns of FAW, CEW, and other migratory pests.
- 4.Reports on the adoption and economic impact of IPM and IRM strategies.
Outcomes or Projected Impacts
- 1.Instances of resistance to important crop protection technologies are identified through coordinated research informed by field observations across states and regions, and this information is provided to corn farmers and other stakeholders to allow more effective pest management.
- 2.Updated IPM recommendations that increase farmer profitability and improve the environmental footprint of corn production.
- 3.Insect resistance management policies and recommendations that reduce the risk of arthropod pest resistance and improve stewardship of crop protection technologies.
- 4.Advances in our understanding of pest ecology, behavior, movement, rearing, and genetics will be used to improve research approaches and models, increasing our capacity to respond to management issues and develop innovative IPM practices.
Milestones
(2025):Initiation of new project (October). Coordination of research teams and initiation of research projects for Objectives 1, 2, and 3.(2026):Implementation of field and laboratory research for Objectives 1, 2, and 3. Development of extension and outreach materials as appropriate under Objective 4. New projects in response to previous results may be developed.
(2027):Ongoing field and laboratory research for Objectives 1, 2, and 3, and dissemination of recommendations through Objective 4. New projects in response to previous results may be developed.
(2028):Ongoing field and laboratory research for Objectives 1, 2, and 3, and dissemination of recommendations through Objective 4. New projects in response to previous results may be developed.
(2029):Ongoing field and laboratory research for Objectives 1, 2, and 3, and dissemination of recommendations through Objective 4. Review of progress during the current project and preparation of a new multi-state proposal for submission in Fall 2029. New projects in response to previous results may be developed.
(2030):Completion of field and laboratory research for Objectives 1, 2, and 3, and dissemination of recommendations through Objective 4. Project ends in September 2030, with the goal of beginning a new multi-state project in October 2030.
Projected Participation
View Appendix E: ParticipationOutreach Plan
Members of NC246 are involved in a wide variety of outreach efforts related to the sustainable management of corn arthropod pests. They recommend IPM-based approaches that exploit multiple strategies for suppressing pests to avoid growers' reliance on any one practice or tactic, and associate rapid selection for pest resistance. Many committee members interact regularly with farmers, media, government agencies, state commodity boards, and other Ag professionals regarding IPM, IRM, and pest biology. In addition to helping farmers and the public understand IRM programs, committee members with extension appointments will provide guidance about how transgenic crops and IRM programs fit into a larger field corn IPM program. Members provide growers with specific, science-based guidance to make informed decisions about the management of corn insect pests.
Traditional in-person and electronic extension education will be held for producers, industry, consultants, and regulators to discuss findings and share information and ideas to enhance policy and regulatory decisions compatible with the economic, environmental, health, and social needs of both the agricultural and non-agricultural communities. Feedback from growers will be used to help direct research activities of the committee. Newsletters, traditional extension materials, Web pages, Blogs, Ag Twitter, and other social media, videos, interactive training modules, scientific publications, surveys and focus groups, and position statements will be used to disseminate information to the agricultural and public sectors. These efforts are encompassed in Objective 4. NC246 activities will also focus on expanding the land-grant university research and Extension presence and leadership roles in the traditional and electronic media arenas to provide unbiased science-based IPM and IRM decision support to clientele.
Organization/Governance
The project will operate following the "Guidelines for Multistate Research Activities" document developed by the State Agricultural Experiment Station Directors in cooperation with USDA NIFA and ESCOP, available on the NIMSS website.
The project will be administered by a Technical Committee consisting of all Appendix E participants of the project; all are eligible for office, regardless of sponsoring agency affiliation or the number of members per SAES. An Executive Committee will consist of the Past Chair, Chair, Vice Chair, and Secretary (each serving 1-year terms), as well as the Administrative Advisor. The Executive Committee will conduct business during and between annual meetings (e.g. annual reports, NIMSS entries, project rewrites). Other important standing committees include Local Arrangements and Nominating Committee, the membership of which will vary from year to year.
An annual meeting of the full Technical Committee will be held to summarize and critically evaluate progress, analyze results, and plan future activities, reports, and publications. The Executive Committee, in consultation with Local Arrangements, the Technical Committee, and with the concurrence of the Administrative Advisor, notifies the Technical Committee members of the time and place of meetings, prepares the agenda, and presides at meetings. The Administrative Advisor authorizes the annual meeting 90-120 days in advance. The Executive Committee, in coordination with Local Arrangements, will be responsible for organizing, setting the agenda, and presiding over an annual Open Meeting with Industry, EPA, and other invited guests, which will be scheduled as part of the regular annual Technical Committee meeting.
An incoming Secretary will be nominated by the Nominating Committee and voted upon by the full Technical Committee at the annual meeting. The Secretary will serve a 1-year term culminating at the close of the annual meeting. The Secretary will rotate into the Vice Chair position after the first year, then Chair, and finally Past Chair. Therefore, election as Secretary implies a 4-year commitment as an Executive Committee officer, although alterations to this pattern can be addressed as needed.
Literature Cited
Abdelgaffar, H. M., Oppert, C., Sun, X., Monserrate, J., and Jurat-Fuentes, J.L. 2019. Differential heliothine susceptibility to Cry1Ac associated with gut proteolytic activity. Pestic. Biochem. Physiol. 153: 1-8.
Adedipe, F., Grubbs, N., Coates, B., Wiegmmann, B., and Lorenzen, M. 2019. Structural and functional insights into the Diabrotica virgifera virgiera ATP-binding cassette transporter gene family. BMC Genomics. 20: 899.
Andow, D.A., Pueppke, S.G., Schaafsma, A.W., Gassmann, A.J., Sappington, T.W., Meinke, L.J., Mitchell, P.D., Hurley, T.M., Hellmich, R.L., and Porter, R.P. 2016. Early detection and mitigation of resistance to Bt maize by western corn rootworm (Coleoptera: Chrysomelidae). J. Econ. Entomol. 109: 1-12.
Arnemann, J.A., James, W.J., Walsh, T.K., Guedes, J.V.C., Smagghe, G., Castiglioni, E., and Tay, W.T. 2016. Mitochondrial DNA COI characterization of Helicoverpa armigera (Lepidoptera: Noctuidae) from Paraguay and Uruguay. Genet. Mol. Res. 15.
Arnemann, J.A., Roxburgh, S., Walsh, T., Guedes, J., Gordon, K., Smagghe, G., and Tay, W.T. 2019. Multiple incursion pathways for Helicoverpa armigera in Brazil show its genetic diversity spreading in a connected world. Sci. Rep. 9: 19380.
Ball, H.J., and Weekman, G.T. 1962. Insecticide resistance in the adult western corn rootworm in Nebraska. J. Econ. Entomol. 55: 439-441.
Ball, H.J., and Weekman, G.T. 1963. Differential resistance of corn rootworms to insecticides in Nebraska and adjoining states. J. Econ. Entomol. 56: 553-555.
Banerjee, R., De Bortoli, C.P., Huang, F., Lamour, K., Meagher, R., Buntin, D., Ni, X., Reay-Jones, F.P.F., Stewart, S., and Jurat-Fuentes, J.L. 2022. Large genomic deletion linked to field-evolved resistance to Cry1F corn in fall armyworm (Spodoptera frugiperda) from Florida. Sci. Rep. 12: 13580.
