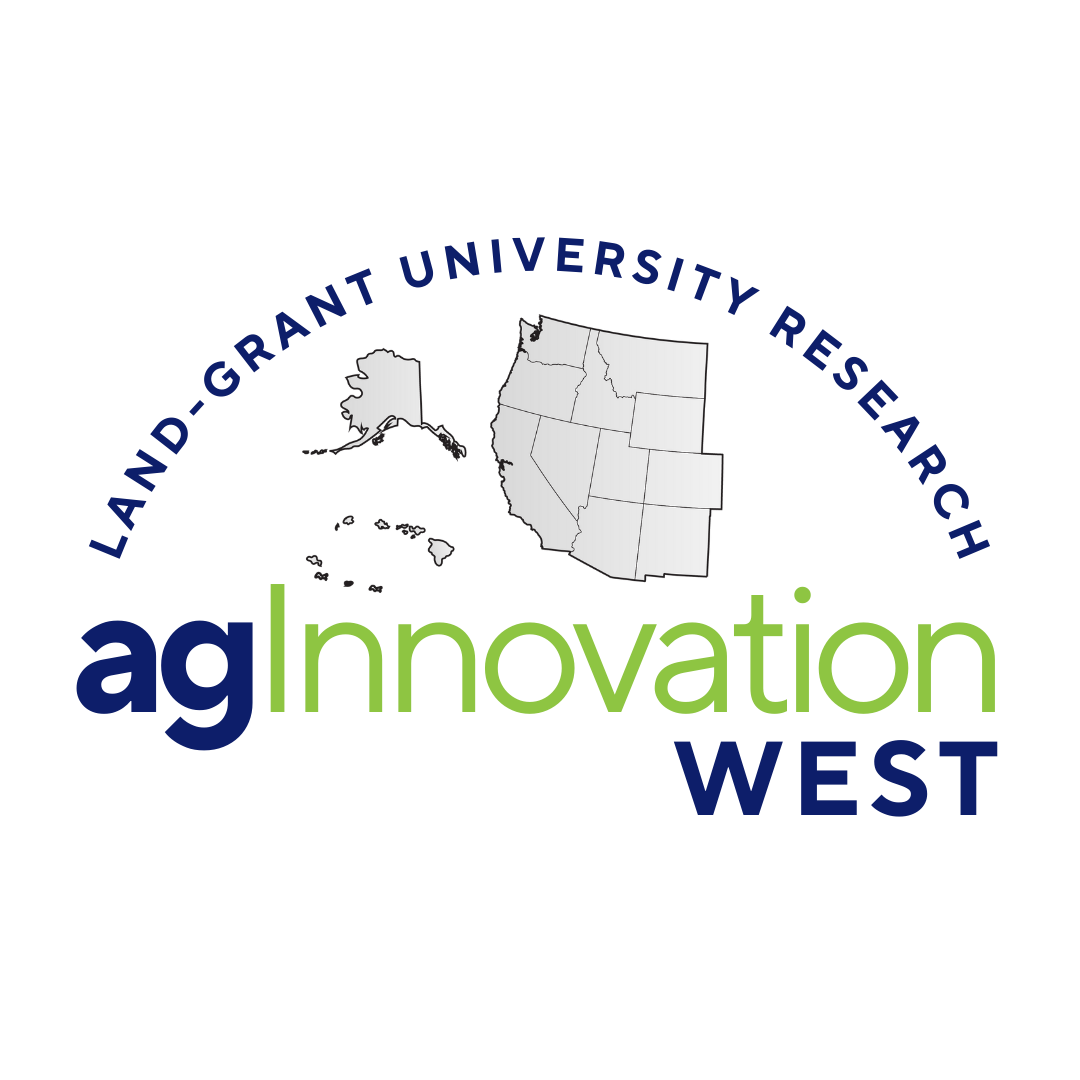
W5188: Soil, Water, and Environmental Physics to Sustain Agriculture and Natural Resources
(Multistate Research Project)
Status: Active
W5188: Soil, Water, and Environmental Physics to Sustain Agriculture and Natural Resources
Duration: 10/01/2024 to 09/30/2029
Administrative Advisor(s):
NIFA Reps:
Non-Technical Summary
Soil physics plays a critical role in our understanding of the functions of soil in regulating water, energy, and gas transport at the Earth’s surface, and progress in recent years has led to an even greater appreciation of the complexity and variability of soils. Historically, the field of soil physics was concerned with agricultural issues such as irrigation scheduling, nutrient management, and improving crop productivity. In recent years, new areas of study have emerged, including emphases on interdisciplinary work related to the impacts and applications of soil physics in ecology, hydrology, geohydrology, biogeochemistry, climate science, and other related fields. This project is one avenue through which these interdisciplinary studies are carried out. The collaborations developed through this multistate project have affected multiple generations of soil physicists and other scientists with significant engagement in soil physics, and the continuation of the project will serve to maintain many of these relationships into the future while simultaneously creating a pathway for new scientists to join.
Statement of Issues and Justification
Soil and the underlying vadose zone (in the remainder of this document, for simplicity, included in the term “soil”) are critical components of the Earth system, maintaining plant and animal life, supporting food production, providing key ecosystem services, and being a critical storage, reaction, and transport medium for water, dissolved solutes, gasses, and pollutants. Soils (i.e., soils and their underlying vadose zone) regulate water, energy, and nutrient movement throughout the terrestrial system, sustaining life above and below ground. As demands for food and water increase, so do the demands placed on soil resources. In order to sustain soils and their key functions into the future, efforts must be made to steward and protect this non-renewable resource.
Soil physics plays a critical role in our understanding of the functions of soil in regulating mass and energy transport at the Earth’s surface, and progress in recent years has led to an even greater appreciation of the complexity and heterogeneity of soils. From micropore to the continental scale, understanding and quantifying soil heterogeneity remains a key challenge. Yet, this heterogeneity is a determining factor in the fate of water, nutrients, and energy with the groundwater-soil-plant-atmosphere continuum. Much research has been done at moderate spatial scales (i.e., a single field or plot), but many questions remain regarding these processes at much finer scales (i.e., nm or smaller) and at larger spatial scales (i.e,. 100s of km or larger). Recent advances in technology have increased our ability to image soil at smaller scales, and increasing computational power allows simulations of water movement at the continental scale; still, much remains to be learned about this complex system.
Historically, the field of soil physics was concerned with issues of agricultural importance such as irrigation scheduling, nutrient management, and improving crop productivity. In recent years, that focus has remained but new foci have emerged, including emphases on interdisciplinary work related to the impacts and applications of soil physics in ecology, hydrology, geohydrology, biogeochemistry, climate science, and other related fields. This trend has been observed in the field at large but also has been seen quite clearly in the outputs from this multistate project, which has grown in the breadth of topics studied over the past decades.
The collaborations developed through this multistate project have affected multiple generations of soil physicists and other scientists with significant engagement in soil physics, and the continuation of the project will serve to maintain many of these relationships into the future while simultaneously creating a pathway for new scientists to join. From the collaborations within this group, the field of soil physics has been transformed by new fundamental knowledge and applications of this knowledge have been developed for broad societal and environmental benefit. Members of this group tend to form and reform around new multi-investigator programs to address emerging critical questions for sustainable solutions to grand challenges. This flexible and synergistic approach has been extremely productive, and it encourages a rich pollination of ideas and solutions to complex problems. The multistate committee structure is a convenient and efficient platform for establishing national research collaborations, validating approaches and techniques, pooling data, creating rigorous peer reviews, sharing equipment and developing the next generation of highly-trained soil scientists, environmental scientists, and engineers. This renewal proposal seeks to maintain the ties between this extremely productive and creative group. Without the W5188 committee, the field would not be as focused on national needs research. The proposal also highlights our efforts to improve environmental monitoring, implement basic soil physics research, reach out to a broader scientific community (e.g., plant science, ecology, chemistry, and microbiology), and educate and communicate to stakeholders and colleagues within and outside our traditional disciplines.
