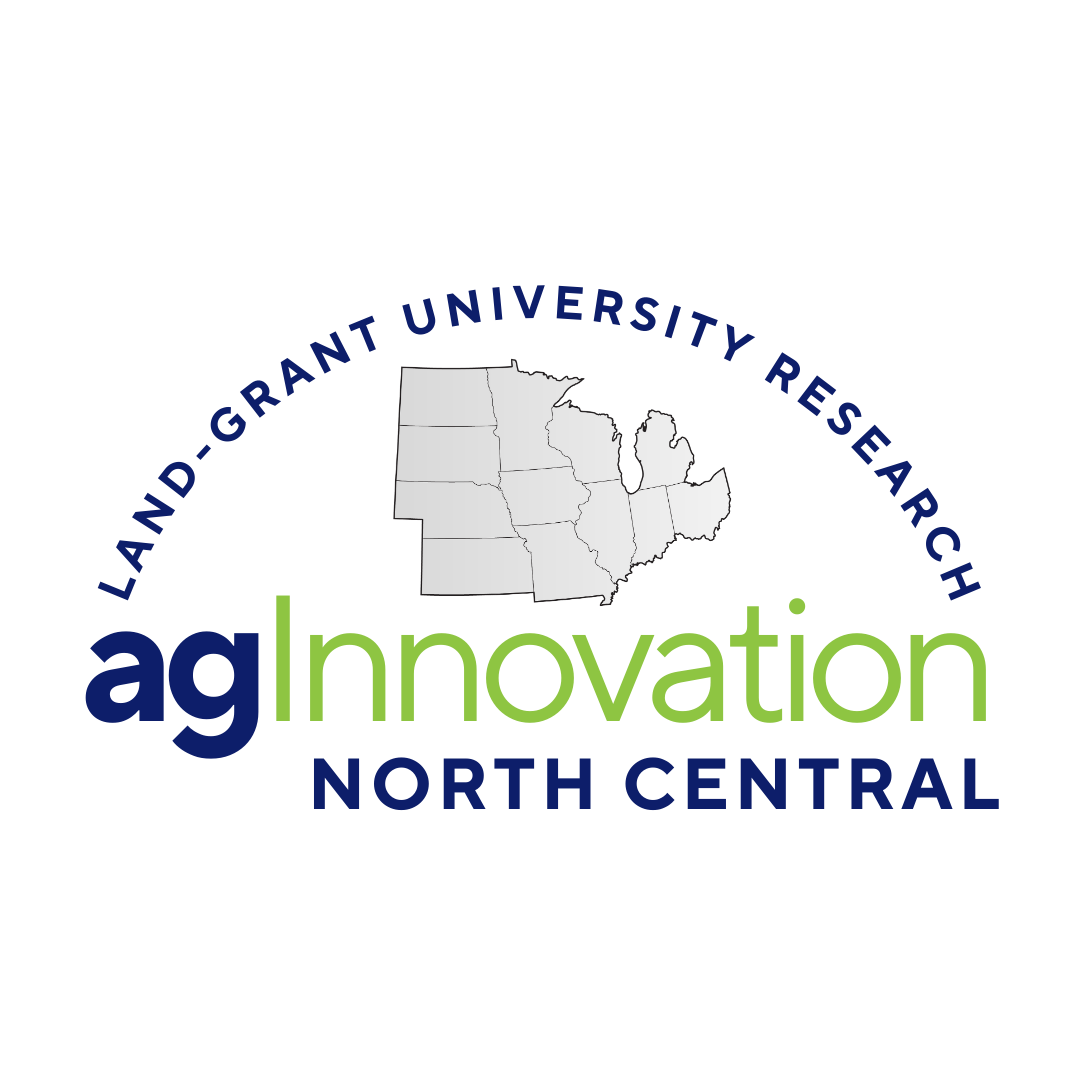
NC_temp1186: Water Management and Quality for Specialty Crop Production and Health
(Multistate Research Project)
Status: Under Review
NC_temp1186: Water Management and Quality for Specialty Crop Production and Health
Duration: 10/01/2025 to 09/30/2030
Administrative Advisor(s):
NIFA Reps:
Non-Technical Summary
The production of specialty crops is associated with intensive use of water, fertilizers and agrichemicals. These crops and related green industry activities include container and soilless-grown ornamental and edible plants in nurseries, greenhouses, controlled-environments (hydroponics, vertical farming, plant factories), and an urban component of outdoor urban gardening/farming. The need for significant volumes of high-quality water for these commodities has become a major challenge due to climate change (recurrent and severe droughts) and increasing competition for fresh, good-quality water resources with other priority urban and suburban needs. The overarching goal of this multistate project is to addresses pressing water issues affecting the sustainability and profitability of specialty crops, in particular addressing quality of available (traditional and alternative) irrigation water sources, improving irrigation use efficiency, properly managing drainage and runoff effluents and improved use of substrates and soilless culture. The primary audiences to benefit from the results and educational programs resulting from the proposed research activities of this multistate project include commercial growers, horticulture consultants and educators, horticulture suppliers and even homeowners growing specialty crops on their backyard gardens and residential landscapes. The expected outcomes from this project include significant improvements in the efficient use and conservation of fresh, and good-quality water resources, reductions in both the use of fertilizer and agrichemicals and their potentially contaminant effluents (environmental benefits), knowledge on the proper use and management of alternative water sources, new and improved soilless substrates; all together leading to sustained, or increased, specialty crop productivity and quality (crop growth benefits).
Statement of Issues and Justification
Accelerated population growth and climate change have positioned the availability and quality of freshwater resources as most crucial for society at the local, regional and national scales. In United States, irrigated agriculture and urban/public supply account for 37% and 12%, respectively of the total daily water use (Dieter et al., 2015). Specialty crop producers and the green industry, which includes ornamental plant producers, urban landscape & green infrastructure managers, and urban farmers, have become significantly affected by accessibility to high quality water resources and the concomitant issues of water use efficiency, irrigation management, salinity and drought, among others.
The production systems in this specialty crop sector, with an annual farmgate value of $11.8 billion dollars (NASS, 2020), traditionally have included ornamental growers, but it has diversified to include edible crops in soilless culture, including controlled-environment greenhouses and buildings (plant factories, vertical farming). The urban component of the green industry also extends to include outdoor urban gardening/farming. Altogether, these commodities are known for their intensive management and use of good quality water sources (Cabrera, 2021; Fulcher et al., 2016). Thus, their profitability and sustainability are jeopardized when challenged with water availability and quality issues due to pervasive issues of seasonal and extended droughts (McCabe et al., 2022), reduced allocations (Milman and Canon, 2023), restrictions/prohibitions on urban potable water use (Nemati et al., 2023), and regional salinity issues (i.e., saltwater intrusion, deicing salts) (Burga, 2023; Kaushal et al., 2023). Furthermore, the intensive use of fertilizers and agrichemicals in these commodities (Abdi et al., 2021; Cabrera, 2021) coupled with poor water use efficiencies lead to high pollution potential from their tailwater effluents into local water bodies (surface and ground), triggering local and regional scrutiny, pervasive monitoring and challenging environmental regulations and enforcement (California Water Boards, 2024; Majsztrik and Lea-Cox, 2013).
Under these scenarios, a range of water management and conservation practices need to be implemented by specialty crops and green industries, including increases in applied water use efficiency and reduced water footprints; use of alternative water sources of lesser quality; capture, treatment and recycling of drainage and on-farm stormwater effluents; development and use of improved/engineered soilless substrates; and management of field soils. Water quality aspects relevant to use of alternative and recycled water sources for irrigation include chemical (alkalinity, salinity, emergent environmental contaminants, nitrogen, phosphorus, pesticide residues, pharmacological residues, etc.), biological (plant pathogens, algae, biofilm, human food safety pathogens, coliform bacteria, etc.), and physical components (suspended particulates, turbidity, micro- and nanoplastics, etc.).
These challenges support the continuation of a multistate, multidisciplinary research and extension group that addresses the broad range of water quantity, quality and plant production issues affecting the profitability and sustainability of specialty crops. To address these challenges and produce tangible and practical solutions to these issues through research and extension activities, this multistate project has identified four principal areas of concern: (1) quality of irrigation sources, (2) irrigation management and efficiency, (3) runoff management, and (4) substrates and soilless culture.