Banerjee, R., Hasler, J., Meagher, R., Nagoshi, R., Hietala, L., Huang, F., Narva, K., and Jurat-Fuentes, J.L. 2017. Mechanism and DNA-based detection of field-evolved resistance to transgenic Bt corn in fall armyworm (Spodoptera frugiperda). Sci. Rep. 7: 10877.
Benowitz, K.M., Allan, C.W., Degain, B.A., Li, X., Fabrick, J.A., Tabashnik, B.E., Carrière, Y. and Matzkin, L.M., 2022. Novel genetic basis of resistance to Bt toxin Cry1Ac in Helicoverpa zea. Genetics. 221(1): p.iyac037.
Bilbo, T.R., Reay-Jones, F.P.F., Reisig, D.D. and Greene, J.K. 2019. Susceptibility of corn earworm (Lepidoptera: Noctuidae) to Cry1A. 105 and Cry2Ab2 in North and South Carolina. J. Econ. Entomol. 112(4): 845-1857.
Bilbo, T.R., Reay-Jones, F.P.F., Reisig, D.D., Musser, F.R., and Greene, J.K. 2018. Effects of bt corn on the development and fecundity of corn earworm (Lepidoptera: Noctuidae). J. Econ. Entomol. 111(5): 2233-2241.
Brévault, T., Tabashnik, B.E., and Carrière, Y. 2015. A seed mixture increases dominance of resistance to Bt cotton in Helicoverpa zea. Sci. Rep. doi: 10.1038/srep09807.
Burd, A.D., Gould, F., Bradley, J.R., Van Duyn, J.W. and Moar, W.J. 2003. Estimated frequency of nonrecessive Bt resistance genes in bollworm, Helicoverpa zea (Boddie) (Lepidoptera: Noctuidae) in eastern North Carolina. J. Econ. Entomol. 96(1): 137-142.
Burkness, E.C., and Hutchison, W.D. 2012. Bt pollen dispersal and Bt kernel mosaics: Integrity of non-Bt refugia for lepidopteran resistance management in maize. J. Econ. Entomol. 105: 1477-1487.
Burkness, E.C., O'Rourke, P.K., and Hutchison, W.D. 2011. Cross-pollination of nontransgenic corn ears with transgenic Bt corn: Efficacy against lepidopteran pests and implications for resistance management. J. Econ. Entomol. 104: 1476-1479.
Bryant, T., Greene, J.K., and Reay-Jones, F.P.F. 2023. Within-field spatial patterns of Euschistus servus and Nezara viridula (Hemiptera: Pentatomidae) in field corn. Environ. Entomol. 52: 709-721.
Calles-Torrez, V., Knodel, J.J., Boetel, M.A., French, B.W., Fuller, B.W. and Ransom, J.K. 2019. Field-evolved resistance of northern and western corn rootworm (Coleoptera: Chrysomelidae) populations to corn hybrids expressing single and pyramided Cry3Bb1 and Cry34/35Ab1 Bt proteins in North Dakota. J. Econ. Entomol. 112(4): 1875-1886.
Chilcutt, C.F., and Tabashnik, B.E. 2004. Contamination of refuges by Bacillus thuringiensis toxin genes from transgenic maize. Proc. Natl. Acad. Sci. U.S.A. 101: 7526-7529.
Coates, B.S., Kozak, G.M., Kim, K., Sun, J., Wang, Y., Fleischer, S.J., Dopman, E.B., Sappington, T.W. 2019. Influence of host plant, geography and pheromone strain on genomic differentiation in sympatric populations of Ostrinia nubilalis. Mol. Ecol. 28(19):4439-4452. https://doi.org/10.1111/mec.15234.
Coates, B.S., Alves, A.P., Wang, H. Walden, K.K.O., French, B.W., Miller, N.J., Abel, C.A., Robertson, H.M., Sappington, T.W., and Siegfried, B.D. 2012. Distribution of genes and repetitive elements in the Diabrotica virgifera virgifera genome estimated using BAC sequencing. BioMed Res. Int. 604076. https://doi.org/10.1155/2012/604076
Coates, B.S., Deleury, E., Gassmann, A.J., Hibbard, B.E., Meinke, L.J., Miller, N.J., Petzold-Maxwell, J., French, B.W., Sappington, T.W., Siegfried, B.D., and Guillemaud, T. 2021. Up-regulation of apoptotic- and cell survival-related gene pathways following exposures of western corn rootworm to B. thuringiensis crystalline pesticidal proteins in transgenic maize roots. BMC Genomics. 22: 639.
Coates, B.S., Johnson, H., Kim, K.S., Hellmich, R.L., Abel, C.A., Mason, C., and Sappington, T.W. 2013b. Frequency of hybridization between Ostrinia nubilalis E- and Z-pheromone races in regions of sympatry within the United States. Ecol. Evol. 3: 2459-2470.
Coates, B.S., and Siegfried, B.D. 2015. Linkage of an ABCC transporter to a single QTL that controls Ostrinia nubilalis larval resistance to the Bacillus thuringiensis Cry1Fa toxin. Ins. Biochem. Mol. Biol. 63: 86-96.
Coates, B.S., Sumerford, D.V. and Lewis, L.C. 2008. Segregation of European corn borer, Ostrinia nubilalis, aminopeptidase 1, cadherin, and bre5-like alleles, from a colony resistant to Bacillus thuringiensis Cry1Ab toxins, are not associated with F2 larval weights when fed a diet containing Cry1Ab. J. Insect Sci. 8(1): 21.
Coates, B.S., Sumerford, D.V., Lopez, M.D., Wang, H., Fraser, L.M., Kroemer, J.A., Spencer, T., Kim, K.S., Abel, C.A., Hellmich, R.L., and Siegfried, B.D. 2011. A single major QTL controls expression of larval Cry1F resistance trait in Ostrinia nubilalis (Lepidoptera: Crambidae) and is independent of midgut receptor genes. Genetica. 139: 961-972.
Coates, B.S., Sumerford, D.V., Miller, N.J., Kim, K.S., Sappington, T.W., Siegfried, B.D., and Lewis, L.C. 2009. Comparative performance of single nucleotide polymorphism and microsatellite markers for population genetic analysis. J. Hered. 100(5): 556-564.
Coates, B.S., Sumerford, D.V., Siegfried, B.D., Hellmich, R.L., and Abel, C.A. 2013a. Unlinked genetic loci control the reduced transcription of aminopeptidase N1 and 3 in the European corn borer and determine tolerance to Bacillus thuringiensis Cry1Ab toxin. Insect Biochem. Mol. Biol. 43: 1152-1160.
Coates, B.S., Walden, K.K., Lata, D., Vellichirammal, N.N., Mitchell, R.F., Andersson, M.N., McKay, R., Lorenzen, M.D., Grubbs, N., Wang, Y.H. and Han, J. 2023. A draft Diabrotica virgifera virgifera genome: insights into control and host plant adaption by a major maize pest insect. BMC Genomics. 24(1): 19.
Coons, R. 2009. U.S., Canadian regulators OK SmartStax, reduce refuge requirements, Chemical Week. pp. 1.
Crespo, A.L.B., Rodrigo-Simon, A., Siqueira, H.A.A., Pereira, E.J.G., Ferre, J., and Siegfried, B.D. 2011. Cross-resistance and mechanism of resistance to Cry1Ab toxin from Bacillus thuringiensis in a field-derived strain of European corn borer, Ostrinia nubilalis. J. Invert. Pathol. 107: 185-192.