Related, Current and Previous Work
Though other active multistate research projects examine related soil, water quality, and water quantity issues, none of them focus on the interactions and feedbacks between soil physical and hydraulic properties, soil structure, energy and mass balances, soil health, and climate, and between soil/vadose zone processes and groundwater. Relevant multistate projects with some aspects similar to the proposed W5188 activities include:
- NC1034: Impact Analyses and Decision Strategies for Agricultural Research
- NC1178: Land use and management practice impacts on soil carbon and associated agroecosytems services
- NC1186: Water Management and Quality for Specialty Crop Production and Health
- NCAC1: Crop and Soil Research
- NC1195: Enhancing nitrogen utilization in corn based cropping systems to increase yield, improve profitability and minimize environmental impacts
- NC1198: Enhancing the Resilience of Agriculture and Food of the Middle: Building for the Future
- WDC52: Implementing and Correlating Soil Health Management and Assessment in Western States
- WERA1022: Irrigation Technologies and Scheduling for Water Conservation and Water Resources Management
- S1090: AI in Agroecosystems: Big Data and Smart Technology-Driven Sustainable Production
- SERA6: Methodology, Interpretation, and Implementation of Soil, Plant, Byproduct, and Water Analyses
- NCERA3: Soil and Landscape Assessment, Function and Interpretation
- NCERA59: Soil Organic Matter: Formation, Function and Management
- W4147: Managing Plant Microbe Interactions in Soil to Promote Sustainable Agriculture
- W508: Western Water Network for Agriculture and Water Smart Communities: Responding to Climate Change and Other Stressors to Water Resources
The results of the previous W4188 multistate project are extensive, timely, and applicable to numerous agricultural and environmental issues. With the national dialog further expanding to include impacts of climate change, links between population growth in the U.S. and land use change, the need for sustaining the health of soils, and the importance of soil to moderate and control the water budget and important ecological systems, the general themes of W5188 are even more critical. There is consensus that the soil physics community can and should continue to pursue collaborative efforts, so that our thus-far integrated knowledge and skills can be applied to sustainable agricultural and environmental practices, natural resource stewardship, and the adaptation to and mitigation of global climate change.
This project was the 2021 winner of the “Excellence in Multistate Research” award and represents the largest group of soil physics researchers in the U.S. outside of the Soil Science Society of America (SSSA) Soil Physics and Hydrology Division. This group was also recently recognized by the National Academies of Sciences, Engineering, and Medicine as being exemplary in working on issues of national importance to advance knowledge and provide clear economic, environmental, and social benefits.
The objectives of this multistate project are to: 1) Improve fundamental understanding of soil physical and vadose zone processes; 2) Apply soil physical and vadose zone concepts to improve soil and water management; 3) Develop new instrumentation, methodology, and models to characterize and interpret soil physical and vadose zone processes; and 4) Translate new concepts and methods to students, stakeholders, and the public.
Objectives
-
Improve fundamental understanding of soil physical and vadose zone processes
Comments: 1.1. Improve understanding of preferential flow and its role in biogeochemistry 1.2. Study the role of soils in greenhouse gas emissions 1.3. Dynamic changes in soil properties and influence on processes, including water retention, coupled heat and mass transfer processes (e.g., solutes, gasses, water) 1.4. Surface energy balance and evapotranspiration 1.5. Drivers of hydrologic change 1.6. Water, solute, and heat flow in heterogeneous systems 1.7. Deep vadose zone processes and linkages to groundwater 1.8. Behaviors of emerging contaminants in soils **See attachment for Decryption of Objectives** -
Apply soil physical and vadose zone concepts to improve soil and water management
Comments: 2.1. Applications to address soil function and soil resiliency, including climate change mitigation 2.2. Address soil-related challenges within the water-food-energy-climate- environment nexus 2.3. Physics of non-soils growing media for food production on earth and at reduced gravity (on orbit, moon, Mars) 2.4. Applying soil physics to assess or improve soil health 2.5. Soil moisture and other soil sensing networks and their applications 2.6. Proximal and large-scale soil moisture sensing technologies **See attachment for Decryption of Objectives** -
Develop new instrumentation, methodology, and models to characterize and interpret soil physical and vadose zone processes
Comments: 3.1. Sensor development 3.2. Sensor protocols and evaluation/inter-comparison 3.3. Model-data fusion and integration for decision-support systems (including AI and robotics/IOT) 3.4. Development and parameterization of process-based models that simulate soil and vadose zone processes 3.5. Upscaling and downscaling of in situ, proximal, and remote sensing data for parameterization of models in the absence/scarcity of soil geodatabases 3.6. Apply geophysical tools to better quantify subsurface heterogeneity, hydrologically relevant properties, and groundwater and vadose zone interactions 3.7. Integration of sensor data, remote sensing data, in situ measurements across scales into scale-appropriate data analysis, modeling, and decision-support tools **See attachment for Decryption of Objectives** -
4. Translate new concepts and methods to students, stakeholders, and the public
Comments: 4.1. Making our science more actionable for stakeholders and decision makers through knowledge translation, extension, and public outreach 4.2. Open-access and reproducible science 4.3. Open-access educational resources 4.4. Improved pedagogy and teaching methods 4.5. K-12 outreach and education 4.6. Diversity, equity, and inclusion and improving recruitment, retention of students in soil physics, hydrology, and environmental sciences 4.7. Improving interdisciplinary interactions **See attachment for Decryption of Objectives**
Methods
Methods for each sub-objective (states to add their information) Objectives:
- Improve fundamental understanding of soil physical and vadose zone (Fundamental understanding)
1.1. Improve understanding of preferential flow and its role in biogeochemistry
- OR, VA: use isotope tracers in mobile/immobile domains, electrical resistivity tomography and transient electromagnetic method to detect preferential flow
- DE: conduct column experiments to link soil structure with water flow and distribution and with biogeochemical processes (e.g., C dynamics, enzyme activities)
- DE: perform water isotope analysis of field samples from a coastal wetland to improve understanding of vadose processes influenced by tidal events, storms and seawater intrusion.
- TX: detect and predict preferential flow using in situ soil moisture sensors
- CA: quantify impact of preferential flow on soil health and its dynamics under different shade/light treatments
- CA, OR: perform tracer experiments and monitor nitrate, EC and oxyanion concentrations in the vadose zone and compare to vadose zone models that represent preferential flow
- UT, MN, VA, CA, TX: develop novel models/algorithms for describing preferential flow in soil
- MN, NV, CA, VA: improve understanding of infiltration behavior in water- repellent soils, and thereby improve understanding of preferential flow in water-repellent soils.
1.2. Study the role of soils in greenhouse gas emissions
- TX/LA/VA: evaluate GHG emissions under different pasture and row cropping management practices
- MT: measure carbon sequestration potential and greenhouse gas implications of bioenergy grass production
- AL: evaluate the effects of biochar and biopolymers on soil thermal and physical properties
- CA: monitor CO2 and N2O emissions and N cycling from agricultural fields flooded for groundwater recharge
- MN: quantify methane emissions from peatland soils by field measurements and modeling.
1.3. Dynamic changes in soil properties and influence on processes, including water retention, coupled heat and mass transfer processes (e.g., solutes, gasses, water)
- AL, VA: quantify variation of in-situ soil hydraulic properties in space and time under different land uses.
- AL: evaluate the effects of biopolymers on soil hydraulic
- AL: evaluate water retention and hysteresis in two highly weathered soils and poultry litter
- IA/NC: characterize soil structure information from transport properties
- DE: measure effects of flooding and salinity on soil physical and hydraulic properties
- CA: evaluate impact of microclimate and different light/shade treatments on soil dynamics and the changes/evolution of soil properties
- CA: evaluate impact of intentional flooding of agricultural soils for groundwater recharge on physical soil clogging and infiltration rate
- WA, CT: evaluate the effects of emerging pollutants, including micro- and nanoplastics, on soil properties
- NM: evaluate the effect of land fallowing and addition of rock dust as amendments
- OR: dynamics of temperature, water flow, and in situ solute transport during drywell-recharge
- OR: understand change in hydraulic properties, in situ clay mobilization and clogging during various vadose zone MAR.
- KS: evaluate and measure in situ soil water retention curves
1.4. Surface energy balance and evapotranspiration
- CA: compare performance of micrometeorological and isotopic methods for evapotranspiration partitioning
- CA: estimate plant response to different light treatments
- AL: investigate microclimate conditions inside and outside of agroforestry systems
- DE: improve understanding of evaporation and evapotranspiration processes from soil under the influence of salt
- AZ: develop new means for estimation of crop water consumption from remotely sensed SWIR reflectance to conserve agricultural water
- KS: Test new low-cost sensors for measuring field-scale evapotranspiration
1.5. Drivers of hydrologic change
- DE: establish and instrument a long-term monitoring site at the Jones Reserve to observe the changes in soil biogeochemical and hydrological processes under the influence of coastal flooding and seawater intrusion.
- NV: measure fire-impacts on soil structure, and measure sorptivity of sub- critically water-repellent soil in the field.
- AZ: fire-impacts on soil hydraulic properties and biogeochemistry, and time- scale of soil recovery
- CA: Assessing drought impacts on streamflow and groundwater resources across the US
- CA: Assessing the potential impact of managed aquifer recharge on streamflow and groundwater
- MT: analysis of woody plant expansion (WPE) and effects of prescribed fire in the Northern Great Plains
- WA,CT: quantify changes in soil hydrology induced by agricultural plastic mulch films
- KS: measure soil moisture at the watershed level using in situ, proximal and remote sensors to better understand the link between soil moisture and
1.6. Water, solutes, and heat flow in heterogeneous systems
- LA: quantify spatial variability of soil properties and their influences on field- scale soil water dynamics and crop growth.