1. Quality of irrigation water sources
The aesthetic requirements of ornamental specialty crops place constraints on the chemical quality of the water used for their irrigation, particularly when this is largely accomplished with overhead sprinkler systems. Furthermore, a broad majority of ornamental species, woody plants and edible specialty crops in particular, are fairly sensitive to moderate concentrations of total soluble salts and specific ions like sodium (Na), chloride (Cl) and boron (B) (Duncan et al., 2009; Grieve et al., 2012; Niu and Cabrera, 2010). Thus, traditional water sources can have local/regional quality issues that require treatment and management before their use for irrigation.
The traditional water sources used in specialty crops include surface and groundwater, but their increasing regional scarcity due to severe droughts and competition by other priority uses are giving way to alternative sources like municipal reclaimed water and recycled agricultural tailwater from runoff and drainages (Cabrera et al., 2018; Duncan et al., 2009). Although the quality of groundwater sources are often impaired by natural geological features, their chemical footprint is often stable over time. Conversely, surface waters are fairly vulnerable to contamination and significant changes in their chemical, physical, and biological quality because they do not have a protective over-layer of soil. Non-traditional irrigation sources like municipal reclaimed water often are contaminated by salts, pollutants from industrial, commercial and residential activities (Bale et al., 2017; Tanji et al., 2007) and emerging environmental contaminants (e.g., microplastics, PFAS, pharmaceutical residues; Donley et al., 2024; Fork et al., 2021; Thornton-Hampton et al., 2022). Agricultural tailwaters (drainages) can also fluctuate significantly in their quality, often containing significant residues of fertilizer salts and organic pesticides (Abdi et al., 2021; Alexandrino et al., 2022; Majsztrik et al., 2017).
Specialty crop producers must therefore know which tools and techniques to use to systematically monitor key water quality parameters, employ technologies to remove harmful contaminants, adopt/modify irrigation management practices and production systems, and/or select crops that are tolerant of lower quality water sources. There may also be opportunity to use specialty crop production to mitigate emerging environmental contaminants from waters, whether recycled or used only once, and help improve water quality and protect communities and water users around them.
2. Irrigation management and use efficiency
Efficient use of water is key to reduce waste of this resource. Sound irrigation management practices are required to reduce both water inputs (volumes required to grow a crop) and outputs (drainage and runoff). While container nursery and greenhouse growers vary in their water application practices (Majsztrik et al., 2018a), water application rates from these commodities are 4 to 5-fold higher than irrigated agronomic crops (for example 84” vs 18” per year; Cabrera et al., 2013). Under the assumption of similar overall water use efficiencies in both agronomic and specialty cropping systems, the latter generate significantly higher drainage output volumes per unit area. An intensive and frequent irrigation program is essential for crop production in soilless substrates, as these have high levels of porosity and low water holding capacity, and they have significantly smaller rootzone volumes compared to field-grown plants (Caron and Michel, 2021). Most nursery and floriculture crops in the US are grown in containers (NASS, 2020), and thus require daily (often several times a day) irrigation applications. Increases in irrigation use efficiency are necessary in containerized production systems because soilless substrates provide little buffering capacity to reduce leaching of nutrients and pesticides.
While overhead sprinklers are the traditional method to irrigate container nursery crops (Spinelli et al., 2024), other more efficient micro-irrigation options are available, including micro-sprinklers, low-volume spray stakes and drip irrigation, and recirculating sub-irrigation systems in greenhouse crops. In general, the more efficient irrigation systems are more expensive to install and maintain. There is limited information available on differences in water use among these different irrigation approaches for container crop production, on the economics of their use, and their compatibility with the quality of alternative water sources.
In addition to advances in equipment, improvements in irrigation management and efficiency require knowledge on how, when, and how much water to apply (Basiri-Jahromi, et al., 2020), namely control of timing, volume, and delivery of water based on measurement of soil/substrate moisture status, potential crop water use through evapotranspiration modeling (climate-based or from a proxy reference crop), and/or measurement of crop water stress. However, successful use and adoption of these methods requires science-based knowledge, cost-effective tools and the training of practitioners.
3. Runoff management
Specialty crop producers across the US have begun to capture, treat and recycle tailwater and stormwater runoff from their facilities. Procedures can significantly increase water use efficiency (WUE), potentially reduce production costs, and mitigate pollution associated with fertilizer runoff when water is recycled. However, recycled runoff effluents often contains agrichemical residues with phytotoxic effects (Abdi et al., 2021; Baz and Fernandez, 2002; Warsaw et al., 2012). Phytotoxicity problems are often due to residues of agrichemicals with high water solubility, or when persistent agrichemicals are extensively used, and the recycled water is applied to crops sensitive to that compound. Phytotoxic effects of preemergent herbicide residues of 1-10 parts per million (ppm) and the growth retardant paclobutrazol at 5 parts per billion (ppb) have been reported in several ornamental crops (Baz and Fernandez, 2002; Raudales et al., 2024).
The costs of infrastructure to collect, capture and treat irrigation water runoff discourages adoption of recycling practices (Gottlieb et al., 2022; Pitton et al., 2018). Managing runoff is a major challenge for container crop growers, as the volumes of water applied and the generated runoff are significantly higher than from field production; thus, when compounded with increased agrichemical loads can have a major impact on nearby receiving bodies of water (Lea-Cox and Ross, 2018). Thus, assessment and management of runoff play a critical role in minimizing the environmental impacts of specialty crop operations (White, 2013). Runoff can be substantially reduced by effective irrigation management (Pershey et al., 2015), and its capture, treatment and recycling help increase WUE and reduce pollution (Majsztrik et al., 2017). Large greenhouse operations have addressed runoff by using closed (sub)irrigation systems, but these are cost-prohibitive and impractical for smaller operations and outdoor container nurseries. Several promising treatment technologies (activated carbon, bioreactors, floating treatment wetlands, cold plasma-activated water) have been developed at the laboratory scale and are in various stages of investigation at the production scale.
Recycled irrigation water can contain and spread pathogens from a single infected plant to an entire operation, resulting in severe crop losses (Nyberg et al. 2014). Dozens of species of fungi, oomycetes, bacteria, viruses, and nematodes have been identified in recycled water sources (Redekar et al., 2019). Thus, there is a need to assess the risk posed by pathogens in recycled waters and evaluate mitigation strategies. While there are several water treatment technologies for waterborne pathogens, their eventual adoption involves several economic and crop management factors, along with extension/outreach education to growers (Raudales et al., 2014).
4. Substrates and soilless culture
Continued reductions of water, fertilizer and pesticide inputs, and runoff from specialty crop production can be accomplished with the proper selection, design and management of container substrates and soilless culture methods, which have the potential for higher yields than soil-based production. However, because of their small rootzone volumes, soilless systems provide a limited and dynamic reservoir capacity of water, mineral nutrients and oxygen (Caron and Michel, 2021), thus requiring systematic monitoring and management to minimize plant stress and ensure maximum crop growth potential.
Recent research demonstrates that substrate components can be stratified (layered) within a container to improve irrigation and fertilizer efficiency while producing plants with similar yield and quality to those grown in conventional systems (Fields et al., 2021; Owen et al., 2022). Further exploration into substrate stratification has revealed additional benefits for growers including reducing weed growth (Yvraj et al., 2022) and reducing reliance on peat moss, which can also lower substrate-related costs (Fields and Criscione, 2023). The engineering of new substrates and soilless systems require further refinement to improve supply and delivery of nutrients to increase nutrient use efficiency and reduce leaching and volatilization losses.
The traditional approaches to substrate evaluation and development have focused on static physical (water holding and air-filled porosity) and chemical properties (pH, electrical conductivity, selected ions). However, limited attention has been applied to the dynamic changes in these physical and chemical properties that occur over the relatively short time intervals that substrate systems remain in flux (s, min. hr.), or with continuous root growth over the crop production cycle (Judd et al., 2015). These dynamic root environments affect plant growth and development, crop timing, profitability, and resource efficiency (Caron and Michel, 2021). Additionally, little is known about the biological properties of soilless substrates, as historically, they were considered of minor importance (Giuffrida et al., 2021); though recent research has highlighted the complex dynamics and relationships of microbial communities of various substrates and how they are affected by the crop plants and fertilization inputs (Quijia-Pillajo et al., 2024; Valles-Ramirez et al., 2023).
Proposed participating stations and other project members: Land Grant States/Institutions: AR, CA, FL, GA, LA, MA, MD, MI, MO, NC, NJ, OH, OR, SC, TN, UT, VA. Non-Land Grant Participating States/Institutions: USDA-ARS Application Technology Unit, Wooster, OH; USDA-ARS National Arboretum, McMinnville, TN
Related, Current and Previous Work
The membership of this multistate team has strong relationships with colleagues working on related areas, and we intend to continue these active collaborations. For instance, members of NC1186 lead two recently (2024) funded USDA-SCRI grant projects: Labor, Efficiency, Automation, and Production: LEAP Nursery Crops Toward Sustainability (2024-51181-43291) and Beyond Peat: Substrate Security for a Changing Climate (2024-51181-43290).