Crespo, A.L.B., Spencer, T., Tan, S.Y., and Siegfried, B.D. 2010. Fitness costs of Cry1Ab resistance in a field-derived strain of Ostrinia nubilalis (Lepidoptera: Crambidae). J. Econ. Entomol. 103: 1386-1393.
De Bortoli, C.P., Santos, R.F., Assirati, G.J., Sun, X., Hietala, L., and Jurat-Fuentes, J.L. 2024. Exposure to Cry1 toxins increases long flight tendency in susceptible but not in Cry1F-resistant female Spodoptera frugiperda (Lepidoptera: Noctuidae). Insects. 15: 7. https://doi.org/10.3390/insects15010007
Dively, G.P., Huang, F., Oyediran, I., Burd, T., and Morsello, S. 2019. Evaluation of gene flow in structured and seed blend refuge systems of non-Bt and Bt corn. J. Pest Sci. https://doi.org/10.1007/s10340-019-01126-4.
Dively, G.P., Kuhar, T.P., Taylor, S., Doughty, H.B., Holmstrom, K., Gilrein, D., Nault, B.A., Ingerson-Mahar, J., Whalen, J., Reisig, D. and Frank, D.L. 2021. Sweet corn sentinel monitoring for Lepidopteran field-evolved resistance to Bt toxins. J. Econ. Entomol. 114(1): 307-319.
Dively, G.P., Kuhar, T.P., Taylor, S.V., Doughty, H., Holmstrom, K., Gilrein, D.O., Nault, B.A., Ingerson-Mahar, J., Huseth, A., Reisig, D. Fleischer, S, Owens, D., Tilmon, K., Reay-Jones, F., Porter, P., Smith, J., Saguez, J., Wells, J., Congdon, C., Byker, H., Jensen, B, DiFonzo, C., Hutchison, W.D., Burkness, E., Wright, R., Crossley, M., Darby, H., Bilbo, T., Seiter, N., Krupke, C., Abel, C., Coates, B.S., McManus, B., Fuller, B., Bradshaw, J., Peterson, J.A., Buntin, D., Paula-Moraes, S., Kesheimer, K., Crow, W., Gore, J., Huang, F., Ludwick, D.C., Raudenbush, A., Jimenez, S., Carriere, Y., Elkner, T., and Hamby, K. 2023. Extended sentinel monitoring of Helicoverpa zea resistance to Cry and Vip3Aa toxins in Bt sweet corn: Assessing changes in phenotypic and allele frequencies of resistance. Insects. 14(7): 577.
Dively, G.P., Venugopal, P.D. and Finkenbinder, C. 2016. Field-evolved resistance in corn earworm to Cry proteins expressed by transgenic sweet corn. PLoS One. 11(12): p.e0169115.
Eyun, S.I., Wang, H., Pauchet, Y., Ffrench-Constant, R.H., Benson, A.K., Valencia-Jiménez, A., Moriyama, E.N., and Siegfried, B.D. 2014. Molecular evolution of glycoside hydrolase genes in the western corn rootworm (Diabrotica virgifera virgifera). PLoS One. 9(4): p.e94052.
Fabrick, J.A., Heu, C.C., LeRoy, D.M., DeGain, B.A., Yelich, A.J., Unnithan, G.C., Wu, Y., Li, X., Carrière, Y., and Tabashnik, B.E. 2022. Knockout of ABC transporter gene ABCA2 confers resistance to Bt toxin Cry2Ab in Helicoverpa zea. Sci. Rep. 12(1): 16706.
Farhan, Y., Smith, J.L., Sovic, M.G., and Michel, A.P. 2023. Genetic mutations linked to field-evolved Cry1Fa-resistance in the European corn borer, Ostrinia nubilalis. Sci. Rep. 13: 8081.
Flagel, L.E., Swarup, S., Chen, M., Bauer, C., Wanjugi, H., Carroll, M., Hill, P., Tuscan, M., Bansal, R., Flannagan, R., Clark, T., Michel, A.P., Head, G.P., and Goldman, B.S. 2015. Genetic markers for western corn rootworm resistance to Bt toxin. G3. 5: 399.
Frank, D.L., Kurtz, R., Tinsley, N.A., Gassmann, A.J., Meinke, L.J., Moellenbeck, D., Gray, M.E., Bledsoe, L.W., Krupke, C.H., Estes, R.E., Weber, P., and Hibbard, B.E. 2015. Effect of seed blends and soil-insecticide on western and northern corn rootworm emergence from mCry3A+eCry3.1Ab Bt maize. J. Econ. Entomol. 108: 1260-1270.
French, B. W., B. S. Coates, and T. Sappington. 2014. Inheritance of an extended diapause trait in the northern corn rootworm, Diabrotica barberi (Coleoptera: Chrysomelidae). J. Appl. Entomol. 138: 213-221.
Gassmann, A.J., Petzold-Maxwell, J.L., Clifton, E.H., Dunbar, M.W., Hoffmann, A.M., Ingber, D.A., and Keweshan, R.S. 2014. Field-evolved resistance by western corn rootworm to multiple Bacillus thuringiensis toxins in transgenic maize. Proc. Natl. Acad. Sci. USA 111: 5141-5146.
Gassmann, A.J., Petzold-Maxwell, J.L., Keweshan, R.S., and Dunbar, M.W. 2011. Field-evolved resistance to Bt maize by western corn rootworm. PLoS One. 6(7):e22629. https://doi.org/10.1371/journal.pone.0022629
Gassmann, A.J., Petzold-Maxwell, J.L., Keweshan, R.S., and Dunbar, M.W. 2012. Western corn rootworm and Bt maize: challenges of pest resistance in the field. GM Crops Food. 3(3):235-244.
Gassmann, A.J., Shrestha, R.B., Jakka, S.R., Dunbar, M.W., Clifton, E.H., Paolino, A.R., Ingber, D.A., French, B.W., Masloski, K.E., Dounda, J.W., and St. Clair, C.R. 2016. Evidence of resistance to Cry34/35Ab1 corn by western corn rootworm (Coleoptera: Chrysomelidae): root injury in the field and larval survival in plant-based bioassays. J. Econ. Entomol. 109: 1872-1880
Goldstein, J.A., Mason, C.E., and Pesek, J. 2010. Dispersal and movement behavior of neonate European corn borer (Lepidoptera: Crambidae) on non-bt and transgenic bt corn. J. Econ. Entomol. https://doi.org/10.1603/ec093 04
Gould, F. 1994. Potential and problems with high‐dose strategies for pesticidal engineered crops. Biocon. Sci. Technol. 4: 451-461.
Gould, F., Cohen, M.B., Bentur, J.S., Kennedy, G.G., and Van Duyn, J. 2006. Impact of small fitness costs on pest adaptation to crop varieties with multiple toxins: A heuristic model. J. Econ. Entomol. 99: 2091-2099.
Gray, M. E., T. W. Sappington, N. J. Miller, J. Moeser, and M. O. Bohn. 2009. Adaptation and invasiveness of western corn rootworm: intensifying research on a worsening pest. Ann. Rev. Entomol. 54: 303-321.
Hayden, J., and Brambila, J. 2015. Pest alert: Helicoverpa armigera (Lepidoptera: Noctuidae), the old world bollworm. Florida Department of Agriculture and Consumer Services. 2015 Jun 17.