- VA: identify causes of tree mortality from growing media
- OR: use water and heat flow as tracers for recharge from drywell-
- CA: identify field/orchard-scale water, nitrogen, and salt fluxes in irrigated agriculture, through highly heterogeneous alluvial soil and vadose zone systems into groundwater.
- CA: perform basin-scale assessment of nutrient and salt management practices on nitrate and salt fluxes into groundwater.
1.7. Deep vadose zone processes and linkages to groundwater
- OR: explain complex deep vadose zone hydrology and subsurface heterogeneity on infiltration, recharge, and contaminant transport from drywell-MAR.
- CA: improve mountain system recharge prediction in the Sierra Nevada
- CA: assess the role of spatial variability in subsurface geological and geochemical heterogeneity on groundwater recharge and solute/contaminant
- CA: examine nitrogen and carbon cycling processes and mobilization of heavy metals in the deep vadose zone.
- CA, OR: perform water quality threats assessment of drywells as stormwater drainage and aquifer recharge tools.
- NM: perform transient storage model parameter optimization using the simulated annealing method.
- AZ: build release database of Maricopa Deep Infiltration Site experimental and interpreted data.
- NE: assess spatial and temporal heterogeneity of deep vadose zone denitrification zones and effects on fate and transport of agricultural and industrial contaminants.
- MN: quantify the spatial distribution of chloride in groundwater and the contribution of groundwater seepage of chloride to surface waters.
1.8. Behaviors of emerging contaminants in soils
- MI, VA, NE: track the fate and transport of environmental contaminants in soil, water, and plant systems, including urban and irrigated cropping
- CA: create new contaminant transport modules for the HYDRUS-1D
- CA: use reactive transport models that capture nitrogen cycling
- CA: perform crop modeling to understand climate and hydrologic change impact on nitrogen and carbon cycling and nitrate leaching.
- WA, CT: analyze surface properties of micro- and nanoplastics in terrestrial systems; assess fate and transport of micro- and nanoplastics in soils
- OR: chemical and biological fingerprinting for contaminant source tracking
2. Apply soil physical and vadose zone concepts to improve soil and water management. (Applied science)
2.1. Applications to address soil function and soil resiliency (including climate change mitigation)
- CA: identify impact of regenerative agricultural practices on soil physical parameters and functioning.
- CA: assess impact of managed aquifer recharge on soil water and groundwater balance, soil health and water quality.
- NM: quantify response of SOC and N to different cover crops and mixtures in a limited irrigation winter wheat-sorghum-fallow rotation.
- FL: enhance our understanding of the water dynamics and hydraulic properties of sandy soils as well as their influence on agricultural water and nutrient management and sustainability of surface and groundwater
- TX: enhance estimates of soil physical properties for soil health and groundwater management and prediction.
- NE: develop irrigation management and technologies to improve vadose zone water quality and aquifer protection.
- KY: improve nitrogen and irrigation management relative to landscape
2.2. Address soil-related challenges within the water-food-energy-climate nexus
- TX, KS: use soil moisture information for improving agricultural production and decision making
- NM: measure effects of salinity on food and forage
- TX: evaluate rootzone soil water dynamic under various agronomic practices/conditions in semiarid environments.
- CA: incorporate nitrogen in the water-energy-food
- CA: compare SWAT and HYDRUS modeling approaches to estimate nitrogen leaching from crop rotations with tomatoes under California conditions.
- VA: manage soil water content and infiltration in agricultural systems (e.g., vineyards, row crops).
- NM: optimize planting density and irrigation depth of hybrid maize seed
- NE: evaluate nitrates, salinity, and munition contamination in vadose zones underlying irrigated agricultural fields.
- AZ: have staged-release of gridded high-res (100m) hydraulic properties for the contiguous USA (700+ million points) based on Soil Grids with transition to NRCS-SOLUS-100/30.
- AZ: update and validate ensemble Pedotransfer functions with NRCS-NASIS
- AZ: quantify the potential for enhanced weathering in Arizona agricultural and rangeland systems.
- OR: integrate water harvesting from agrivoltaics with drywell-MAR to mitigate surface runoff, nutrient leaching, and alternative water for
- OR: use alternate water such as waste water for recharge and understand the change in soil properties and contaminant transport.
2.3. Physics of non-soils growing media for food production
- UT: improve plant growth media for “pick and eat” production in reduced gravity conditions.
- VA: quantify hydraulic properties of different soilless substrates to optimize irrigation strategies and rates.
- ID: recommend strategies for tension-based irrigation
- AZ: characterize/engineer optimal soilless substrates for soilless culture applications; simulate flow and transport processes in soilless substrates to optimize container geometry (i.e., prevent dead volumes) and irrigation
- FL: improve physical and hydraulic properties of sandy soils with domestic soil substrates.
2.4. Applying soil physics to assess or improve soil health
- LA, VA: explore effects of cover cropping management on soil water and nutrient stores and fluxes.
- FL: develop data-driven modeling tools for advancing soil health in agriculture, mitigation of climate change impacts, and the security and sustainability of soil and water resources.
- TX: evaluate impacts of soil health practices on soil physical properties, review on hydrologic impacts of soil health practices.
- NM: measure soil health changes due to land fallowing and addition of
- AZ: understand stockpiling of topsoil affects soil health in semiarid mining
2.5. Soil moisture networks and their applications
- CA: measure water and nitrogen fluxes in agricultural fields; perform vadose zone monitoring (soil water tension, soil water content, soil water solution).
- WY: maintain a soil moisture and rainfall monitoring network in Wyoming rangelands and evaluate drought conditions.
- KS: maintain hydrological monitoring network at the Konza Prairie to study the connection between rootzone soil moisture and streamflow in tallgrass prairies; determine optimal in situ soil moisture monitoring depths.
- AZ: perform long-term modeling of soil moisture dynamics at NRCS SCAN sites using high-resolution soil hydraulic properties.
- OK: evaluate and improve soil moisture prediction algorithms for use in dynamic soil surveys.
- OK: develop applications of soil climate measurements and soil moisture predictions in forecasting streamflow and water table depth.
- OR: develop deep vadose zone sensor based monitoring for recharge estimation and contaminant transport.
2.6. Proximal and large-scale soil moisture sensing technologies
- KS, OK, TX: apply cosmic ray neutron sensors for proximal soil moisture
- AZ: estimate farm scale root zone soil moisture from remotely sensed
- AZ: interpret SMAP data with high-resolution gridded hydraulic
- FL: perform high-resolution profile soil moisture mapping with microwave proximal and remote sensors and AI techniques.
3. Develop new instrumentation, methodology, and models to characterize and interpret soil physical and vadose zone processes. (Methodology)
3.1. Sensor development
- DE: explore the potential of using VIS-NIR soil spectral measurement to develop a rapid tool for determining soil salinization for both saltine and non- saline soil.
- IA, NC: evaluate thermo-TDR sensors, impacts of salinity on
- UT: develop new electromagnetic sensing and measurement methods in
- WI: develop in situ multi-functional soil moisture, nitrate, and temperature
- VA: perform field tests of low-cost systems to measure near-surface greenhouse gas emissions.
3.2. Sensor protocols and evaluation/inter-comparison
- KS, TX, OK: install in situ soil moisture sensor
- TX: utilize acquired waveforms from the new Acclima TDR-315N sensor for the characterization of soil properties and to improve water content calibrations specific to a given soil.
- OR: develop a deep vadose zone monitoring
- UT, KS, TX: develop standards for electromagnetic-based sensor calibration and evaluation
3.3. Model-data fusion and integration for decision-support systems (including AI and robotics/IOT)
- KS: continue work on prototyping a deep neural network to quantify bare soil, green canopy cover, and crop residue using digital images.
- TX: perform field monitoring under different land use land covers for improved understanding of soil moisture, temperature, and carbon dynamics; develop new soil hydraulic response units using various satellite
- AZ: develop short- and mid-term forecasts of actual evapotranspiration with deep learning.
- AZ: develop a novel physical-empirical model linking shortwave infrared reflectance and soil water retention.
- WI: integrate in situ soil moisture sensors and remote sensing data using machine learning and data assimilation for mapping soil moisture at high spatial (100-m) and temporal (daily) resolutions.
- CA: develop a modeling framework for plant response to different light spectra under agrivoltaics systems.
- TX: fuse data from satellite and in situ platforms to assess surface moisture spatiotemporal distributions, dry down patterns, and associated hydrologic fluxes (ET and baseflow) estimation.
- FL: integrate physical and data driven models for characterizing soil hydraulic properties and water flow.
- CA: characterize hydrologic flow paths in mountainous areas using geochemical data and mixing models.
3.4. Development and parameterization of process-based models that simulate soil and vadose zone processes
- VA: develop new theoretical and experimental framework to analyze gas diffusivity in soils and soilless substrates with non-uniform water
- CA: continue HYDRUS model
- CA: improve vegetation parameterization in integrated groundwater-land surface models.