A search of multistate projects in NIMSS and NIFA Data Gateway was conducted to identify overlaps and potential collaborations with other projects and members. The multistate NE2335 group (Resource Optimization in Controlled Environment Agriculture, 2023-2028) offers potential collaboration as one of their objectives focuses on root-zone management, including management of substrates, water and nutrients in soilless greenhouse production systems. Our team also collaborates with members of S1051 (Sustainable Practices, Economic Contributions, Consumer Behavior, and Labor Management in the U.S. Environmental Horticulture Industry) on water audits and life-cycle analyses. Some members of our team are also collaborating in the multistate coordinating committee SERA27 (Nursery Crop and Landscape Systems), which includes water (drought, flooding) as one of the abiotic stressors in ornamental plant production and landscape management. There are multistate projects in other commodities with components related to irrigation water and quality, and the NC1186 team will continue to actively identify opportunities for joint meetings and collaboration.
1. Water quality of irrigation sources
Laundry graywaters of different composition were used to irrigate a dozen containerized ornamental species (Cabrera and Altland, 2024), and plant growth and quality in most species were comparable to those irrigated with tap water. However, bleach in graywater caused negative effects on growth/quality of some species. It is hypothesized that effluents from new generation biodegradable, plant-based detergents will significantly reduce the phytotoxicity potential of graywaters used to irrigate landscape plants. Information on their short and long-term effects on effects on soil microbiology is also needed.
Laboratory studies are being conducted to evaluate the effect of wetting agents and application rates on water retention and solute movement/leaching in sands (turf greens) and urban soils (Jiang et al., 2022), and their implications on the safe use of municipal reclaimed waters as irrigation sources.
Ozone and cold plasma technologies are used to disinfect recycled nutrient solutions in nursery/greenhouse crops, especially for treatment of surface irrigation water sources and recirculated nutrient solution. Research is primarily focused on the effective dose response for control of pathogens, algae and biofilm, however there can be negative secondary effects such as decreased the solubility of Fe and Mn in hydroponic solutions (Thakulla and Fisher, 2023). Design and choice of water treatment systems requires consideration not only of sanitation, but also compatibility with other aspects of nursery operations such as fertilizers, worker safety, and beneficial microbes.
The runoff water quality from a commercial container nursery was assessed with a stormwater management model (SWMM). Storm events generated higher concentrations of sediments (TSS), but comparable total N and P (TN, TP), than irrigation events (Nayeb Yazdi et al., 2019). However, TSS, TN and TP storm loads were 900, 35 and 50 times greater than irrigation loads. The practical application of SWMM or similar models needs further evaluation in different nursery layouts, regions and management practices to characterize quality, treatment and management of these sources for recycling or release.
2. Irrigation management and use efficiency
Garden rose cultivars were exposed to deficit irrigation (80%, 50%, 20% ETo) in Utah, evaluating growth, aesthetics, stomatal conductance, foliage temperature. While increasing water stress reduced growth and physiology of most cultivars, some maintained growth and acceptable quality at 20% ETo deficit irrigation treatment (Chen et al., 2023). A California study on groundcovers found that native species (Baccharis, Eriogonum, Rhadogia) can satisfactorily tolerate deficit irrigation of 20% ETo, likely due to their ability to maintain the canopy cooler than air temperature, even in limited irrigation (Sapkota et al., 2023a,b).
Some methods of plant-based irrigation management were reviewed, addressing their advantages and disadvantages in research and commercial settings (Levin and Nackley, 2021). Potential use of these tools in commercial production still requires R&D to overcome their key limitations: prohibitive cost, massive data output, interpretation difficulty and limited dynamic ability to provide decision support. Research from our multistate teams are showing promising results of mini-lysimeter (McCauley et al., 2021) and leaching fraction (Cypher et al., 2022) methodologies for irrigation management in container crops, responding efficiently to diurnal and seasonal crop water demand, increasing applied WUE and reducing leachates (drainage and runoff). These systems more holistically measure whole-plant water use in container crops compared to more technically complex soil/leaf moisture sensors, and thus a higher potential of adoption by commercial growers.
The performance and distribution uniformity (DU) of several irrigation sprinklers at different pressures and wind speeds was recently evaluated (Spinelli et al., 2024), with some having highest DU at low pressures, but DU of most was negatively correlated with wind speed. Further evaluations are needed to allow producers to select sprinklers that best fit their operation and maximize water conservation.
Water footprint (WF) is a broader sustainability concept than WUE, by including estimates of both water applied and water polluted in the production process. The WUE of greenhouse roses ranged from 0.7 to 2.3g dry weight per L of water applied, whereas WF ranged from 8 to 26 liters per single cut flower across a range of nitrogen and irrigation treatments (Cabrera, 2024). Research on the WF of specialty crops is needed, as consumers and markets in some regions are using carbon and water footprints as important criterions to guide their purchases.
3. Runoff management
Management of contaminants by water containment and recycling systems is critical to reduce environmental impacts and optimize intensive plant production. Grower concerns about the presence of plant pathogens, pesticide residues, and salinity potentially limit the willingness of growers to recycle water. Several ongoing research projects in FL, LA, MI, OH, OR, and SC address our capacity to know when, where, and how a contaminant (whether sediment, agrichemical, or pathogen) needs to be managed to optimize plant health in the production setting. Treatment technologies to manage sediment and some agrichemical contaminants exist and have been optimized for other applications. Development and evaluation of treatment technologies (such as bioreactors, filter socks, floating wetlands, activated carbon filters, particle filters, sedimentation ponds, carbon bioreactors, slow-sand filtration etc.) for their utility in mitigation of contaminants (sediment, agrichemical, and pathogen) in high-intensity production systems is ongoing (Abdi et al., 2020, 2024a, 2025a,b; Bell et al., 2019, 2024, 2024; Dekle et al., 2024, 2025; Garcia Chance et al., 2019; Oki et al., 2017; Sahoo et al. 2024; Spangler et al., 2019a, 2019b; White et al., 2019; Yazdi et al., 2021).
Surveys of water quality in nursery recycling and tailwater recovery ponds have been conducted by NC1186 members in CA, FL, MD, NC, SC, and VA (Garcia Chance et al., 2019b). Members in MI and OH have used model production areas to determine how runoff flows are influenced by irrigation method (Abdi et al., 2021a,b, 2024b). NC1186 members from SC, FL, OH, CA, MD, and MI who participated in the Clean WateR3 project (SCRI 2014-51181-22372) have developed information related to which treatment technologies can be used both effectively and economically by green industry producers to contain and recycle water (Abdi et al., 2020, 2024a; Bell et al., 2018, 2024; Garcia Chance et al., 2019; Majstrik et al., 2019; White et al., 2019). Members in LA, MI, OH are investigating production scale treatment systems at cooperator sites to evaluate suitability and effectiveness for commercial operations. The long-term goal is to integrate physical, chemical, and biological technologies in treatment chains in order to reduce contaminant presence in production runoff. NC1186 members are collaborating to develop research projects to address these gaps in knowledge.
4. Substrates and soilless culture
Substrate physical properties are characterized using static (porometer analysis; Fonteno and Bilderback, 1993) and dynamic (moisture characteristic curves; Dane and Hopmans, 2002; Verdonck et al., 1978) procedures. Chemical analysis is conducted using saturated media extract (Warncke, 1986) or pour through analysis (Wright, 1986) during production cycles. These analyses have been used to formulate and model substrates with "ideal" conditions for specific containers with a given geometry (Milks et al., 1989). However, advances in dynamic procedures have led to a more holistic approach to characterize soilless substrates. Techniques that assess water, air, pH, and nutrient flux (Altland and Jeong, 2016; Altland et al., 2018; Criscione et al., 2022), transport gradients (Hoskins et al., 2014a, 2014b; Fields et al., 2017), or consumption (Fields et al., 2018) throughout plant/crop root development are needed to assess and select soilless substrates to deal with increasing challenges in crop production (Caron et al., 2014). As the horticulture industry transitions to more efficient and sustainable production practices, we are presented with challenges to maximize biomass while minimizing resource utilization. To meet these challenges, more intrinsic parameters are needed and deserve greater attention.