Head, G.P., Carroll, M., Evans, S., Rule, D.M., Willse, A., Clark, T., Storer, N., Flannagan, R., Samuel, L., and Meinke, L.J. 2017. Evaluation of SmartStax and SmartStax PRO maize against western corn rootworm and northern corn rootworm: Efficacy and resistance management. Pest Manag. Sci. 73: 1883-1899.
Hibbard, B.E., Frank, D.L., Kurtz, R., Boudreau, E., Ellersieck, M.R., and Odhiambo, J.F. 2011. Mortality impact of Bt transgenic maize roots expressing eCry3.1Ab, mCry3A, and eCry3.1Ab plus mCry3A on western corn rootworm larvae in the field. J. Econ. Entomol. 104: 1584-1591.
Hitchon, A.J., Smith, J.L., French, B.W., and Schaafsma, A.W. 2015. Impact of the Bt corn proteins Cry34/35Ab1 and Cry3Bb1 alone or pyramided on western corn rootworm (Coleoptera: Chrysomelidae) beetle emergence in the field. J. Econ. Entomol. 108: 1986-1993.
Hoffmann, A.M., French, B.W., Hellmich, R.L., Lauter, N., and Gassmann, A.J. 2015. Fitness costs of resistance to Cry3Bb1 maize by western corn rootworm. J. Appl. Entomol. 139: 403-415.
Huang, F., Niu, Y., Silva, T., Brown, S., Towles, T., Kerns, D., Jurat-Fuentes, J.L., Head, G.P., Carroll, M., Walker, W., and Lin, S. 2023. An extended investigation of unexpected Helicoverpa zea (Boddie) survival and ear injury on a transgenic maize hybrid expressing Cry1A/Cry2A/Vip3A toxins. Toxins. 15(7): 474.
Huang, F., Qureshi, J.A., Meagher, Jr. R.L., Reisig, D.D., Head, G.P., Andow, D.A., Ni, X., Kerns, D., Buntin, G.D., Niu, Y., Yang, F., and Dangal, V. 2014. Cry1F resistance in fall armyworm Spodoptera frugiperda: single gene versus pyramided Bt maize. PLoS One. 9: e112958.
Huynh, M.P., Bernklau, E.J., Coudron, T.A., Shelby, K.S., Bjostad, L.B., and Hibbard, B.E. 2019a. Characterization of corn root factors to improve artificial diet for western corn rootworm (Coleoptera: Chrysomelidae) larvae. J. Insect Sci. 19(2): 20.
Huynh, M.P., Hibbard, B.E., Vella, M.E., Lapointe, S.L., Niedz, R.P., Shelby, K.S., and Coudron, T.A. 2019b. Development of an improved and accessible diet for western corn rootworm larvae using response surface modeling. Sci. Rep. 9: 16009.
Huynh, M.P., Hibbard, B.E., Lapointe, S.L., Niedz, R.P., French, B.W., Pereira, A.E., Finke, D.L., Shelby, K.S., and Coudron, T.A. 2019c. Multidimensional approach to formulating a specialized diet for northern corn rootworm larvae. Sci. Rep. 9: 3709.
Huynh, M.P., Meihls, L.N., Hibbard, B.E., Lapointe, S.L., Niedz, R.P., Ludwick, D.C., and Coudron, T.A. 2017. Diet improvement for western corn rootworm (Coleoptera: Chrysomelidae) larvae. PLoS One. 12(11): e0187997.
Ingber, D.A., and Gassmann, A.J. 2015. Inheritance and fitness costs of resistance to Cry3Bb1 corn by western corn rootworm (Coleoptera: Chrysomelidae). J. Econ. Entomol. 108: 2421-2432.
Jakka, S.R., Gong, L., Hasler, J., Banerjee, R., Sheets, J.J., Narva, K., Blanco, C.A., and Jurat-Fuentes, J.L. 2015. Field-evolved mode 1 resistance of the fall armyworm to transgenic Cry1Fa-expressing corn associated with reduced Cry1Fa toxin binding and midgut alkaline phosphatase expression. Appl. Environ. Microbiol. 82: 1023-1034.
Jakka, S.R., Knight, V.R., and Jurat-Fuentes, J.L. 2014. Fitness costs associated with field-evolved resistance to Bt maize in Spodoptera frugiperda (Lepidoptera: Noctuidae). J. Econ. Entomol. 107: 342-351.
Jakka, S.R., Shrestha, R.B., and Gassmann, A.J. 2016. Broad-spectrum resistance to Bacillus thuringiensis toxins by western corn rootworm (Diabrotica virgifera virgifera). Sci. Rep. 6: 27860.
Kang, J., Onstad, D.W., Hellmich, R.L., Moser, S.E., and Hutchison, W.D. 2012. Modeling the impact of cross-pollination and low toxin expression in corn kernels on adaptation of European corn borer (Lepidoptera: Crambidae) to transgenic insecticidal corn. Environ. Entomol. 41: 200-211.
Kaur, G., Guo, J., Brown, S., Head, G.P., Price, P.A., Paula-Moraes, S., Ni, X., Dimase, M., and Huang, F. 2019. Field-evolved resistance of Helicoverpa zea (Boddie) to transgenic maize expressing pyramided Cry1A.105/Cry2Ab2 proteins in northeast Louisiana, the United States. J. Invertebr. Pathol. 163: 11-20.
Kerns, D.D., Yang, F., Kerns, D.L., Stewart, S.D., and Jurat-Fuentes, J.L. 2023. Reduced toxin binding associated with resistance to Vip3Aa in the corn earworm (Helicoverpa zea). Appl. Environ. Microbiol. 89: e0164423.
Keweshan, R.S., Head, G.P., and Gassmann, A.J. 2015. Effects of pyramided Bt corn and blended refuges on western corn rootworm and northern corn rootworm (Coleoptera: Chrysomelidae). J. Econ. Entomol. 108: 720-729.
Knolhoff, L.M., Glas, J.J., Spencer, J.L., and Berenbaum, M.R. 2010. Oviposition behaviors in relation to rotation resistance in the western corn rootworm. Environ. Entomol. 39(6): 1922-1928. https://doi.org/10.1603/EN09250.
Kozak, G.M., Wadsworth, C.B., Kahne, S.C., Bogdanowicz, S.M., Harrison, R.G., Coates, B.S., and Dopman, E.B. 2017. A combination of sexual and ecological divergence contributes to rearrangement spread during initial stages of speciation. Mol. Ecol. 26:2331–2347.
Kozak, G.M., Wadsworth, C.B., Kahne, S.C., Bogdanowicz, S.M., Harrison, R.G., Coates, B.S. and Dopman, E.B. 2019. Genomic basis of circannual rhythm in the European corn borer moth. Curr Biol. 29(20): 3501-3509.
Lata, D., Coates, B.S., Walden, K.O., Robertson, H.M., and Miller, N.J. 2022. Genome size evolution in the beetle genus Diabrotica. G3. 12(4): jkac052. doi: 10.1093/g3journal/jkac052.
Lassance, J.M., Groot, A.T., Liénard, M.A., Antony, B., Borgwardt, C., Andersson, F., Hedenström, E., Heckel, D.G. and Löfstedt, C. 2010. Allelic variation in a fatty-acyl reductase gene causes divergence in moth sex pheromones. Nature. 466(7305):486-489.