- KY: evaluate soil hydraulic property parameters within the Root Zone Water Quality Model (RZWQM2); assess spatial variability of soil physical properties and modeling of spatial soil hydrologic processes at different scales; parameterize and adapt multidimensional watershed model for decision support in water and nitrogen management.
- NE: identify the frequency and occurrence of funnel flows and denitrification hotspots in deep vadose zones.
- UT: develop new soil water flow equations using machine learning that go beyond Richardson-Richards Equation.
- WY: continue refinement of a numerical 1-D vertical coupled water-heat- solute flow and transport model for soils in cold regions.
3.5. Upscaling and downscaling of in situ, proximal, and remote sensing data for parameterization of models in the absence/scarcity of soil geodatabases.
- FL: integrate SMAP and SOLUS digital maps for real-time and high- resolution soil moisture mapping.
- KY: analyze crop yield, remotely sensed vegetation indices, topographic information and soil textural information at different resolutions to quantify the change of information of space-time relationships, and identify scales that effectively contribute to the improvement of management.
3.6. Apply geophysical tools to better quantify subsurface heterogeneity, hydrologically relevant properties, and groundwater and vadose zone interactions
- WY: evaluate different methods to predict subsurface hydraulic parameters using electrical resistivity tomography and seismic refraction data.
- NM: perform noninvasive geophysical and sensor methods for hyporheic zone
- NE, OR: characterize subsurface properties and heterogeneity using methods such as ERT, TEM, NMR, GPR, boreholes, and Nebraska GeoCloud.
- ID: integrate ERT and EMI measurements in irrigation design
3.7. Integration of sensor data, remote sensing data, in situ measurements across scales into scale-appropriate data analysis, modeling, and decision-support tools
- CA: compare land surface-based (mass balance) monitoring of water and nitrogen fluxes, (plot-scale, spatially repeated) vadose zone monitoring of water content, soil water tension, and nitrogen concentrations, and (large plot- scale, spatially repeated) shallow groundwater monitoring of water levels and nitrate concentrations.
- CA: compare modeling approaches for assessing spatially distributed (resolution: field scale/hydrologic response unit) basin-scale nitrate and salinity transport in recharge to groundwater: mass balance, HYDRUS,
- KY: perform co-regionalization of soil measurements, soil and crop sensor data and remote sensing and their integration with landscape topography to parameterize 1-D (RZWQM2) and 3-D (SWAT) crop growth and soil process models for decision support.
4. Translate new concepts and methods to students, stakeholders, and the public. (Outreach, Extension, and Education)
4.1. Making our science more actionable for stakeholders and decision makers through knowledge translation, extension, and public outreach
- OR: develop and implement an action plan to reduce the nitrate concentration in groundwater less than 7 mg/L and repeal the GWMA status of the Lower Umatilla basin.
- CA: establish and implement a novel framework for the role of scientist communication in policy making.
- CA: perform an economic analysis of grower behavior under various groundwater salinization scenarios.
- CA: develop and implement groundwater sustainability plans for California groundwater basins.
- CA: develop and implement water quality guidance and decision-support tools for managers of agricultural or other managed aquifer recharge operations.
- ID: build out the Western Water
- KY: Hold short courses for farmers, extension agents, and consultants to analyze field-scale data of yield maps, drone and satellite remote sensing and topographic elevation and convert them into management decisions.
- Perform field days (many locations)
4.2. Open-access and reproducible science (e.g., develop open data APIs, standardize data formats and protocols to integrate outputs across networks and test new datasets like Open ET)
- CA: develop a comprehensive framework and implement case study for measuring stream depletion of surface water due to groundwater
- AZ: continue annual releases of NRCS-SOLUS-based estimates of gridded soil hydraulic property Geotiff data, workflow annotations, and underlying Python/R code.
- CA: release source codes for web apps and web resources for diagnosis and improvement of saline and sodic soils.
4.3. Open-access educational resources
- OK: release open-source textbook “Rain or Shine”.
- CA: release web apps and web resources for diagnosis and improvement of saline and sodic soils
4.4. Improved pedagogy (teaching) methods (e.g., hands-on experiences like lab and field sessions)
- CA: hold HYDRUS short
4.5. K-12 outreach and education
- TX: perform K-12 teacher trainings in St. Louis and Puerto Rico.
- VA: lead demonstration days on soil health with K-5 students
- WI: hold presentations at the Wisconsin Science Festivals and Ag Discovery Day to increase the public awareness of soil.
4.6. DEI and improving recruitment, retention of students in soil physics, hydrology, and environmental sciences
- TX: serve on ASA-CSSA-SSSA DEI committee, AGU Hydrology JEDI committee, SSSA K-12 committee.
Measurement of Progress and Results
Outputs
- New methods and approaches to study mass and energy transport processes in soils at spatial and temporal scales appropriate for effective resource management. Comments: The research described herein will create outputs (which we define as activities, services, methods, approaches) that will significantly improve the science and applications of mass and energy transport in near-surface environments. These outputs include:
- New knowledge affecting the environmental impacts of soil, water and chemically-based agricultural practices and broader land uses.
- Methods to transfer results from non-destructive imaging into quantitative assessments of soil structure.
- New instruments and analytical techniques for measuring water, chemical, and energy fluxes.
- New tools and capabilities to quantify and monitor movement of agricultural contaminants from the vadose zone to ground water and to the atmosphere.
- New methodologies (computer and analytical models) that integrate knowledge of mass and energy transport, improving resource management.
- New statistical methods to link crop yield and variability from sensor measurements.
- Updated versions of various numerical tools being developed: HYDRUS, HP1, CW2D module, the UNSATCHEM modules, the HYDRUS package for MODFLOW, SMART, and others.
- Machine learning methods and tools to interpret large datasets from field studies and monitoring sites.
- Improved methods for uncertainty quantification
- Improved methods for data assimilation
- Continued support of young faculty, postdocs and students, who are dedicated to studying the role of soil physics in environmental processes.
- New graduate level, international course on soils in the global groundwater-agriculture interface.
Outcomes or Projected Impacts
- New scientific knowledge and information about fundamental physical, chemical and biological processes will help to understand the transformation and transport of pesticides, pathogens, colloids, nutrients, salts, and emerging contaminants. The breadth of research topical areas will lead to a diverse set of outcomes (which we define as results, impacts, and accomplishments) that will significantly improve our knowledge regarding mass and energy transport in near-surface environments. These outcomes will help us determine how to enhance soil sustainability and benefit society as follows:
- Improved understanding of the role of scale in basin-scale processes, including evapotranspiration, water balance and ecological functions and services.
- Improved understanding of processes that control behavior of emerging contaminants from gray water or treated wastewater in soil/water systems, including mitigation practices.
- Guidance to producers on the sustainability of drip irrigation in salt-affected soils with reduced quantity or marginal quality irrigation water will be improved.
- Broader use of frequency-dependent dielectric measurements in soil to infer soil textural properties, in addition to water content and electrical conductivity.
- Improved measurement techniques will better characterize the relationships between soil, climate, and geomorphic position at the landscape scale.
- Improvements in the Evaluation and prediction of land-use changes on managed lands (impact of grazing on compaction, erodibility, plant communities).
- Landscape-scale predictive capabilities for soil evaporation implemented into large-scale climate models.
- Improved protection of soil and water resources from energy production (i.e., coal and mineral extraction, in particular mine tailings).
- Solution to the closure problem for various hydrologic fluxes, including heterogeneity adopted into lumped parameter models (i.e., SWAT).
- Assessments, briefings, and legislative testimony in direct support of policy and decision- making bodies at the state and federal level.
Milestones
(2024):● First open data release of gridded soil hydraulic properties based on NRCS-SOLUS, including manuscript. Release of Maricopa Deep Infiltration data (open access) ● Determine surface properties and colloidal stabilities of different types of micro- and nanoplastics. ● Complete simulations of soil moisture dynamics at in situ monitoring stations nationwide using SOILWAT2 and TOPOFIRE. ● Complete development for methodology to downscale GRACE satellite total water storage anomaly to HUC-12 catchment scale. ● Publish the results from laboratory experiments aimed at quantifying gas diffusion rates and pore size distributions of nursery substrates. ● Complete development of the theoretical framework and initial results for quantifying hydraulic properties of porous media using tension infiltration. ● Quantification of changes in dynamic soil physical properties at various times throughout the growing season, and relationship to soil properties in undisturbed locations ● Development of remote-sensing based approach for detecting soil change based on land use type/change ● Quantify inter- and intra-sensor variability using in situ soil moisture sensor testbeds ● Complete K-12 teacher trainings on soil science topics in Puerto Rico at SSSA Summer Meeting(2025):● Second open data release of gridded soil hydraulic properties based on NRCS-SOLUS, including manuscript. Release of NRCS-NASIS-validated ensemble pedotransfer functions. ● Proof-of-concept decadal simulations of soil moisture dynamics as NRCS-SCAN locations. ● Quantification of transport characteristics of micro- and nanoplastics under both saturated and unsaturated flow conditions in porous media. ● Improve Oklahoma Automated Soil Information System (OASIS) and evaluate potential for nationwide expansion. ● Build and test a new system for monitoring soil particle movement during erosion. ● Test system for distributed measurements of CO2 fluxes using low-cost sensors. ● Application of field-scale soil moisture data for predicting soil cracking
(2026):Provisional third open data release of gridded soil hydraulic properties based on NRCS- SOLUS, including manuscript. Updated decadal simulations of soil moisture dynamics as NRCS-SCAN locations. ● Determination of colloidal stability of micro- and nanoplastics in soil and aqueous environments. ● Continue the development of modeling frameworks to understand soil water repellency and preferential flow processes in soils ● Complete development of preferential flow capable Green-Ampt type infiltration model. ● Complete watershed-scale cosmic-ray neutron rover surveys of soil moisture spatial distributions in four watersheds. ● Provide recommendations for vineyard soil management that enhances wine-grape quality.