Engineering advanced substrates requires investigation into substrate-root interactions (Criscione and Fields, 2024), gas diffusion (Allaire et al., 1996), hydraulic conductivity (Fields et al., 2018), solute transport (Hoskins et al., 2014b), and mineral incorporation to remediate and supply nutrients. Measuring these parameters in situ can provide inferences into electrochemical flux, O2 supply, CO2 exchange, water movement and the interconnectivity between substrate particles, pores, and roots. Understanding thermal transport properties of substrate is required to mitigate supra-optimal temperatures that are becoming increasingly problematic with climate change. Utilizing new methodology and tools will allow scientists to continue to assist stakeholders to improve resource use efficiency with sustainable crop growth.
Substrate stratification, or the layering of unique substrates within a single container (Fields et al., 2021) allows growers to defy gravity by redistributing air and water retention within the container system. Stratification offers growers targeted fertilizer application (Ammons et al., 2022), improved irrigation efficiency (Criscione et al., 2022), improved root growth (Fields et al., 2022), and reducing reliance in peatmoss (Fields and Criscione, 2023). As this new stratification technique gains momentum with stakeholders, researchers aim to optimize individual strata by incorporating materials derived from regional by-products and bio-residues. The concept of stratification can also be used to reduce agrichemical leaching, including the use of ferrous sulfate to reduce phosphorus leaching from containers (Shreckhise and Altland, 2024).
Objectives
-
Water quality of irrigation sources. Characterize the quality of conventional and alternative or non-traditional water sources in different regions of the country. Determine water quality (chemical, physical and biological) parameters and levels that are most limiting for specialty crop production systems and evaluate suitable treatment and irrigation management options.
-
Irrigation management and use efficiency. Determine the water quantity requirements of distinct crop production systems with different container types and environmental conditions. Compare irrigation methods and their effects on crop water use, plant growth and quality, runoff volume and quality. Identify methods and techniques that reduce water use, leaching, and runoff, leading to enhanced crop water use efficiency (WUE) and reduced water footprints. Develop and optimize irrigation methods that can be easily deployed and modulated in intensive crop production systems using real-time information on plant and substrate water status and environmental conditions.
-
Runoff management. Evaluate methods and practices that enhance the containment of irrigation drainage and reduce contaminants in irrigation return flow. Identify improvements to recycled water management, characterizing critical control points within production systems. Develop chemical, physical, and biologically-based water treatment technologies and BMP guidelines that help mitigate the undesirable effects of sediments, agrichemicals, emerging environmental contaminants, and pathogens found in captured runoff intended for recycling in specialty crops.
-
Substrates and soilless culture. Assess physical, chemical, and biological properties of conventional and engineered soilless substrates and components and their impacts on plant growth and quality, water use and recycling, WUE, nutrient delivery and retention, crop fertility and pollution potential in intensively managed specialty crops. Expand knowledge of how soilless substrate systems affect crop biomass and yields, root growth, pathogen and weed pressures, and dynamic physicochemical properties including hydraulic conductivity, pH, cation and anion exchange, plant-water availability, gas exchange and moisture retention.
Methods
This highly collaborative NC1186 team has many years of experience in multistate projects together. Bullets present significant priority areas in each objective, including illustrative examples of projects/activities specific to certain locations.
Methods for (1) Water quality of irrigation sources
- Along with collaborators provide analyses and visualization of water quality issues in conventional and alternative, surface and ground water sources available to specialty crops across USA regions.
- Conduct studies to assess risks from, and crop growth/quality responses to abiotic (salinity, alkalinity, specific ions, etc.) and biotic (biofilm, algae, plant pathogens) quality parameters from irrigation sources, including pesticide residues and emerging contaminants (microplastics, PFAS, pharmaceuticals, etc.).
- Evaluate innovative and emerging technologies that:
- ease monitoring, interpretation and management of current and emerging water quality constituents and contaminants (current and emerging);
- remediate pesticides, pathogens, algae, biofilm, heavy metals and emerging contaminants in conventional and alternative irrigation sources;
- help successfully produce specialty crops with locally available, low quality water sources (alternative, recycled), selecting plants/crops and irrigation practices and equipment most suitable to prevalent and pressing quality issues.
- enhance root health, including dissolved oxygen and beneficial microbes.
- MO, NJ and SC will continue evaluation of and BMPs for use of alternative (reclaimed, graywater) and conventional irrigation waters tainted with salinity (saltwater intrusion, deicing salts).
- FL, MI, NC, USDA-ARS OH and SC will evaluate commercial irrigation catchment basins and recirculation storage tanks to develop case studies and BMPs on monitoring chemical quality, pesticides and emergent contaminants (micro/nano-plastics, PFAS, heavy metals, etc.), turbidity, dissolved oxygen, and recommended remediation methods.
- Lead collaborators: AR, FL, MI, MO, NC, NJ, SC, USDA-ARS OH
Methods for (2) Irrigation management and use efficiency
- Quantify daily and seasonal water demand of crops/plants based on evapotranspiration (ET) and other suitable methods and models. Determine water use efficiency (WUE) and water footprint (WF) of specialty crops in production and landscape environments.
- Determine irrigation efficiency and ROI of conventional and new/novel sprinkler, micro-irrigation, and drip irrigation systems.
- Evaluate use efficiency of fertilization sources/methods as affected by irrigation management.
- Measure plant responses to incipient, intermittent and sustained drought stress to help determine when irrigation is needed to sustain growth and quality. Parameters to be measured include changes in plant growth/morphology/quality (canopy size, stem diameter, growth indices, aesthetics, etc.) and physiology (canopy temperature & reflectance, leaf transpiration, photosynthesis and chlorophyll fluorescence, etc.).
- CA will study the impact of irrigation management (volumetric water content and matric tension) and chemical/biological treatments on the incidence of diseases (i.e. Phytophthora) in containerized ornamental annual crops. Researchers will also evaluate nitrogen leaching from container crops as affected by new and alternative substrates and irrigation management.
- MD will evaluate precision agriculture technologies like wireless sensor networks to help determine and control water delivery, and unpiloted aerial systems to detect crop stress and assist production managers make informed decisions on irrigation and fertilizer applications.
- NJ will continue evaluation of WUE and WF of container nursery and greenhouse crops.
- OR and CA will continue irrigation efficiency studies for sprinklers and micro-irrigation systems/emitters. Distribution uniformity, wear, influence of wind and other parameters will be tested. A reference ET guide will be developed to improve irrigation scheduling in greenhouse crops.
- Lead collaborators: CA, MD, MI, NJ, OR, SC, TN, USDA-ARS TN
Methods for (3) Runoff management
- Identify, adapt, and assess select filtration and chemically- and biologically based treatment technologies to manage nutrient, pesticide, particulate, pathogenic, and biological contaminants in production runoff.
- Develop and evaluate treatment technologies at laboratory, pilot, and commercial scales, focusing on the performance of individual and integrated treatment systems.
- Evaluate bioreactors hydraulic retention times, redox status, and placement to improve water quality as appropriate to recycling (i.e. remove pesticides but retain nutrients).
- MI will determine ideal combination and placement of treatment technologies in series to remove/remediate various agrochemicals from discharged water.
- FL will evaluate efficacy of physical particle filters and flocculation to remove suspended particles and increase water clarity in case study commercial irrigation reservoir sites.
- USDA-ARS, MI, and SC will develop Fe-enriched substrates that sorb or to filter phosphates from container leachates or other off-site release (remove all agrochemicals).
- Lead collaborators: FL, MI, NC, SC, USDA-ARS OH, USDA-ARS TN
Methods for (4) Substrates and soilless culture
- Hydraulic balance of substrate stratification practices will be evaluated to optimize pairing strata for improved water use efficiency (WUE). Water and air balance within individual layers and across the entire profile will be engineered to create uniform moisture gradients which growers can deploy to enhance WUE and nutrient use efficiency (NUE).
- Physical-chemical properties of different substrates will be thoroughly and comparatively evaluated. Relationship between substrate properties, nutrient and plant growth will be further evaluated.
- Substrate hydraulic characteristics are measured mostly on fallow profiles, with limited information on root development effects on substrate storage capacity and hydraulics. Thus, the relationship between in-situ root development and substrate hydraulic properties will be explored with predictive water infiltration models (e.g., HYDRUS). Plants will be grown in cores at different time intervals to achieve progressive increases in rooting levels. Root morphology features (length, diameter, surface area, volume, fine vs coarse root ratios) will be measured at each harvest interval to find correlations across substrate characteristics.