Li, G., Reisig, D., Miao, J., Gould, F., Huang, F., and Feng, H. 2016. Frequency of Cry1F non-recessive resistance alleles in North Carolina field populations of Spodoptera frugiperda (Lepidoptera: Noctuidae). PLoS One. 11(4): e0154492.
Liu, S., Sappington, T.W., Coates, B.S., and Bonning, B.C. 2021. Nudivirus sequences identified from the southern and western corn rootworms (Coleoptera: Chrysomelidae). Viruses. 13(2): 269. https://doi.org/10.3390/v13020269.
Liu, S., Sappington, T.W., Coates, B.S., and Bonning, B.C. 2022. Sequences encoding a novel toursvirus identified from southern and northern corn rootworms (Coleoptera: Chrysomelidae). Viruses. 14(2): 397. https://doi.org/10.3390/v14020397.
Ludwick, D.C., Meihls, L.N., Ostlie, K.R., Potter, B.D., French, L., and Hibbard, B.E. 2017. Minnesota field population of western corn rootworm (Coleoptera: Chrysomelidae) shows incomplete resistance to Cry34Ab1/Cry35Ab1 and Cry3Bb1. J. Appl. Entomol. 141: 28-40.
Ludwick, D.C., Meihls, L.N., Huynh, M.P., Pereira, A.E., French, B.W., Coudron, T.A., and Hibbard, B.E. 2018. A new artificial diet for western corn rootworm larvae is compatible with and detects resistance to all current Bt toxins. Sci. Rep. 8: 5379.
Mallet, J., and Porter, P. 1992. Preventing insect adaptation to insect-resistant crops: are seed mixtures or refugia the best strategy? Proc. Biol. Sci. 250: 165-169.
Marçon, P.C.R.G., Siegfried, B.D., Spencer, T., and Hutchison, W.D. 2000. Development of diagnostic concentrations for monitoring Bacillus thuringiensis resistance in European corn borer (Lepidoptera: Crambidae). J. Econ. Entomol. 93: 925-930.
Marçon, P.C.R.G., Young, L.J., Steffey, K.L., and Siegfried, B.D. 1999. Baseline susceptibility of European corn borer (Lepidoptera: Crambidae) to Bacillus thuringiensis toxins. J. Econ. Entomol. 92: 279-285.
Meihls, L.N., Higdon, M.L., Ellersieck, M.R., and Hibbard, B.E. 2012. Greenhouse-selected resistance to Cry3Bb1-producing corn in three western corn rootworm populations. PLoS One. 7: e51055
Meihls, L.N., Huynh, M.P., Ludwick, D.C., Coudron, T.A., French, B.W., Shelby, K.S., Hitchon, A.J., Smith, J.L., Schaafsma, A.W., Pereira, A.E., and Hibbard, B.E. 2018. Comparison of six artificial diets for western corn rootworm bioassays and rearing. J. Econ. Entomol. 111(6): 2727-2733.
Meinke, L.J., Siegfried, B.D., Wright, R.J., and Chandler, L.D. 1998. Adult susceptibility of western corn rootworm (Coleoptera: Chrysomelidae) populations to selected insecticides. J. Econ. Entomol. 91: 594-600.
National Agricultural Statistics Service, (annual) June Agricultural Survey for 2000–24. https://www.ers.usda.gov/data-products/adoption-of-genetically-engineered-crops-in-the-united-states/recent-trends-in-ge-adoption/#:~:text=Although%20HT%20seeds%20are%20also,corn%2C%20cotton%2C%20and%20soybeans.
Niu, Y., Oyediran, I., Yu, W., Lin, S., Dimase, M., Brown, S., Reay-Jones, F.P., Cook, D., Reisig, D., Thrash, B., and Ni, X. 2021. Populations of Helicoverpa zea (Boddie) in the southeastern United States are commonly resistant to Cry1Ab, but still susceptible to Vip3Aa20 expressed in MIR 162 corn. Toxins. 13(1): 63.
North, H.L., Fu, Z., Metz, R., Stull, M.A., Johnson, C.D., Shirley, X., Crumley, K., Reisig, D., Kerns, D.L., Gilligan, T., Walsh, T., Jiggins, C.D., and Sword, G.A. 2023. Rapid evolution of pesticide resistance via adaptation and interspecific introgression in a major North American crop pest. bioRxiv. doi: https://doi.org/10.1101/2023.10.04.560869
Nufer, M.I., Coates, B.S., Abel, C.A., O’Neill, P., McCracken, M., Jain, D., Pierce, C.A., Glover, J., Towles, T., Reddy, G.V., and Perera, O.P. 2024. Anatomy of a pest control failure: Introgression of cytochrome P450 337B3 alleles from invasive old-world bollworm into native corn earworm. bioRxiv. doi: https://doi.org/10.1101/2024.03.22.584691
Olmstead, D. L., B. A. Nault, and A. M. Shelton. 2016. Biology, Ecology, and Evolving Management of Helicoverpa zea (Lepidoptera: Noctuidae) in Sweet Corn in the United States. J. Econ. Entomol. 109: 1667–1676.
Onstad, D. W., Crespo, A.L.B., Pan, Z., Crain, P.R., Thompson, S.D., Pilcher, C.D., and Sethi, A. 2018. Blended refuge and insect resistance management for insecticidal corn. Environ. Entomol. 47: 210-219.
Onstad, D.W., Mitchell, P.D., Hurley, T.M., Lundgren, J.G., Porter, R.P., Krupke, C.H., Spencer, J.L., DiFonzo, C.D., Baute, T.S., Hellmich, R.L., Buschman, L.L., Hutchison, W.D., and Tooker, J.F. 2011. Seeds of change: Corn seed mixtures for resistance management and IPM. J. Econ. Entomol. 104: 343-352.
Oswald, K.J., French, B.W., Nielson, C., and Bagley, M. 2012. Assessment of fitness costs in Cry3Bb1-resistant and susceptible western corn rootworm (Coleoptera: Chrysomelidae) laboratory colonies. J. Appl. Entomol. 136: 730-740.
Pannuti, L.E.R., Paula-Moraes, S.V., Hunt, T.E., Baldin, E.L.L., Dana, L, Malaquias, J.V. 2016. Plant-to-plant movement of Striacosta albicosta (Lepidoptera: Noctuidae) and Spodoptera frugiperda (Lepidoptera: Noctuidae) in maize (Zea mays ). J.of Econ. of Entomol. 109(3): 1125–1131. https://doi.org/10.1093/jee/tow042
Paula-Moraes, S., Hunt, T. E., Wright, R. J., Hein, G. L., and Blankenship, E. E. 2012. On-plant movement and feeding of western bean cutworm (Lepidoptera: Noctuidae) early instars on corn, Environ. Entomol. 41(6): 1494–1500. https://doi.org/10.1603/EN12149
Paula-Moraes, S., Hunt, T. E., Wright, R. J., Hein, G. L., and Blankenship, E. E. 2013. Western bean cutworm survival and the development of economic injury levels and economic thresholds in field corn. J. Econ. Entomol. 106: 1274-1285.