(2027):● Provisional simulations of decadal soil moisture dynamic at selected points within contiguous USA, consistent with SMAP. ● Determination of how the use of biodegradable plastic mulch films affect soil health. ● Use improved soil moisture datasets for streamflow forecasting and prediction of changes in groundwater levels. ● Complete development of peatland soil flow and transport model for simulation of methane and mercury export out of peatland soils.
(2028):● Prepare and submit new proposal for 5-year project renewal. ● Complete mapping of concentration and residence time of chloride in groundwater of the Twin Cities Metro area.
Projected Participation
View Appendix E: ParticipationOutreach Plan
The project members comprise a group of dedicated soil, water and environmental scientists and engineers who excel in the communication of their research through different communications platforms, and who are active participants in soil and environmental research at universities and federal facilities across the country. For this multi-state project, we have developed a new objective (Objective 4) that specifically details many of the focal areas and activities that the project members will undertake as part of this project. Many of our members conduct workshops, short courses, and classes to educate other scientists and the public, and contribute to state, regional and federal agencies. They also lead undergraduate and graduate education and supervise research.Although most of our members do not have formal extension appointments, our members regularly participate in field days organized by extension faculty at the land grant universities. Our members are involved in publishing extension pamphlets, articles, and videos on their research projects. We are also involved in providing inputs to federal regulations pertinent to our research activities. For instance, our work on the environmental fate of microplastics helps the National Organic Standards Board to determine how to regulate the use of plastics in organic agriculture. Members of our group are actively involved in these discussions. As another instance, results of hydrologic and water quality modeling in agricultural production settings in southeastern Minnesota is being used to guide placement of nitrogen management BMPs intended to reduce nitrate transport to groundwater and surface water in the region.
W4188 members have published their findings in top-tier, peer-reviewed journals, targeting both science and engineering communities and are actively involved in organizing and participating many professional society international/national/regional meetings (SSSA, AGU, ESA, EGU, ASABE, ASCE, GRA, GSA), and major workshops and symposia sponsored by these societies. They serve as Editors and Associate Editors on journal editorial boards and as ad hoc manuscript reviewers, and therefore, enhance the overall quality of published research. Members also serve the scientific community by their engagement in competitive grant review panels of federal and regional entities, and as peer reviewers for domestic and international grant proposals. Our members have been instrumental in creating the International Soil Modeling Consortium, now with more than 600 members worldwide. They frequently engage with legislators at the state and federal level, and with managers, directors, and personnel in local, regional, state, and national water management organizations (e.g., irrigation and water districts, state agencies, regional MOUs) to support scientifically-based policy development and assist in technically sound decision-making. Through entrepreneurship, committee members have developed commercially available instruments, analytical tools, and textbooks. We fully expect this type of outreach to continue and thrive. Results of our work will be available through the annual project report, the project website (https://www.nimss.org/projects/18606), periodic joint meetings with related multistate research and/or coordinating groups, and through the international reputations and professional visibility of participants. The members will also work with consulting firms, companies and farmers to adopt measurement and management technologies.
Organization/Governance
The current W4188 multistate committee consists of members representing universities, the USDA-ARS, National Laboratories, and other research units. In addition, visiting scientists (U.S. and global) participate along with member hosts. Officers of the new W5188 will be the Chair and Secretary. The Secretary is elected each year at the annual meeting and advances to Chair the following year. The Chair may appoint members to serve on subcommittees as needed.
Meetings will be approved by the Administrative Advisor. The current Secretary will be responsible for making local arrangements. Committee meetings typically have been held in Las Vegas, NV during early January, but members may decide (by voting) to choose new locations. Virtual and hybrid options have been offered since 2020. At each meeting, research accomplishments are reviewed, new opportunities and recommendations for multistate coordination/collaboration are discussed, and strategies for maximizing the impact of committee productivity are suggested. In addition, we invite scientists from different disciplines (e.g., geomorphology, land use planning, ecology) to provide opportunities for initiating transdisciplinary collaboration on new cutting-edge research directions that would engage areas of our expertise. In this way, fresh perspectives are injected into the committee, encouraging outward-looking and multi- disciplinary approaches toward pressing agricultural and environmental problems. The project committee and its precursors have had strong historical participation at the annual meetings (35-50 attendees, 59 in 2023), with new members inducted each year to ensure longevity and infusion of fresh perspectives. Although meeting attendance can vary from year to year, existing W4188 members have indicated a strong desire to continue participation.
Literature Cited
Abatzoglou, J.T. and Williams, A.P., 2016. Impact of anthropogenic climate change on wildfire across western US forests. Proceedings of the National Academy of Sciences, 113(42), pp.11770-11775.
Abbaszadeh, P., Moradkhani, H., & Zhan, X. (2019). Downscaling SMAP radiometer soil moisture over the CONUS using an ensemble learning method. Water Resources Research, 55(1), 324-344.
Alaoui, A., Germann, P., Jarvis, N., & Acutis, M. (2003). Dual-porosity and kinematic wave approaches to assess the degree of preferential flow in an unsaturated soil. Hydrological Sciences Journal, 48(3), 455-472.
Alaoui A. Lipiec J. Gerke H.H.. 2011. A review of the changes in the soil pore system due to soil deformation: A hydrodynamic perspective. Soil Tillage Res. 115–116:1–15. doi:10.1016/j.still.2011.06.002
Alizamir, M., Kim, S., Zounemat-Kermani, M., Heddam, S., Kim, N. W., & Singh, V. P. (2020).
Kernel extreme learning machine: an efficient model for estimating daily dew point temperature using weather data. Water, 12(9), 2600.
Allen, D.E., Singh, B.P., & Dalal, R.C. (2011). Soil health indicators under climate change: A review of current knowledge. In: B.P. Singh, A.L. Cowie, K.Y. Chan (Eds.), Soil Health and Climate Change. Springer Berlin Heidelberg, Berlin, Heidelberg, pp. 25-45.
Andreasen, M., Jensen, K. H., Desilets, D., Franz, T. E., Zreda, M., Bogena, H. R., & Looms, M. C. (2017). Status and perspectives on the cosmic‐ray neutron method for soil moisture estimation and other environmental science applications. Vadose Zone Journal, 16(8), 1-11. https://doi.org/10.2136/vzj2017.04.0086
Angers, D.A., Recous, S., & Aita, C. (1997). Fate of carbon and nitrogen in water-stable aggregates during decomposition of 13C15N-labelled wheat straw in situ. European Journal of Soil Science, 48(2), 295-300.
Azarbad, H., Tremblay, J., Giard-Laliberté, C., Bainard, L. D., & Yergeau, E. (2020). Four decades of soil water stress history together with host genotype constrain the response of the wheat microbiome to soil moisture. FEMS microbiology ecology, 96(7), fiaa098.
Bagnall, D. K., Morgan, C. L., Cope, M., Bean, G. M., Cappellazzi, S., Greub, K., ... & Honeycutt, C.W. (2022). Carbon‐sensitive pedotransfer functions for plant available water. Soil Science Society of America Journal, 86(3), 612-629.
Ball, B. C. (2013). Soil structure and greenhouse gas emissions: a synthesis of 20 years of experimentation. European Journal of Soil Science, 64(3), 357-373.
Basset, C., Abou Najm, M., Ghezzehei, T., Hao, X., & Daccache, A. (2023). How does soil structure affect water infiltration? A meta-data systematic review. Soil and Tillage Research, 226, 105577.
Baveye, P. C., Baveye, J., & Gowdy, J. (2016). Soil “ecosystem” services and natural capital: critical appraisal of research on uncertain ground. Frontiers in Environmental Science, 4, 41.
Benard, P., Schepers, J. R., Crosta, M., Zarebanadkouki, M., & Carminati, A. (2021). Physics of viscous bridges in soil biological hotspots. Water Resources Research, 57(11), e2021WR030052.
Benard, P., Zarebanadkouki, M., Brax, M., Kaltenbach, R., Jerjen, I., Marone, F., ... & Carminati, A. (2019). Microhydrological niches in soils: How mucilage and EPS alter the biophysical properties of the rhizosphere and other biological hotspots. Vadose Zone Journal, 18(1), 1- 10.