- The mechanism of substrate decomposition will be thoroughly explored from chemical and biological standpoints. Substrates will be evaluated for their initial composition (lignin, cellulose, hemi-cellulose), stability and microbial communities over 2, 6, and 12 months in simulated production to understand how microbial community dynamics affect substrate decomposition. Linking compositional analysis, stability analysis, and microbial communities of substrates will provide a comprehensive understanding of structural and biological factors that influence substrate decomposition over time. Compositional analysis and microbiome will be correlated to substrate stability to determine if these values determined in the laboratory can predict substrate physical stability in a broad spectrum of organic substrates.
- "Cyclic" or multiple irrigation events of equal time are used to replace crop daily water loss via ET to increase application efficiency, increase substrate water availability, and lower substrate temperature throughout the day. Scheduling of equal cyclic irrigation events is inefficient due to preferential flow of solutes in bark-based substrates, and will be further optimized by altering timing of individual application volumes to better reflect substrate water availability, further minimizing amounts of water and agrichemicals leached. Crop response and WUE will be determined under varying cyclic irrigation schedules in conventional and stratified bark substrate of varying texture (fine to coarse).
- Recent reports have shown that when stratified containers are cyclically irrigated, there is more uniformity in moisture gradients, with greater water availability in the top layer. The relationships between cyclical irrigation and stratified systems will be more deeply examined to understand how root morphology is influenced temporally and spatially. In-situ root measurements will be correlated with substrate water availability across the stratified layers.
- LA, NC and USDA-ARS OH will evaluate hydraulic characteristics of conventional and stratified substrates. These teams will also assess the stability/decomposition of various organic components and their effect on media physical properties.
- LA and VA will assess dynamics and relationships between crop root growth and substrate physical properties.
- USDA-ARS OH will study microbial community analyses in traditional, emergent and engineered substrates.
- GA will continue studies on additions of biochar amendments to improve properties of soilless substrates and enhance crop growth and quality.
- Lead collaborators: LA, GA, MI, NC, TN, USDA-ARS OH, USDA-ARS TN, VA
Measurement of Progress and Results
Outputs
- Improved strategies and guidelines for efficient irrigation management which reduces water and fertilizer use while maintaining crop yields/quality and minimizing environmental impacts.
- Develop and/or improve viable strategies to secure, capture, store, treat and use available conventional and alternative water sources for specialty crops, ensuring their sustainability and climate resilience.
- Refereed journal publications for the scientific community and industry/extension articles and factsheets for stakeholders. Presentations at grower meetings and scientific events. Serve as a clearinghouse for publications and speakers to regional, national and international trade journals, conferences, workshops, etc. Member websites will be used to disseminate information generated from this multistate group.
- Continue applying for regional and national grants to fund research, students and extension outreach activities.
Outcomes or Projected Impacts
- Conservation of water resources by specialty growers will leave more freshwater/good-quality water available for other priority and beneficial uses.
- Reduced use and costs of water, fertilizers, energy and labor (increased resource use efficiency), along with reductions in water and agrichemical effluents (environmental benefits) and sustained or increased crop productivity and quality (plant growth benefits).
- Reducing volumes and contaminant loads from agricultural runoff/tailwaters will lead to decreased carbon and water footprints of operations and help protect the quality of adjacent ground and surface water bodies.
- An enhanced and applicable understanding of improved and engineered soilless substrates will amplify water and fertilizer use efficiencies and reduce their footprints. This includes development of integrated substrate/irrigation/nutrition models for management of crops in greenhouses and outdoor nurseries.
- Knowledge of crop responses to alternative and recycled water sources can help specialty crop and green industry professionals, and homeowners to identify and choose appropriate plants and irrigation practices for urban landscapes where only alternative and low-quality water sources will be allowed.
Milestones
(1):Continue ID of key quality issues of regionally available water sources, including their securing and storage (catchment basins, storage reservoirs and tanks, etc.). Identify and address knowledge gaps in specific water quality issues, treatment technologies and irrigation BMPs for their proper use. Carry out studies on plant selection and responses to current and emergent water contaminants (thru 5-year period). Develop and refine engineered substrates with improved properties. Continue investigation of precision ag technologies to be implemented in ornamental crop production systems including wireless networks and drones for monitoring crop environment (thru 5 year period). Present regional-national seminars and workshops covering all objectives/activities with as many project participants (thru entire 5-year project).(2):On Years 2 to 4 of this project we will continue crop growth response and irrigation studies with alternative/recycled waters with challenging qualities. Interpret regional water quality and production practices data, ID key and emerging issues, and run targeted trials to refine and demonstrate suitable treatment/management options. Evaluate technologies at laboratory and commercial scale for managing most challenging water quality issues. Compare and validate performance of improved/engineered substrates (physical, chemical, biological). Field measure efficiency and runoff characteristics of irrigation emitters and layouts in production scenarios. Continue development of irrigation techniques and strategies to reduce water use and footprints, improve efficiency and operational water security, and reduce contaminant loads in runoff. Identify cost-effective knowledge, technologies and practices to reduce current and emergent chemical, physical and biological contaminants in recycled water for specialty crop production. Devise and improve measurement and monitoring of static and dynamic substrate properties along with their short- and long-term impacts on crop shoot-root responses and transport and fate of water and agrichemicals. Validate the integrated substrate/agrichemical/irrigation model. Peer-refereed and extension publications for disseminating information to green industry.
(3):Integrate knowledge and outreach, including case studies on water conservation and treatment options at selected sites. Update BMP guidelines for production and landscape irrigation, plant selection, runoff capture, water recycling/treatment, and soilless substrate selection/management.
Projected Participation
View Appendix E: ParticipationOutreach Plan
This multistate group will deliver face-to-face presentations to industry and academia at regional, national and international meetings. Examples of these meetings are the Annual Conference of the American Society for Horticultural Science, symposia of the International Society for Horticultural Science, the International Horticultural Congress (2026), grower association meetings such as International Plant Propagators’ Society, Farwest (OAN), Cultivate (AmericanHort), and specialized workshops organized around dates and locations of annual meetings of NC1186. An irrigation management workshop will be developed for Spanish-speaking workers from field nursery and greenhouse crops, with theoretical principles and practical applications suitable to these audiences, including hands-on demonstrations on irrigation methods and their monitoring, and irrigation application efficiency.
In order to increase the international impact of this project, our goal is to include overseas workshops with other research/extension colleagues and grower organizations, similar to that organized by NC1186 collaborators with the Seedling Growers Assn. of South Africa in 2023 (Fisher, 2023).
Peer-reviewed publications, extension bulletins and factsheets, and popular press articles will be generated. Online extension training courses on irrigation and substrates will be developed for the Greenhouse Training Online program (hort.ifas.ufl.edu/training, Freyre et al., 2018) and asynchronous online modules (Marble et al., 2016) covering advanced nursery irrigation and substrates concepts will be revised or created and posted in the Tennessee Master Nursery Producer program (www.tnmasternursery.com).
Organization/Governance
This multistate project has a leadership composed of a secretary, vice-chair, and chair who are elected every year by the annual meeting participants. These positions have one-year terms, where responsibilities progress in order from secretary to vice-chair to chair. The project has an annual meeting, with location varying each year, discussed at each annual meeting and voted thereafter (via online poll) by all membership, identifying the date(s) and place that will ensure a wider attendance. The annual meeting activities are summarized, and minutes prepared by the secretary and distributed to all membership for edits/corrections. The annual reports are submitted by the current chair into NIMSS. All members have voting rights.
Literature Cited
Abdi, D.E., J.S. Owen Jr., J.C. Brindley, A.C. Birnbaum, P.C. Wilson, F.O. Hinz, G. Reguera, J. Lee, B.M. Cregg, D.R. Kort, R.T. Fernandez. 2020. Nutrient and pesticide remediation using a two-stage bioreactor-adsorptive system under two hydraulic retention times. Water Research 170, 115311. https://doi.org/10.1016/j.watres.2019.115311
Abdi, D.E., J.S. Owen Jr., P.C. Wilson, F.O. Hinz, B.M. Cregg, R.T. Fernandez. 2021a. Reducing pesticide transport in surface and subsurface irrigation return flow in specialty crop production. Agr. Water Mgmt. 256: 107124. https://doi.org/10.1016/j.agwat.2021.107124
Abdi, D.E., J.S. Owen Jr., J.C. Brindley, A.C. Birnbaum, P.C. Wilson, F.O. Hinz, B.M. Cregg, R.T. Fernandez. 2021b. Irrigation return flow and nutrient movement mitigation by irrigation method for container plant production. Irrigation Sci. 39:567-585. https://link.springer.com/article/10.1007/s00271-021-00727-1
Abdi, D.E., M. Tabares, N. Redekar, J. Parke, G. Reguera, R.T. Fernandez. 2024a. Control of microbiome composition and agrichemical remediation in woodchip bioreactors by hydraulic retention time. Environ. Sci. & Technol. Submitted, In-review
Abdi, D.E., J.S. Owen Jr., J.C. Brindley, A.C. Birnbaum, P.C. Wilson, F.O. Hinz, B.M. Cregg, R.T. Fernandez. in-review 2024b. Irrigation scheduling based on substrate moisture sensors conserves water and reduces nutrient movement without affecting growth of container produced crops. Agr. Water Mgmt. Submitted, In-review
Abdi, D.E., B. Slade, J.S. Fields, M.P. Hayes, M. Ahmed, J. Beasley, and J. Kuehny. Submission in 2025. Evaluating blended bioreactor media for nutrient remediation and seedling establishment. Science of the Total Environment. In Preparation.