Paula-Moraes, S.V., Calixto, E.S., Santos, A.A., Reay-Jones, F.P.F., Reisig, D., Farhan, Y., Smith, J.L., and Hutchison, W.D. 2024. Continental-scale migration patterns and origin of Helicoverpa zea (Lepidoptera: Noctuidae) based on a biogeochemical marker. Environ. Entomol. 53(3): 487-497. https://doi.org/10.1093/ee/nvae034
Pereira, A.E., Souza, D.S., Zukoff, S.N., Meinke, L.J., and Siegfried, B.D. 2017. Cross-resistance and synergism assays provide evidence for multiple mechanisms of resistance to pyrethroids in western corn rootworm populations. PLoS One. 12(6): e0179311.
Pereira, E.J.G., Storer, N.P., and Siegfried, B.D. 2011. Fitness costs of Cry1F resistance in laboratory-selected European corn borer (Lepidoptera: Crambidae). J. Appl. Entomol. 135: 1-6.
Pereira, A.E., Wang, H., Zukoff, S.N., Meinke, L.J., French, B.W., and Siegfried, B.D. 2015. Evidence of field-evolved resistance to bifenthrin in western corn rootworm (Diabrotica virgifera virgifera LeConte) populations in western Nebraska and Kansas. PLoS One. 10(11): e0142299.
Petzold-Maxwell, J., Siegfried, B.D., Hellmich, R.L., Abel, C.A., Coates, B.S., Spencer, T.A., and Gassmann, A.J. 2014. Effect of maize lines on fitness costs of Cry1F resistance in the European corn borer. J. Econ. Entomol. 107: 764-772.
Pezzini, D.T., Reisig, D.D., Buntin, G.D., Del Pozo-Valdivia, A.I., Gould, F., Paula-Moraes, S.V., and Reay-Jones, F.P. 2023. Impact of seed blend and structured maize refuge on Helicoverpa zea (Lepidoptera: Noctuidae) potential phenological resistance development parameters in pupae and adults. Pest Manag. Sci. 79(10): 3493-3503.
Pezzini, D., Taylor, K.L., Reisig, D.D., and Fritz, M.L. 2024. Cross-pollination in seed blended refuge and selection for Vip3a resistance in a Lepidopteran pest as detected by genomic monitoring. PNAS. doi:10.1073/pnas.2319838121.
Pleau, M.J., Huesing, J.E., Head, G.P., and Feir, D.J. 2002. Development of an artificial diet for the western corn rootworm. Entomol. Exp. Appl. 105: 1-11.
Razze, J.M., and Mason, C.E. 2012. Dispersal behavior of neonate European corn borer (Lepidoptera: Crambidae) on Bt corn. J. Econ. Entomol. https ://doi.org/10.1603/ec112 88.
Razze, J.M., Mason, C.E., and Pizzolato, T.D. 2011. Feeding behavior of neonate Ostrinia nubilalis (Lepidoptera: Crambidae) on Cry1Ab Bt corn: Implications for resistance management. J. Econ. Entomol. 104: 806-813.
Reay-Jones, F. P. F. 2019. Pest status and management of corn earworm (Lepidoptera: Noctuidae) in field corn in the united states. J. Integrated. Pest Manage. 10, 19. doi: 10.1093/jipm/pmz017
Reay-Jones, F.P.F., Bilbo, T.R., and Reisig, D.D. 2020. Decline in sublethal effects of bt corn on corn earworm (Lepidoptera: Noctuidae) linked to increasing levels of resistance. J. Econ. Entomol. 113(5): 2241-2249. doi:2210.1093/jee/toaa2163.
Reay-Jones, F.P.F., Buntin, G. D., Reisig, D. D., Bridges, W. C., Jr. 2024. Longitudinal trials illustrate interactive effects between declining Bt efficacy against Helicoverpa zea and planting dates of corn. J. Econ. Entomol. In press.
Reinders, J.D., Hitt, B.D., Stroup, W.W., French, B.W., and Meinke, L.J. 2018. Spatial variation in western corn rootworm (Coleoptera: Chrysomelidae) susceptibility to Cry3 toxins in Nebraska. PLoS One. 13(11): e0208266.
Reisig, D.D., Huseth, A.S., Bacheler, J.S., Aghaee, M.A., Braswell, L., Burrack, H.J., Flanders, K., Greene, J.K., Herbert, D.A., Jacobson, A., and Paula-Moraes, S.V. 2018. Long-term empirical and observational evidence of practical Helicoverpa zea resistance to cotton with pyramided Bt toxins. J. Econ. Entomol. 111(4): 1824-1833.
Reisig, D., Kesheimer, K., Bateman, N., Studebaker, G., Meyer, R., Reay-Jones, F., Shields, E., Owens, D., Buntin, G.D., Seiter, N., Hodgson, E., Sisson, A., Zuckoff, A., Villanueva, R., Towles, T., Hamby, K., DiFonzo, C., Hutchison, B., Potter, B., Catchot, A., Cook, D., Bradshaw, J., Peterson, J., Beauzay, P., Knodel, J., Tilmon, K., Baute, T., Varenhorst, A., Brown, S., Kerns, D., Porter, P., Taylor, S., and Jenson, B. 2022. Corn invertebrate loss estimates from the United States and Ontario, Canada – 2021. Crop Protection Network publication CPN-2019-21. https://doi.org/10.31274/cpn-20220722-0.
Reisig, D., Kesheimer, K., Bateman, N., Studebaker, G., Meyer, R., Reay-Jones, F., Wise, K., Owens, D., Buntin, G.D., Smith, J., Seiter, N., Hodgson, E., Sisson, A., Zuckoff, A., Villanueva, R., Towles, T., Hamby, K., Cramer, M., DiFonzo, C., Hutchison, B., Potter, B., Crow, W., Bish, M., Bradshaw, J., Peterson, J., Beauzay, P., Knodel, J., Tilmon, K., Baute, T., Varenhorst, A., Brown, S., Kerns, D., Porter, P., Bick, E., and Conley, S. 2023. Corn invertebrate loss estimates from the United States and Ontario, Canada – 2022. Crop Protection Network publication CPN-2019-22. https://doi.org/10.31274/cpn-20230421-0.
Reisig, D., Graham, S., Bateman, N., Studebaker, G., Meyer, R., Reay-Jones, F., Wise, K., Owens, D., Buntin, G.D., Smith, J., Seiter, N., Sisson, A., Zuckoff, A., Villanueva, R., Hamby, K., Cramer, M., DiFonzo, C., Yang, F., Hutchison, B., Crow, W., Towles, T., Floyd, C., Bradshaw, J., Peterson, J., Beauzay, P., Knodel, J., Krupke, C., Tilmon, K., Baute, T., Varenhorst, A., Brown, S., Kerns, D., Porter, P., Malone, S., and Bick, E. 2024. Corn invertebrate loss estimates from the United States and Ontario, Canada – 2023. Crop Protection Network publication CPN-2019-23. https://doi.org/10.31274/cpn-20240219-1.
Sappington, T.W. 2024. Critical facets of European corn borer adult movement ecology relevant to mitigating field resistance to Bt-corn. Insects. 15.
Schlum, K., Lamour, K., Tandy, P., Emrich, S.J., de Bortoli, C.P., Rao, T., Viteri Dillon, D.M., Linares-Ramirez, A.M., and Jurat-Fuentes, J.L. 2021. Genetic screening to identify candidate resistance alleles to Cry1F corn in fall armyworm using targeted sequencing. Insects. 12: 618.