Berhe, AA, Ghezzehei, TA. Race and racism in soil science. Eur J Soil Sci. 2021; 72: 1292– 1297. https://doi.org/10.1111/ejss.13078
Bernhardt, E. S., Blaszczak, J. R., Ficken, C. D., Fork, M. L., Kaiser, K. E., & Seybold, E. C. (2017). Control points in ecosystems: moving beyond the hot spot hot moment concept. Ecosystems, 20, 665-682.
Beven, K. (2018). A century of denial: Preferential and nonequilibrium water flow in soils, 1864‐ 1984. Vadose Zone Journal, 17(1), 1-17.
Bingham, G. E., Jones, S. B., Or, D., Podolski, I. G., Levinskikh, M. A., Sytchov, V. N., ... & Jahns, G. (2000). Microgravity effects on water supply and substrate properties in porous matrix root support systems. Acta Astronautica, 47(11), 839-848.
Blanco-Canqui, H., & Ruis, S.J. (2020). Cover crop impacts on soil physical properties: A review. Soil Science Society of America Journal, 84(5), 1527-1576.
Blaud, A., Lerch, T.Z., Chevallier, T., Nunan, N., Chenu, C., & Brauman, A. (2012). Dynamics of bacterial communities in relation to soil aggregate formation during the decomposition of 13C-labelled rice straw. Applied Soil Ecology, 53, 1-9.
Bond-Lamberty, B., Smith, A. P., & Bailey, V. (2016). Temperature and moisture effects on greenhouse gas emissions from deep active-layer boreal soils. Biogeosciences, 13(24), 6669- 6681.
Bordoloi, R., Das, B., Yam, G., Pandey, P. K., & Tripathi, O. P. (2019). Modeling of water holding capacity using readily available soil characteristics. Agricultural Research, 8, 347-355.
Bronick, C.J., & Lal, R. (2005). Soil structure and management: A review. Geoderma, 124(1), 3-22.
Brown, W. G., Cosh, M. H., Dong, J., & Ochsner, T. E. (2023). Upscaling soil moisture from point scale to field scale: Toward a general model. Vadose Zone Journal, 22(2), e20244. https://doi.org/10.1002/vzj2.20244
Bundt, M., Widmer, F., Pesaro, M., Zeyer, J., & Blaser, P. (2001). Preferential flow paths: biological ‘hot spots’ in soils. Soil Biology and Biochemistry, 33(6), 729-738.
Bünemann, E.K., Bongiorno, G., Bai, Z., Creamer, R.E., De Deyn, G., de Goede, R., Fleskens, L., Geissen, V., Kuyper, T.W., Mäder, P., Pulleman, M., Sukkel, W., van Groenigen, J.W., & Brussaard, L. (2018). Soil quality-A critical review. Soil Biology and Biochemistry, 120, 105-125.
Burri, K., Graf, F., & Böll, A. (2009). Revegetation measures improve soil aggregate stability: A case study of a landslide area in Central Switzerland. Forest Snow and Landscape Research, 82(1), 45-60.
Butters,G.L., Jury, W.A., Field scale transport of bromide in an unsaturated soil 2. Dispersion modeling, Water Resour. Res., 25 (7) (1989), pp. 1583-1589.
Camporese, M., Cassiani, G., Deiana, R., & Salandin, P. (2011). Assessment of local hydraulic properties from electrical resistivity tomography monitoring of a three‐dimensional synthetic tracer test experiment. Water Resources Research, 47(12).
Carminati, A., & Javaux, M. (2020). Soil rather than xylem vulnerability controls stomatal response to drought. Trends in Plant Science, 25(9), 868-880.
Casa, R., Castaldi, F., Pascucci, S., Basso, B., & Pignatti, S. (2013). Geophysical and hyperspectral data fusion techniques for in-field estimation of soil properties. Vadose Zone Journal, 12(4). Challinor, A., Watson, J., Lobell, D. et al. A meta-analysis of crop yield under climate change and adaptation. Nature Clim Change 4, 287–291 (2014). https://doi.org/10.1038/nclimate2153
Chen, F., and J. Dudhia (2001), Coupling an advanced land surface-hydrology model with the Penn State-NCAR MM5 modeling system. Part I: Model implementation and sensitivity, Mon. Weather Rev., doi:10.1175/1520-0493(2001)1292.0.CO
Clark, L.J., Whalley, W.R., & Barraclough, P.B. (2003). How do roots penetrate strong soil? In: J. Abe (Ed.), Roots: The Dynamic Interface between Plants and the Earth: The 6th Symposium of the International Society of Root Research, 11–15 November 2001, Nagoya, Japan. Springer Netherlands, Dordrecht, pp. 93-104.
Cockett, R., Heagy, L. J. & Haber, E. 2018. Efficient 3D inversions using the Richards equation.
Computers and Geosciences, 116, 91–102
Cosh, M. H., Caldwell, T. G., Baker, C. B., Bolten, J. D., Edwards, N., Goble, P., ... & Woloszyn, E. (2021). Developing a strategy for the national coordinated soil moisture monitoring network. Vadose Zone Journal, 20(4), e20139.
Cosh, M. H., Ochsner, T. E., McKee, L., Dong, J., Basara, J. B., Evett, S. R., ... & Sayde, C. (2016). The soil moisture active passive marena, Oklahoma, in situ sensor testbed (SMAP-MOISST): Testbed design and evaluation of in situ sensors. Vadose Zone Journal, 15(4), vzj2015-09. https://doi.org/10.2136/vzj2015.09.0122
Coyne, M.S., Pena-Yewtukhiw, E.M., Grove, J.H., Sant'Anna, A.C., & Mata-Padrino, D. (2022).
Soil health-It's not all biology. Soil Security, 6, 100051.
Crow, W. T., Berg, A. A., Cosh, M. H., Loew, A., Mohanty, B. P., Panciera, R., ... & Walker, J. P. (2012). Upscaling sparse ground‐based soil moisture observations for the validation of coarse‐resolution satellite soil moisture products. Reviews of Geophysics, 50(2). https://doi.org/10.1029/2011RG000372
Dagan, G., E. Bresler Unsaturated flow in spatially variable fields I. Derivation of models of infiltration and redistribution, Water Resour. Res., 19 (2) (1983), pp. 413-420
Davidson, Z. E., & Palermo, C. (2015). Developing research competence in undergraduate students through hands on learning. Journal of Biomedical Education, 2015.
DeBano, L.F., 2000. The role of fire and soil heating on water repellency in wildland environments: a review. Journal of hydrology, 231, pp.195-206.
De Carlo, L., Vivaldi, G. A., & Caputo, M. C. (2021). Electromagnetic induction measurements for investigating soil salinization caused by saline reclaimed water. Atmosphere, 13(1), 73.
de Dios Benavides-Solorio, J. and MacDonald, L.H., 2005. Measurement and prediction of post-fire erosion at the hillslope scale, Colorado Front Range. International Journal of Wildland Fire, 14(4), pp.457-474.
Dekker, L.W. and Ritsema, C.J., 1994. How water moves in a water repellent sandy soil: 1. Potential and actual water repellency. Water Resources Research, 30(9), pp.2507-2517.
Della Vecchia, G., Dieudonné, A. C., Jommi, C., & Charlier, R. (2015). Accounting for evolving pore size distribution in water retention models for compacted clays. International Journal for Numerical and Analytical Methods in Geomechanics, 39(7), 702-723.
DeCarlo, K. F., & Caylor, K. K. (2019). Biophysical effects on soil crack morphology in a faunally active dryland vertisol. Geoderma, 334, 134-145.
Di Paola A, Caporaso L, Di Paola F, Bombelli A, Vasenev I, Nesterova OV, Castaldi S, Valentini R. The expansion of wheat thermal suitability of Russia in response to climate change. Land Use Policy. 2018 Nov 1;78:70-7.
Dogrul, E. C., & Kadir, T. N. (2012). Integrated Water Flow Model (IWFM v3. 02)– Theoretical documentation. Sacramento, CA: California Department of Water Resources. Dong, Y., McCartney, J. S., & Lu, N. (2015). Critical review of thermal conductivity models for unsaturated soils. Geotechnical and Geological Engineering, 33, 207-221.
Donovan, V.M., Wonkka, C.L. and Twidwell, D., 2017. Surging wildfire activity in a grassland biome. Geophysical Research Letters, 44(12), pp.5986-5993.
Du, Y., Guo, S., Wang, R., Song, X., & Ju, X. (2023). Soil pore structure mediates the effects of soil oxygen on the dynamics of greenhouse gases during wetting–drying phases. Science of The Total Environment, 895, 165192.