Abdi, D.E., B. Slade, J.S. Fields, M.P. Hayes, J. Beasley, and J. Kuehny. Submission in 2025. Assessing bioretention cell infiltration layer media to remediate nutrients and support establishment of Hibiscus moscheutos ‘Luna’. Science of the Total Environment. In Preparation
Alexandrino, D.A., Almeida, C.M.R., Mucha, A.P., Carvalho, M.F. 2022. Revisiting pesticide pollution: The case of fluorinated pesticides. Environmental Pollution 292, 118315. https://doi.org/10.1016/j.envpol.2021.118315
Allaire, S.E., J. Caron, I. Duchesne, L.-E. Parent, and J.A. Rioux. 1996. Air-filled porosity, gas relative diffusivity, and tortuosity: Indices of Prunus × cistena growth in peat substrates. J. Am. Soc. Hortic. Sci. 121: 236–242.
Altland, J.E. and K.Y. Jeong. 2016. Dolomitic lime amendment affects pine bark substrate pH, nutrient availability, and plant growth: A review. HortTechnology 26: 565–573. https://doi.org/10.21273/horttech03465-16
Altland, J.E., and K.Y. Jeong. 2018. Initial substrate moisture content and storage temperature affect chemical properties of bagged substrates containing poultry litter fertilizer. HortScience 53: 1191-1196. https://doi.org/10.21273/HORTSCI13004-18
Altland, J.E., J.S. Owen, B.E. Jackson, and J.S. Fields. 2018. Physical and hydraulic properties of commercial pine-bark substrate products used in production of containerized crops. HortScience 53: 1883-1890.
Ammons, A., LeBude, A.V., Owen Jr, J.S., McGinnis, M. 2022. Effect of irrigation, fertilizer rate and placement, and two substrates on growth of rose and hydrangea. J. Env. Hort. 40: 123-128. https://doi.org/10.24266/2573-5586-40.3.123
Bale, A.E., S.E. Greco, B.J.L. Pitton, D.L. Haver, and L.R. Oki. 2017. Pollutant loading from low density residential neighborhoods in California. Environmental Monitoring and Assessment. 189:386. DOI:10.1007/s10661-017-6104-2.
Basiri-Jahromi, N., A. Fulcher, F. Walker, and J.E. Altland. 2020. Photosynthesis, growth, and water use of Hydrangea paniculata ‘Silver Dollar’ using a physiological-based or a substrate physical properties-based irrigation schedule and a biochar substrate amendment. Irrigation Science 38, 263–274. https://doi.org/10.1007/s00271-020-00670-7
Baz, M. and R.T. Fernandez. 2002. Evaluating woody ornamentals for use in herbicide phytoremediation. J. Amer. Soc. Hort. Sci. 127:991-997.
Bell, NL, SN Jeffers, SA White. 2021. Potential susceptibility of six aquatic plant species to infection by five species of Phytophthora. Plant Disease. 105:4074-4083. https://doi.org/10.1094/PDIS-10-20-2190-RE
Bell, NL, NR Redekar, SN Jeffers, DR Hitchcock, JL Parke, SA White. 2024. A model system to evaluate the potential of floating treatment wetlands to manage Phytophthora species in recycled irrigation water. J. Nat. Res. Agric. Ecosys. 2: 103-118. https://doi.org/10.13031/jnrae.15949
Briggs J, T. Whitwell, R.T. Fernandez, M.B. Riley. 2002. Effect of integrated pest management strategies on chlorothalonil, metalaxyl, and thiophanate-methyl runoff at a container nursery. J. Amer. Soc. Hort. Sci. 127: 1018-1024.
Burga, S. 2023. What to know about the saltwater threat to Louisiana’s drinking water supply. Time Magazine – US: Climate Change, September 24, 2023. Accessed on September 18, 2024, from: https://time.com/6317056/saltwater-threat-mississippi-river-drinking-water/
Cabrera, R.I. 2021. Irrigation and nutrition management, p. 224-257. In: J. Faust and J. Dole (eds.) Cut Flowers and Foliages, Crop Production Book Series, CABI, Wallingford, UK
Cabrera, R.I. 2024. From water use efficiency to water footprint in greenhouse roses. Acta Hort. (Nov. 2024) DOI: 10.17660/ActaHortic.2024.1409.33
Cabrera, R.I. and J.E. Altland. 2024. Residential graywater as an irrigation source for urban landscape plants. Acta Hort. (Nov. 2024) DOI: 10.17660/ActaHortic.2024.1409.25
Cabrera, R.I., Altland, J.E. and Niu, G. 2018. Assessing the potential of nontraditional water sources for landscape irrigation. HortTechnology 28(4): 436-444. https://doi.org/10.21273/HORTTECH04023-18
California Water Boards. 2024. Irrigated Lands Regulatory Program. State Water Resources Control Board, Sacramento, CA. Accessed on September 19, 2024, from: https://www.waterboards.ca.gov/water_issues/programs/agriculture/
Caron, J. and J-C. Michel. 2021. Understanding and optimizing the physical properties of growing media for soilless cultivation, p. 107-137. In: N.S. Gruda (ed.) Advances in Horticultural Soilless Culture, Burleigh Dodds Science Pub. Ltd. UK
Caron, J., S. Pepin, and Y. Periard. 2014. Physics of growing media in the future. Acta. Hort. 1034: 309-318.
Chen, J-J., Y. Sun, L. Oki, J. Sisneroz, K. Reid, L.L. Nackley, R.N. Contreras, S. Kim, U. Schuch, D. Haver, M. Stucke, A. Fron, K. Kopp, S. Jones, and L. Hipps. 2023. Climate-ready landscape plants: Garden roses trialed at reduced irrigation frequency in Utah, USA. HortTechnology, 33: 477–492. https://doi.org/10.21273/HORTTECH05252-23
Criscione, K.S. and J.S. Fields. 2024. Root growth and development in soilless culture – A review. Acta Hortic. 1389: 1-16. DOI: 10.17660/ActaHortic.2024.1389.1
Criscione, K.S., J.S. Fields, and J.S. Owen, Jr. 2022. Root exploration, initial moisture conditions, and irrigation scheduling influence hydration of stratified and non-stratified substrates. Horticulturae 8:826. https://doi.org/10.3390/horticulturae8090826
Criscione, K.S., J.S. Fields, J.S. Owen, L. Fultz, and E. Bush. 2022. Evaluating stratified substrates effect on containerized crop growth under varied irrigation strategies. HortScience 57: 400-413. https://doi.org/10.21273/hortsci16288-21
Cypher, Q., W.C. Wright, X. Sun, L. Fessler, and A. Fulcher. 2022. Automated leaching fraction-based irrigation system reduces leaching, conserves water, and supports crop growth in a commercial nursery. Applied Engineering in Agriculture 38: 807-816. DOI: 10.13031/aea.15082
Dane, J., J. Hopmans. 2002. Water retention and storage/laboratory, pp. 675-720. In: J.H. Dane and G.C. Topp (eds.), Methods of Soil Analysis, Part 4, Physical Methods, Soil Sci. Soc. of Am., Madison, WI.
Dekle, J, WHJ Strosnider, SA White. 2024a. Phosphorus uptake and release patterns in overwintering constructed floating wetlands. Water Sci. & Tech. 89, 588 https://doi.org/10.2166/wst.2024.010
Dekle, J, WHJ Strosnider, SA White. 2025. Removal of phosphorus from irrigation return flow using an iron oxide filter and denitrifying pine bark bioreactor treatment. Env. Sci. Poll. Res. In Print.