Schrader, P.M., Estes, R.E., Tinsley, N.A., Gassmann, A.J., and Gray, M.E. 2017. Evaluation of adult emergence and larval root injury for Cry3Bb1-resistant populations of the western corn rootworm. J. Appl. Entomol. 141: 41-52.
Siegfried, B.D., and Hellmich, R.L. 2012. Understanding successful resistance management: The European corn borer and Bt corn in the United States. Special Issue of GM Crops and Foods 3: 184-193.
Siegfried, B.D., Rangasamy, M., Wang, H., Spencer, T., Haridas, C.V., Tenhumberg, B., Sumerford, D.V., and Storer, N.P. 2014. Estimating the frequency of Cry1F resistance in field populations of the European corn borer (Lepidoptera: Crambidae). Pest Manag. Sci. 70: 725-733.
Siegfried, B.D., and Spencer, T. 2012. Bt resistance monitoring in European corn borers and western corn rootworms. In: M. Oliver and Y. Li, eds. Plant Gene Containment. Elsevier, NY.
Siegfried, B.D., Spencer, T., Crespo, A.L., Storer, N.P., Head, G.P., Owens, E.D., and Guyer, D. 2007. Ten years of monitoring for Bt resistance in the European corn borer: What we know, what we don't know and what we can do better. Amer. Entomol. 53: 208-214.
Siegfried, B.D., Waterfield, N., and Ffrench-Constant, R.H. 2005. Expressed sequence tags from Diabrotica virgifera virgifera midgut identify a coleopteran cadherin and a diversity of cathepsins. Insect Mol. Biol. 14: 137-143.
Silva, T., Sword, G.A., Miller, A., Qureshi, J.A., Head, G.P., Kerns, D.D., Jurat-Fuentes, J.L., Villegas, J., Towles, T.B., Ni, X., Reay-Jones, F.P.F., Carrillo, D., Cook, D.R., Daves, C., Stout, M.J., Thrash, B., Paula-Moraes, S.V., Lin, S., Patla, B., Niu, Y., Sakuno, C.I.R., and Huang, F. 2024. Reversal of practical resistance in fall armyworm to Cry1F maize: a case report on the resistance to susceptibility in Bt crops from the southeastern USA. J. Pest Sci. https://doi.org/10.1007/s10340-024-01804-y.
Smith, E. 2014. Detection of old world bollworm (Helicoverpa armigera) in Puerto Rico. North American Plant Protection Organization. Phytosanitary Alert System Bulletin. https://www.pestalerts.org/nappo/official-pest-reports/617/
Smith, J.L., and Farhan, Y. 2023. Monitoring resistance of Ostrinia nubilalis (Lepidoptera: Crambidae) in Canada to Cry toxins produced by Bt corn. J. Econ. Entomol. 116(3): 916-926.
Smith, J.L., Farhan, Y., and Schaafsma, A.W. 2019. Practical resistance of Ostrinia nubilalis (Lepidoptera: Crambidae) to Cry1F Bacillus thuringiensis maize discovered in Nova Scotia, Canada. Sci. Rep. 9: 18247.
Smith, J.L., Rule, D.M., Lepping, M.D., Farhan, Y., and Schaafsma, A.W. 2017. Evidence for field-evolved resistance of Striacosta albicosta (Lepidoptera: Noctuidae) to Cry1F Bacillus thuringiensis protein and transgenic corn hybrids in Ontario, Canada. J. Econ. Entomol. 110: 2217-2228.
Souza, D. 2019. Characterization of pyrethroid resistance in the western corn rootworm Diabrotica virgifera virgifera LeConte. Ph.D dissertation, University of Nebraska-Lincoln.
Souza, D., Jiménez, A.V., Sarath, G., Meinke, L.J., Miller, N.J., and Siegfried, B.D. 2020. Enhanced metabolism and selection of pyrethroid-resistant western corn rootworms (Diabrotica virgifera virgifera LeConte). Pest Biochem. Physiol. 164: 165-172.
Souza, D., Peterson, J.A., Wright, R.J., and Meinke, L.J. 2019b. Field efficacy of soil insecticides on pyrethroid-resistant western corn rootworms (Diabrotica virgifera virgifera LeConte). Pest Manag. Sci. doi:10.1002/ps.5586
Souza, D., Vieira, B.C., Fritz, B.K., Hoffmann, W.C., Peterson, J.A., Kruger, G.R., and Meinke, L.J. 2019a. Western corn rootworm pyrethroid resistance confirmed by aerial application simulations of commercial insecticides. Sci. Rep. 9: 6713.
Stahlke, A.R., Chen, J., Tembrock, L.R., Sim, S.B., Chudalayandi, S., Geib, S.M., Scheffler, B.E., Perera, O.P., Gilligan, T.M., Childers, A.K., Hackett, K.J., and Coates, B.S. 2022. A chromosome-scale genome assembly of a Helicoverpa zea strain resistant to Bacillus thuringiensis Cry1Ac insecticidal protein. Genome Biol. Evol. 15(3).
Storer, N.P., Babcock, J.M., Schlenz, M., Meade, T., Thompson, G.D., Bing, J.W., and Huckaba, R.M. 2010. Discovery and characterization of field resistance to Bt maize: Spodoptera frugiperda (Lepidoptera: Noctuidae) in Puerto Rico. J. Econ. Entomol. 103: 1031-38.
Tandy, P., Lamour, K., Placidi de Bortoli, C., Nagoshi, R., Emrich, S.J., and Jurat-Fuentes, J.L. 2023. Screening for resistance alleles to Cry1 proteins through targeted sequencing in the native and invasive range of Spodoptera frugiperda (Lepidoptera: Noctuidae). J. Econ. Entomol. 116: 935-944
Taylor, K.L., Hamby, K.A., DeYonke, A.M., Gould, F., and Fritz, M.L. 2021. Genome evolution in an agricultural pest following adoption of transgenic crops. Proc. Natl. Acad. Sci. USA. 118(52): e2020853118.
Taylor, K.L., Quackenbush, J., Lamberty, C., Hamby, K.A., and Fritz, M.L. 2024. Polygenic basis of field evolved Bt Cry resistance in the corn earworm, Helicoverpa zea. bioRxiv. 2024-02.
Tessnow, A.E., Nagoshi, R.N., Meagher, R.L., and Fleischer, S.J. 2023. Revisiting fall armyworm population movement in the United States and Canada. Front. Insect Sci. 3. https://doi.org/10.3389/finsc.2023.1104793
Tinsley, N. A., Spencer, J. L., Estes, R. E., Estes, K. A., Kaluf, A. L., Isard, S. A., Levine, E., & Gray, M. E. (2018). Multi-year surveys reveal significant decline in western corn rootworm densities in Illinois soybean fields. Am. Entomol., 64(2), 112–119.
Unbehend, M., Kozak, G.M., Koutroumpa, F., Coates, B.S., Dekker, T., Groot, A.T., Heckel, D.G., Dopman, E.B. 2021. Bric à brac controls sex pheromone choice by male European corn borer moths. Nat. Commun. 12: 2818. https://doi.org/10.1038/s41467-021-23026-x
Valencia-Montoya, W.A., Elfekih, S., North, H.L., Meier, J.I., Warren, I.A., Tay, W.T., Gordon, K.H.J., Specht, A., Paula-Moraes, S.V., Rane, R., Walsh, T.K., and Jiggins, C.D. 2020. Adaptive introgression across semipermeable species boundaries between local Helicoverpa zea and invasive Helicoverpa armigera moths. Mol. Biol. Evol. 37: 2568-2583.