Ebrahimi, A., & D. Or. (2018). On upscaling of soil microbial processes and biogeochemical fluxes from aggregates to landscapes. Journal of Geophysical Research: Biogeosciences. doi:10.1029/2017jg004347
Ellsworth,T.R., Jury, W.A. (1991). A three-dimensional field study of solute transport through unsaturated, layered, porous media: 2. Characterization of vertical dispersion, Water Resour. Res., 27 (5), 967-981.
Entekhabi, D., Njoku, E. G., O'neill, P. E., Kellogg, K. H., Crow, W. T., Edelstein, W. N., ... & Van Zyl, J. (2010). The soil moisture active passive (SMAP) mission. Proceedings of the IEEE, 98(5), 704-716.
Fan, Y., Wang, X., Funk, T., Rashid, I., Herman, B., Bompoti, N., ... & Li, B. (2022). A critical review for real-time continuous soil monitoring: Advantages, challenges, and perspectives. Environmental Science & Technology, 56(19), 13546-13564.
Fang, B., Lakshmi, V., Bindlish, R., & Jackson, T. J. (2018). Downscaling of SMAP soil moisture using land surface temperature and vegetation data. Vadose Zone Journal, 17(1), 1-15. https://doi.org/10.2136/vzj2017.11.0198
Fatichi, S., D. Or, R. Walko, H. Vereecken, M. H. Young, T. A. Ghezzehei, . . . R. Avissar. Univ (2020). Soil structure is an important omission in Earth System Models. Nature communications, 11(1), 1-11.
Feyen, D. Jacques, A. Timmerman, J. Vanderborght. Modelling water flow and solute transport in heterogeneous soils: a review of recent approaches J. Agric. Eng. Res., 70 (3) (1998), pp. 231-256
Fields, J.S., J.S. Jr., Owen, R.D. Stewart, J.L. Heitman, and J. Caron. 2020. Modeling water fluxes through containerized soilless substrates using HYDRUS. Vadose Zone J. 19:e20031. doi: 10.1002/vzj2.20031.
Filipović, V., Coquet, Y. and Gerke, H.H., 2019. Representation of plot‐scale soil heterogeneity in dual‐domain effective flow and transport models with mass exchange. Vadose Zone Journal, 18(1), pp.1-14.
Fisher, J.B., Melton, F., Middleton, E., Hain, C., Anderson, M., Allen, R., McCabe, M.F., Hook, S., Baldocchi, D., Townsend, P.A. and Kilic, A., 2017. The future of evapotranspiration: Global requirements for ecosystem functioning, carbon and climate feedbacks, agricultural management, and water resources. Water resources research, 53(4), pp.2618-2626.
Foken, T. (2008), The energy balance closure problem: An overview, Ecol. Appl., 18(6), 1351– 1367, doi:10.1890/06-0922.1.
Franklin, S., Vasilas, B., & Jin, Y. (2019). More than meets the dye: Evaluating preferential flow paths as microbial hotspots. Vadose Zone Journal, 18(1), 1-8.
Franklin, S.M., Kravchenko, A.N., Vargas, R., Vasilas, B., Fuhrmann, J.J., & Jin, Y. (2021). The unexplored role of preferential flow in soil carbon dynamics. Soil Biology and Biochemistry, 161, 108398.
Franzluebbers, A.J. (2002). Water infiltration and soil structure related to organic matter and its stratification with depth. Soil and Tillage Research, 66(2), 197-205.
Fuhrmann, I., Maarastawi, S., Neumann, J., Amelung, W., Frindte, K., Knief, C., ... & Siemens, J. (2019). Preferential flow pathways in paddy rice soils as hot spots for nutrient cycling. Geoderma, 337, 594-606.
Gao, Z., Russell, E.S., Missik, J.E., Huang, M., Chen, X., Strickland, C.E., Clayton, R., Arntzen, E., Ma, Y. and Liu, H., 2017. A novel approach to evaluate soil heat flux calculation: An analytical review of nine methods. Journal of Geophysical Research: Atmospheres, 122(13), pp.6934-6949.
Geisen, S., Wall, D. H., & van der Putten, W. H. (2019). Challenges and opportunities for soil biodiversity in the anthropocene. Current Biology, 29(19), R1036-R1044.
Gerke, H. H., & Van Genuchten, M. T. (1993). A dual‐porosity model for simulating the preferential movement of water and solutes in structured porous media. Water resources research, 29(2), 305-319.
Gerke, H.H., and M.Th . van Genuchten. 1993b. Evaluation of a fi rst-order water transfer term for variably saturated dual-porosity fl ow models. Water Resour. Res. 29:1225–1238.
Germann, P. F., & Karlen, M. (2016). Viscous-flow approach to in situ infiltration and in vitro saturated hydraulic conductivity determination. Vadose zone journal, 15(2), vzj2015-05. Ghorbani, A., Sadeghi, M. and Jones, S.B., 2021. Towards new soil water flow equations using physics‐constrained machine learning. Vadose Zone Journal, 20(4), p.e20136. Gregory, P. J., & Marshall, B. (2012). Attribution of climate change: a methodology to estimate the potential contribution to increases in potato yield in Scotland since 1960. Global Change Biology, 18(4), 1372-1388.
Guevara, M., & Vargas, R. (2019). Downscaling satellite soil moisture using geomorphometry and machine learning. PloS One, 14(9), e0219639.
Hadas, A. (1977). Heat transfer in dry aggregated soil: I. heat conduction. Soil Science Society of America Journal, 41(6), 1055-1059.
Hamrani, A., Akbarzadeh, A., & Madramootoo, C. A. (2020). Machine learning for predicting greenhouse gas emissions from agricultural soils. Science of The Total Environment, 741, 140338.
Hartmann, M., & Six, J. (2023). Soil structure and microbiome functions in agroecosystems. Nature Reviews Earth & Environment, 4, 4–18.
Heinse, R., et al. (2015). "Microgravity Oxygen Diffusion and Water Retention Measurements in Unsaturated Porous Media aboard the International Space Station." Vadose Zone Journal 14(6).
Hopmans, J.W. (2020). Transdisciplinary soil hydrology. Vadose Zone J.2020;19:e20085. https://doi.org/10.1002/vzj2.20085
Huang, C. H., Chen, P. J., Lin, Y. J., Chen, B. W., & Zheng, J. X. (2021). A robot-based intelligent management design for agricultural cyber-physical systems. Computers and Electronics in Agriculture, 181, 105967.
Huang, J., Desai, A. R., Zhu, J., Hartemink, A. E., Stoy, P. C., Loheide, S. P., ... & Arriaga, F. (2020). Retrieving heterogeneous surface soil moisture at 100 m across the globe via fusion of remote sensing and land surface parameters. Frontiers in Water, 2, 578367.
Huang, J., McBratney, A. B., Minasny, B., & Triantafilis, J. (2017). Monitoring and modelling soil water dynamics using electromagnetic conductivity imaging and the ensemble Kalman filter. Geoderma, 285, 76-93.
Huang, J., Scudiero, E., Clary, W., Corwin, D. L., & Triantafilis, J. (2017). Time‐lapse monitoring of soil water content using electromagnetic conductivity imaging. Soil Use and Management, 33(2), 191-204. https://doi.org/10.1111/sum.12261
Huang, W. Q., & Chen, K. Y. (2023). Fuzzy Inference Soil Analysis System for Automated Vehicles in Honey Tangerine Orchards. International Journal of Fuzzy Systems, 1-12.
Hudson, B.D. (1994). Soil organic matter and available water capacity. Journal of Soil and Water Conservation, 49(2), 189.
Huisman, J. A., Hubbard, S. S., Redman, J. D., & Annan, A. P. (2003). Measuring soil water content with ground penetrating radar: A review. Vadose zone journal, 2(4), 476-491. https://doi.org/10.2113/2.4.476
Hussain, A., Rasul, G., Mahapatra, B., & Tuladhar, S. (2016). Household food security in the face of climate change in the Hindu-Kush Himalayan region. Food Security, 8, 921-937.
Iizumi, T., & Wagai, R. (2019). Leveraging drought risk reduction for sustainable food, soil and climate via soil organic carbon sequestration. Scientific Reports, 9(1), 19744.
Jana, R. B., Mohanty, B. P., & Sheng, Z. (2012). Upscaling soil hydraulic parameters in the Picacho Mountain region using Bayesian neural networks. Transactions of the ASABE, 55(2), 463- 473.
Jaumann, S., & Roth, K. (2018). Soil hydraulic material properties and layered architecture from time-lapse GPR. Hydrology and Earth System Sciences, 22(4), 2551-2573.
Jayarathne, J. R. R. N., T. K. K. Chamindu Deepagoda, T. J. Clough, M. C. M. Nasvi, S. Thomas, B. Elberling, & K. Smits. (2020). Gas‐Diffusivity based characterization of aggregated agricultural soils. Soil Science Society of America Journal, 84(2), 387-398. doi:10.1002/saj2.20033
Jian, J., Du, X., & Stewart, R.D. (2020). A database for global soil health assessment. Scientific Data, 7(1), 16.