Dieter, C.A., Maupin, M.A., Caldwell, R.R., Harris, M.A., Ivahnenko, T.I., Lovelace, J.K., Barber, N.L., and Linsey, K.S. 2018. Estimated use of water in the United States in 2015. U.S. Geological Survey Circular 1441, 65 p. https://doi.org/10.3133/cir1441
Donley, N., Cox, C., Bennett, K., Temkin, A.M., Andrews, D.Q., Naidenko, O.V. 2024. Forever pesticides: A growing source of PFAS contamination in the environment. Environmental Health Perspectives 132(7), 075003. DOI:10.1289/EHP13954.
Duncan, R.R., R. Carrow, and M.T. Huck. 2009. Turfgrass and Landscape Irrigation Water Quality: Assessment and Management. CRC Press, Boca Raton, FL.
Fields, J.S. and K. Criscione. 2023. Stratified substrates can reduce peat use and improve root productivity in container crop production. HortScience 58: 364-372. https://doi.org/10.21273/HORTSCI17019-22
Fields, J.S., K.S. Criscione, A. Edwards. 2022. Single-screen bark particle separation can be used to engineer stratified substrate systems. HortTechnology 32: 391-397. https://doi.org/10.21273/HORTTECH05018-22
Fields, J.S., J.S. Owen, and J.E. Altland. 2021. Substrate stratification: Layering unique substrates within a container increases resource efficiency without impacting growth of shrub rose. Agronomy 11, 1454. https://doi.org/10.3390/agronomy11081454
Fields, J.S., J.S. Owen, Jr., and H. Scoggins. 2017. The influence of substrate hydraulic conductivity on plant water status of ornamental container crop grown in sub-optimal substrate water potentials. HortScience 52:1419-1428
Fields, J.S., J.S. Owen, Jr., J.E. Altland, M.W. van Iersel, and B.E. Jackson. 2018. Soilless substrate hydrology can be engineered to influence plant water status for an ornamental containerized crop grown within optimal water potentials. J. Amer. Soc. Hort. Sci. 143:268-281.
Fisher, P.R. 2023. Workshop in South Africa helps nursery growers conserve irrigation water. FloralDaily Feb 21 2023, https://www.floraldaily.com/article/9505259/workshop-in-south-africa-helps-nursery-growers-conserve-irrigation-water/
Fonteno, W.C. and T.E. Bilderback. 1993. Impact of hydrogel on physical properties of coarse-structured horticultural substrates. J. Amer. Soc. Hort. Sci. 118: 217-222. https://doi.org/10.21273/JASHS.118.2.217
Fork, M.L., J.B. Fick, A.J. Reisinger, and E.J. Rosi. 2021. Dosing the coast: Leaking sewage infrastructure delivers large annual doses and dynamic mixtures of pharmaceuticals to urban rivers. Env. Sci. & Tech. 55(17): 11637-11645. DOI:10.1021/acs.est.1c00379
Freyre, R., B.J. Pearson, and P.R. Fisher. 2018. International training on greenhouse production using an online platform. Acta Horticulturae 1205:293-297.
Fulcher, A., LeBude, A.V., Owen, J.S., Jr., White, S.A., and Beeson, R.C. (2016). The next ten years: Strategic vision of water resources for nursery producers. HortTechnology 26(2): 121-132. https://doi.org/10.21273/HORTTECH.26.2.121
Garcia Chance, L.M., S.C. van Brundt, J.C. Majsztrik, and S.A. White. 2019. Short- and long-term dynamics of nutrient removal in floating treatment wetlands. Water Research 159: 153-163.
Giuffrida, F., R.P. Mauro, and P. Zaccheo. 2021. Understanding and optimizing the biological properties of growing media for soilless cultivation, p. 171-210. In: N.S. Gruda (ed.) Advances in Horticultural Soilless Culture, Burleigh Dodds Science Pub. Ltd. UK
Gottlieb, P.D., R.G. Brumfield, R.I. Cabrera, D. Farnsworth, and L. Marxen. 2022. An online tool for estimating return-on-investment for water recycling at nurseries. HortTechnology 32: 47-56. https://doi.org/10.21273/HORTTECH04925-21
Grieve, C.M., S.R. Grattan, and E.V. Maas. 2012. Plant salt tolerance, p. 405–459. In: W.W. Wallender and K.K. Tanji (eds.). Agricultural Salinity Assessment and Management, 2nd Ed. Manual Rpt. Eng. Practice No. 71. Amer. Soc. Civil Eng., Reston, VA.
Hoskins, T., J.S. Owen, Jr., and A.X. Niemiera. 2014a. Water movement through a pine-bark substrate during irrigation. HortScience 49: 1432-1436. https://doi.org/10.21273/HORTSCI.49.11.1432
Hoskins, T., J.S. Owen, Jr., J.S. Fields, J.E. Altland, Z. Easton, and A.X. Niemiera. 2014b. Solute transport through a pine bark-based substrate under saturated and unsaturated conditions. J. Amer. Soc. Hort. Sci. 139: 634-641. https://doi.org/10.21273/JASHS.139.6.634
Jiang, M., M.C Fleetwood, S.H. Anderson and X. Xiong. 2022. Wetting agent effects on plant available water for hydrophobic USGA root zones. Agricultural Research & Technology 27 (1), DOI: 10.19080/ARTOAJ.2022.27.556360
Judd, L.A., B.E. Jackson, and W.C. Fonteno. 2015. Rhizometer: An apparatus to observe and measure root growth and its effect on container substrate physical properties over time. HortScience 50: 288-294. https://doi.org/10.21273/HORTSCI.50.2.288
Kaushal, S.S., Likens, G.E., Mayer, P.M. et al. 2023. The anthropogenic salt cycle. Nature Reviews Earth & Environment 4:770–784. https://doi.org/10.1038/s43017-023-00485-y
Langenfeld, N.J., D.F. Pinto, J.E. Faust, R. Heins and B. Bugbee. 2022. Principles of nutrient and water management for indoor agriculture. Sustainability 14, 10204. https://doi.org/10.3390/su141610204
Lea-Cox, J.D. and D.S. Ross. 2018. Managing water and nutrients to reduce environmental impact, p. 273-288. In: D. Merhaut (ed.), Water and Nutrient Management for Greenhouse Crops. Publication No. 3551. University California Agriculture and Natural Resources Communication Resources.
Levin, A. and L.L. Nackley. 2021. Principles and practices of plant-based irrigation management. HortTechnology, 31:650-660. https://doi.org/10.21273/HORTTECH04862-21
Majsztrik, J.C. and Lea-Cox, J. D. 2013. Water quality regulations in the Chesapeake Bay: Working to estimate nutrient loading rates and incentivize best management practices in the nursery and greenhouse industry. HortScience 48(9): 1097-1102. https://doi.org/10.21273/HORTSCI.48.9.1097
Majsztrik, J.C., R.T. Fernandez, P.R. Fisher, D.R. Hitchcock, J.D., Lea-Cox, J.S. Owen, L.R. Oki, and S.A. White. 2017. Water use and treatment in containerized specialty crop production: A review. Water, Air & Soil Pollution. 228: 151, 27p.
Majsztrik, J.C., A.G. Ristvey, D.S. Ross and J.D. Lea-Cox. 2018a. Comparative water and nutrient applications among ornamental operations in Maryland. HortScience 53: 1364-1371.
Majsztrik, J.C., D.R. Hitchcock, S. Kumar, D. Sample, S.A. White. 2018b. Clean WateR3: Developing tools to help specialty crop growers understand the costs and benefits of recycling water. Acta Horticulturae 1191: 187-192
Majsztrik, J.C., W.H.J. Strosnider, M.E. Chase, L.M. Garcia Chance, and S.A. White. 2019. Phosphorus removal from nursery runoff using pilot scale filters. SNA Research Conf. Proc. 63: 147-149.
Marble, S.C., A. Fulcher, and J. Toman. 2016. Advantages and disadvantages of asynchronous online extension programming for delivering master producer content. HortTechnology 26: 584-587. https://doi.org/10.21273/HORTTECH03410-16
McCabe, G. J., Wolock, D. M., Lombard, M., Dudley, R. W., Hammond, J. C., Hecht, J. S., Hodgkins, G. A., Olson, C., Sando, R., Simeone, C., and Wieczorek, M. 2022. A hydrologic perspective of major U.S. droughts. Int. J. Climatol., 1–17. https://doi.org/10.1002/joc.7904
McCauley, D.M., A. Levin, and L.L. Nackley. 2021. Reviewing mini-lysimeter-controlled irrigation in container crop systems. HortTechnology, 31:634- 641. https://doi.org/10.21273/HORTTECH04826-21
Milks, R.R., W.C. Fonteno, and R.A. Larson. 1989. Hydrology of horticultural substrates: II. Predicting physical properties of substrate in containers. J. Amer. Soc. Hort. Sci. 114:53- 56.