Velez, A.M., Spencer, T.A., Alves, A.P., Crespo, A.L.B., and Siegfried, B.D. 2013a. Fitness costs of Cry1F resistance in fall armyworm, Spodoptera frugiperda. J. Appl. Entomol. 138: 315-325.
Velez, A.M., Spencer, T.A., Alves, A.P., Moellenbeck, D., Meagher, R.L., Chirakkal, H., and Siegfried, B.D. 2013b. Inheritance of Cry1F resistance, cross-resistance and frequency of resistant alleles in Spodoptera frugiperda (Lepidoptera: Noctuidae). Bull. Entomol. Res. 103: 700-7013.
Vellichirammal, N.N., Wang, H. Eyun, S., Moriyama, E.N. Coates, B.S., Miller, N.J., and Siegfried, B.D. 2015. Transcriptional analysis of susceptible and resistant European corn borer strains and their response to Cry1F protoxin. BMC Genomics. 16: 558.
Walsh, T.K., Joußen, N., Tian, K., McGaughran, A., Anderson, C.J., Qiu, X., Ahn, S.-J., Bird, L., Pavlidi, N., Vontas, J., Ryu, J., Rasool, A., Macedy, I.B., Tay, W.T., Zhang, Y., Whitehouse, M.E.A., Silvie, P.J., Downes, S., Nemec, L., and Heckel, D.G. 2018. Multiple recombination events between two cytochrome P450 loci contribute to global pyrethroid resistance in Helicoverpa armigera. PLoS One. 13: e0197760.
Wang, H., Eyun, S.I., Arora, K., Tan, S.Y., Gandra, P., Moriyama, E., Khajuria, C., Jurzenski, J., Li, H., Donahue, M., and Narva, K. 2017. Patterns of gene expression in western corn rootworm (Diabrotica virgifera virgifera) neonates, challenged with Cry34Ab1, Cry35Ab1 and Cry34/35Ab1, based on next-generation sequencing. Toxins. 9(4): 124.
Wangila, D.S., Gassmann, A.J., Petzold-Maxwell, J.L., French, B.W., and Meinke, L.J. 2015. Susceptibility of Nebraska western corn rootworm (Coleoptera: Chrysomelidae) populations to Bt corn events. J. Econ. Entomol. 108: 742-751.
Wangila, D.S., and Meinke, L.J. 2017. Effects of adult emergence timing on susceptibility and fitness of Cry3Bb1-resistant western corn rootworms. J. Appl. Entomol. 141: 372-383.
Wechsler, S., & Smith, D. (2018). Has resistance taken root in U.S. corn fields? Demand for insect control. Am. J. Agric. Econ., 100(4), 1136–1150.
Westbrook, J., Fleischer, S., Jairam, S., Meagher, R., and Nagoshi, R. 2019. Multigenerational migration of fall armyworm, a pest insect. Ecosphere. 10: e02919.
Yang, F., González, J.C.S., Head, G.P., Price, P.A., and Kerns, D.L. 2021a. Multiple and non-recessive resistance to Bt proteins in a Cry2Ab2-resistant population of Helicoverpa zea. Crop Prot. 145: 105650.
Yang, F., González, J.C.S., Little, N., Reisig, D., Payne, G., Dos Santos, R.F., Jurat-Fuentes, J.L., Kurtz, R. and Kerns, D.L. 2020b. First documentation of major Vip3Aa resistance alleles in field populations of Helicoverpa zea (Boddie)(Lepidoptera: Noctuidae) in Texas, USA. Sci. Rep. 10(1): 5867.
Yang, F., Head, G.P., Price, P.A., Santiago González, J.C., and Kerns, D.L. 2020a. Inheritance of Bacillus thuringiensis Cry2Ab2 protein resistance in Helicoverpa zea (Lepidoptera: Noctuidae). Pest Manag. Sci. 76(11): 3676-3684.
Yang, F., Kerns, D.L., Brown, S., Head, G.P., and Huang, F. 2017. Pollen contamination in seed mixture increases the dominance of resistance to Bt maize in Spodoptera frugiperda (Lepidoptera: Noctuidae). Pest Manag. Sci. 73: 2379-2385.
Yang, F., Kerns, D.L., Head, G.P., Leonard, B.R., Levy, R., Niu, Y., and Huang, F. 2014. A challenge for the seed mixture refuge strategy in Bt maize: impact of cross-pollination on an ear-feeding pest, corn earworm. PLoS One. 9(11): e112962.
Yang, F., Santiago González, J.C., Sword, G.A., and Kerns, D.L. 2021b. Genetic basis of resistance to the Vip3Aa Bt protein in Helicoverpa zea. Pest Manag. Sci. 77(3): 1530-1535.
Yang, F., Williams, J., Porter, P., Huang, F., and Kerns, D.L. 2019. F2 screen for resistance to Bacillus thuringiensis Vip3Aa51 protein in field populations of Spodoptera frugiperda (Lepidoptera: Noctuidae) from Texas, USA. Crop Prot. 126: 104915.
Yu, W., Head, G.P., and Huang, F. 2022. Inheritance of resistance to Cry1A.105 in Helicoverpa zea (Boddie) (Lepidoptera: Noctuidae). Insects. 13(10): 875.
Zhao, J.Z., Cao, J., Li, Y.X., Collins, H.L., Roush, R.T., Earle, E.D., and Shelton, A.M. 2003. Transgenic plants expressing two Bacillus thuringiensis toxins delay insect resistance evolution. Nature Biotechnology. 21: 1493-1497. DOI: 10.1038/nbt907.
Zhao, Z., Elsik, C.G., Hibbard, B.E., and Shelby, K.S. 2021. Detection of alternative splicing in western corn rootworm (Diabrotica virgifera virgifera LeConte) in association with eCry3.1Ab resistance using RNA‐seq and PacBio Iso‐Seq. Insect Mol. Biol. 30(4): 436-445.
Zhao, Z., Meihls, L.N., Hibbard, B.E., Ji, T., Elsik, C.G., and Shelby, K.S. 2019. Differential gene expression in response to eCry3. 1Ab ingestion in an unselected and eCry3. 1Ab-selected western corn rootworm (Diabrotica virgifera virgifera LeConte) population. Sci. Rep. 9(1): 4896.
Zhao, J.Z., O'Neal, M.A., Richtman, N.M., Thompson, S.D., Cowart, M.C., Nelson, M.E., Pan, Z.Q., Alves, A.P., and Yamamoto, T. 2016. mCry3A- selected western corn rootworm (Coleoptera: Chrysomelidae) colony exhibits high resistance and has reduced binding of mCry3A to midgut tissue. J. Econ. Entomol. 109: 1369-1377.
Zukoff, S.N., Ostlie, K.R., Potter, B., Meihls, L.N., Zukoff, A.L., French, L., Ellersieck, M.R., French, B.W., and Hibbard, B.E. 2016. Multiple assays indicate varying levels of cross resistance in Cry3Bb1-selected field populations of the western corn rootworm to mCry3A, eCry3.1Ab, and Cry34/35Ab1. J. Econ. Entomol. 109(3): 1387–1398. https://doi.org/10.1093/jee/tow073.