Milman, O. and G. Canon. 2023. US states agree breakthrough deal to prevent Colorado River from drying up. The Guardian, May 22, 2023. Accessed on September 18, 2024, from: https://www.theguardian.com/us-news/2023/may/22/colorado-river-states-california-arizona-nevada
National Agricultural Statistics Service (NASS). 2020. 2019 Census of Horticultural Specialties, Vol. 3 - Special Studies - Part 3, AC-17-SS-3. US Dep. of Agriculture – Nat. Agric. Stat. Serv. Washington, DC. https://www.nass.usda.gov/Publications/AgCensus/2017/Online_Resources/Census_of_Horticulture_Specialties/index.php
Nayeb Yazdi, M., D.J. Sample, D. Scott, J.S. Owen, M. Ketabchy, and N. Alamdari. 2019. Water quality characterization of storm and irrigation runoff from a container nursery. Science of the Total Environment 667: 166-178. https://doi.org/10.1016/j.scitotenv.2019.02.326
Nemati, M., D. Tran, and K. Schwabe. 2023. Residential water conservation and the rebound effect: A temporal decomposition and investigation. Water Resources Research 59(4). https://doi.org/10.1029/2022WR032169
Niu, G. and R.I. Cabrera. 2010. Growth and physiological responses of landscape plants to saline water irrigation - A review. HortScience 45: 1605-1609.
Nyberg, E.T., S.A. White, S.N. Jeffers, and W.C. Bridges. 2014. Removal of zoospores of Phytophthora nicotianae from irrigation runoff using slow filtration systems: quantifying physical and biological components. Water, Air, & Soil Pollution. 225: 1999, 11p.
Oki, L.R., S. Bodaghi, E. Lee, D. Haver, B. Pitton, L. Nackley, and Mathews, D.M. 2017. Elimination of tobacco mosaic virus from irrigation runoff using slow sand filtration. Scientia Horticulturae 217: 107-113. https://doi.org/10.1016/j.scienta.2017.01.036.
Owen, J.S., J.S. Fields, and J.E. Altland. 2022. Defying gravity: Utilizing stratified growing media to invent the future. Nursery Management, March 2022. Accessed on 23 September 2024 at: https://www.nurserymag.com/article/stratification-growing-media-research/
Pershey, N.A., B.M. Cregg, J.A. Andresen, and R.T. Fernandez. 2015. Irrigating based on daily water use reduces nursery runoff volume and nutrient load without reducing growth of four conifers. HortScience 50: 1553-1561.
Pitton, B.J., C.R. Hall, D.L. Haver, S.A. White, and L.R. Oki. 2018. A cost analysis for using recycled irrigation water in container nursery production: A southern California nursery case study. Irrigation Science. https://doi.org/10.1007/s00271-018-0578-8
Quijia-Pillajo, J., Chapin, L.J., Quiroz-Moreno, C.D., Altland, J.E., and Jones, M.L. 2024. Nutrient availability and plant phenological stage influence the substrate microbiome in container-grown Impatiens walleriana. BMC Plant Biology 24, 176. https://doi.org/10.1186/s12870-024-04854-7
Raudales, R.E., J.L. Parke, C.L. Guy, and P.R. Fisher. 2014. Control of waterborne microbes in irrigation: A review. Agricultural Water Management 143: 9-28.
Raudales, R.E., P.R. Fisher and C.R. Hall. 2017. The cost of irrigation sources and water treatment in greenhouse production. HortScience 35: 43-54.
Raudales, R.E., Toro-Herrera, M.A., Fisher, P.R., Boldt, J.K., and Altland, J.E. 2024. Paclobutrazol residues in recirculated water in commercial greenhouses. HortTechnology, 34: 198-203. https://doi.org/10.21273/HORTTECH05367-23
Redekar, N.R., J.L. Eberhart, J.L. Parke. 2019. Diversity of Phytophthora, Pythium, and Phytopythium species in recycled irrigation water in a container nursery. Phytobiomes J. 3(1): 31-45.
Sahoo, D, I Busari, HB Nix, SA White. 2024. Impacts of harmful algal blooms on agriculture: a short communication. J. of South Carolina Water Resources 9: 45-50.
Sapkota, A., Haghverdi, A., Merhaut, D., Singh, A., Iradukunda, J.C. 2023a. Response of landscape groundcovers to deficit irrigation: An assessment based on NDVI and visual quality rating. HortScience 58: 274-285. https://doi.org/10.21273/HORTSCI16915-22
Sapkota, A., Haghverdi, A., and Merhaut, D. 2023b. Effects of deficit irrigation on canopy temperature dynamics and physiology of landscape groundcovers. HortScience 58: 1321-1331. https://doi.org/10.21273/HORTSCI17303-23
Shreckhise, J.H. and J.E. Altland. 2024. A base layer of ferrous sulfate-amended pine bark reduces phosphorus leaching from nursery containers. Agronomy 14(4), 757 https://doi.org/10.3390/agronomy14040757
Spangler, J.T., D.J. Sample, L.J. Fox, J.S. Owen, Jr., S.A. White. 2019a. Floating treatment wetland aided nutrient removal from agricultural runoff using two wetland species. Ecol. Eng. 127: 468-479.
Spangler, J.T., D.J. Sample, L.J. Fox, J.P. Albano, S.A. White. 2019b. Assessing nitrogen and phosphorus removal potential of five plant species in floating treatment wetlands receiving simulated nursery runoff. Environ. Sci. Pollut. Res. 26: 5751-5768. https://doi.org/10.1007/s11356-018-3964-0
Spinelli, G., A.C. Bonarrigo, W. Cui, K. Grobowsky, S.H. Jordan, K. Ondris, C. Prieto-García, K. Redding, K. Zalis-Waldman, H.E. Dahlke. 2024. Evaluating the distribution uniformity of ten overhead sprinkler models used in container nurseries. Agricultural Water Management 303: 109042, https://doi.org/10.1016/j.agwat.2024.109042
Thakulla, D. and P.R. Fisher. 2023. Effects of cold plasma and ozone water treatment on micronutrient solubility. Horticulturae 9(5), 568. https://doi.org/10.3390/horticulturae9050568
Thornton-Hampton, L.M., Bouwmeester, H., Brander, S.M., Coffin, S., Cole, M., Hermabessiere, L., Mehinto, A.C., Miller, E., Rochman, C.M., Weisberg, S.B., 2022. Research recommendations to better understand the potential health impacts of microplastics to humans and aquatic ecosystems. Microplastics and Nanoplastics 2, 18. https://doi.org/10.1186/s43591-022-00038-y
Valles-Ramirez, S., Altland, J.E., Testen, A.L., Poelstra, J.W., and Michel, F.C. 2023. Microbial community structure in soilless substrates used for nursery crops. HortScience 58: 1348-1357. https://doi.org/10.21273/HORTSCI17275-23
Verdonck, O.F., T.M. Cappaert, and M.F. de Boodt. 1978. Physical characterization of horticultural substrates. Acta Hortic. 82: 191-200. DOI: 10.17660/ActaHortic.1978.82.26
Warncke, D.D. 1986. Analyzing greenhouse growth media by the saturation extraction method. HortScience 21: 223-225.
Warsaw, A.L., R.T. Fernandez, D.R. Kort, C. Vandervoort, B.M. Cregg, and D.B. Rowe. 2012. Remediation of metalaxyl, trifluralin, and nitrates from nursery runoff using container-grown woody ornamentals. Ecological Engineering 47:254-263.
White, S.A., L.M. Garcia Chance, N.L. Bell, M.E. Chase. 2019. Potential and problems of floating treatment wetlands for mitigating agricultural contaminants. Wetland Sci. & Practice 36: 119-124.
Wright, R.D. 1986. The pour-through nutrient extraction procedure. HortScience 21: 227-229.
Yazdi, MN, JS Owen, SW Lyon, and SA White. 2021. A critical review of irrigation retention reservoir performance and design considerations to enhance water security for specialty crops. J. Cleaner Produc. 321, 128925 https://doi.org/10.1016/j.jclepro.2021.128925
Yvraj, K., Marble, C., Altland, J.E., Pearson, B., Chen, J., Devkota, P. 2022. Effect of substrate stratification on growth of common nursery weed species and container-grown ornamental species. HortTechnology 32:74-83.