Johnson, M. S., & Lehmann, J. (2006). Double-funneling of trees: Stemflow and root-induced preferential flow. Ecoscience, 13(3), 324-333.
Kang, S., Knight, R., & Goebel, M. (2022). Improved imaging of the large-scale structure of a groundwater system with airborne electromagnetic data. Water Resources Research, 58, e2021WR031439. https://doi.org/10.1029/2021WR031439
Kerloch, E., and J.-C. Michel. 2015. Pore Tortuosity and Wettability as Main Characteristics of the Evolution of Hydraulic Properties of Organic Growing Media during Cultivation. Vadose Zone J. 14(6): vzj2014.11.0162. doi: 10.2136/vzj2014.11.0162.
Kerr, Y. H., Waldteufel, P., Richaume, P., Wigneron, J. P., Ferrazzoli, P., Mahmoodi, A., ... & Delwart, S. (2012). The SMOS soil moisture retrieval algorithm. IEEE transactions on geoscience and remote sensing, 50(5), 1384-1403. 10.1109/TGRS.2012.2184548
Klotzsche, A., Jonard, F., Looms, M. C., van der Kruk, J., & Huisman, J. A. (2018). Measuring soil water content with ground penetrating radar: A decade of progress. Vadose Zone Journal, 17(1), 1-9.
Koestel, J., Kemna, A., Javaux, M., Binley, A., & Vereecken, H. (2008). Quantitative imaging of solute transport in an unsaturated and undisturbed soil monolith with 3‐D ERT and TDR. Water resources research, 44(12).
Köhne, J.M., S. Köhne, and J. Šimůnek. 2006. Multi-process herbicide transport in structured soil columns: Experiment and model analysis. J. Contam. Hydrol. 85:1–32.
Kollet, S. J., and R. M. Maxwell (2006), Integrated surface–groundwater flow modeling: A free- surface overland flow boundary condition in a parallel groundwater flow model, Advances in Water Resources, 29(7), 945–958, doi:10.1016/j.advwatres.2005.08.006.
Krause, S., Lewandowski, J., Grimm, N. B., Hannah, D. M., Pinay, G., McDonald, K., ... & Turk, V. (2017). Ecohydrological interfaces as hot spots of ecosystem processes. Water Resources Research, 53(8), 6359-6376.
Kristensen, A. H., A. Thorbjørn, M. P. Jensen, M. Pedersen, & P. Moldrup. (2010). Gas-phase diffusivity and tortuosity of structured soils. J Contam Hydrol, 115(1), 26-33.
Krueger, E.S., Levi, M.R., Achieng, K.O., Bolten, J.D., Carlson, J.D., Coops, N.C., Holden, Z.A., Magi, B.I., Rigden, A.J. and Ochsner, T.E., 2022. Using soil moisture information to better understand and predict wildfire danger: a review of recent developments and outstanding questions. International Journal of Wildland Fire.
Kurtzman, D., & Scanlon, B. R. (2011). Groundwater recharge through vertisols: irrigated cropland vs. natural land, Israel. Vadose Zone Journal, 10(2), 662-674.
Kuzyakov, Y., & Blagodatskaya, E. (2015). Microbial hotspots and hot moments in soil: concept & review. Soil Biology and Biochemistry, 83, 184-199.
Lacetera, N., 2019. Impact of climate change on animal health and welfare. Animal Frontiers, 9(1), pp.26-31.
Lado, M., Paz, A., & Ben-Hur, M. (2004). Organic matter and aggregate size interactions in infiltration, seal formation, and soil loss. Soil Science Society of America Journal, 68(3), 935-942.
Lal, R. (2004). Soil carbon sequestration impacts on global climate change and food security. Science, 304(5677), 1623-1627.
Lal, R. (2011). Soil health and climate change: An overview. In: B.P. Singh, A.L. Cowie, K.Y. Chan (Eds.), Soil Health and Climate Change. Springer Berlin Heidelberg, Berlin, Heidelberg, pp. 3-24.
Lal, R. (2014). Societal value of soil carbon. Journal of Soil and Water Conservation, 69(6), 186A-192A.
Leij, F.J, A. Sciortino, A.W.(2006) Warrick, Infiltration in two parallel soil columns, Water Resour. Res., 42 (12) , pp. 1-15.
Leij, F. J., Ghezzehei, T. A., & Or, D. (2002). Modeling the dynamics of the soil pore-size distribution. Soil and Tillage Research, 64(1-2), 61-78.
Li, Q., Zhu, Y., Shangguan, W., Wang, X., Li, L., & Yu, F. (2022). An attention-aware LSTM model for soil moisture and soil temperature prediction. Geoderma, 409, 115651.
Libohova, Z., Seybold, C., Wysocki, D., Wills, S., Schoeneberger, P., Williams, C., Lindbo, D., Stott, D., & Owens, P.R. (2018). Reevaluating the effects of soil organic matter and other properties on available water-holding capacity using the National Cooperative Soil Survey Characterization Database. Journal of Soil and Water Conservation, 73(4), 411-421.
Lu, J., Zhang, Q., Werner, A.D., Li, Y., Jiang, S., & Tan, Z. (2020). Root-induced changes of soil hydraulic properties-A review. Journal of Hydrology, 589, 125203.
Mair, A., Dupuy, L. X., & Ptashnyk, M. (2022). Model for water infiltration in vegetated soil with preferential flow oriented by plant roots. Plant and Soil, 478(1-2), 709-729.
Maxwell, R. M., M. Putti, S. Meyerhoff, J. Delfs, I.M. Ferguson, V. Ivanov, J. Kim, O. Kolditz, S.J. Kollet, M. Kumar, S. Lopez, J. Niu, C. Paniconi, Y. Park, M.S. Phanikumar, C. Shen, E.A. Sudicky, and M. Sulis (2014), Surface-subsurface model intercomparison: A first set of benchmark results to diagnose integrated hydrology and feedbacks, Water Resources Research, 50, 1531-1549, doi:10.1002/2013WR013725.
McClain, M. E., Boyer, E. W., Dent, C. L., Gergel, S. E., Grimm, N. B., Groffman, P. M., ... & Pinay, G. (2003). Biogeochemical hot spots and hot moments at the interface of terrestrial and aquatic ecosystems. Ecosystems, 301-312.
McCourty, M. A., Gyawali, A. J., & Stewart, R. D. (2018). Of macropores and tillage: influence of biomass incorporation on cover crop decomposition and soil respiration. Soil Use and Management, 34(1), 101-110.
Meixner, T., A. Manning, D. Stonestrom, D.M. Allen, H. Ajami, K. Blasch, A. Brookfield, C.L. Castro, Clark, J.F., D. Gochis, A. Flint, K. Neff, R. Niraula, M. Rodell, B. Scanlon, K. Singha, M. Walvoord. 2016. Implications of prospective climate change for groundwater recharge in the western United States, Journal of Hydrology, doi:10.1016/j.jhydrol.2015.12.027
Meurer, K., Barron, J., Chenu, C., Coucheney, E., Fielding, M., Hallett, P., Herrmann, A.M., Keller, T., Koestel, J., Larsbo, M., Lewan, E., Or, D., Parsons, D., Parvin, N., Taylor, A., Vereecken, H., & Jarvis, N. (2020). A framework for modelling soil structure dynamics induced by biological activity. Global Change Biology, 26, 5382-5403.
Michot, D., Benderitter, Y., Dorigny, A., Nicoullaud, B., King, D., & Tabbagh, A. (2003). Spatial and temporal monitoring of soil water content with an irrigated corn crop cover using surface electrical resistivity tomography. Water Resources Research, 39(5).
Minasny, B., & McBratney, A.B. (2018). Limited effect of organic matter on soil available water capacity. European Journal of Soil Science, 69(1), 39-47.
Mohammed, A.K., Hirmas, D.R., Nemes, A., & Giménez, D. (2020). Exogenous and endogenous controls on the development of soil structure. Geoderma, 357, 113945.
Mohanty, B. P. (2013). Soil hydraulic property estimation using remote sensing: A review. Vadose Zone Journal, 12(4), vzj2013-06.
Montzka, C., Rötzer, K., Bogena, H. R., Sanchez, N., & Vereecken, H. (2018). A new soil moisture downscaling approach for SMAP, SMOS, and ASCAT by predicting sub-grid variability. Remote sensing, 10(3), 427. https://doi.org/10.3390/rs10030427
Naasz, R., J.-C. Michel, and S. Charpentier. 2005. Measuring Hysteretic Hydraulic Properties of Peat and Pine Bark using a Transient Method. Soil Sci. Soc. Am. J. 69(1): 13. doi: 10.2136/sssaj2005.0013.
Naasz, R., J.-C. Michel, and S. Charpentier. 2008. Water repellency of organic growing media related to hysteretic water retention properties. Eur. J. Soil Sci. 59, 156-165. doi: 10.1111/j.1365-2389.2007.00966.x.
Newbery, F., Qi, A., & Fitt, B. D. (2016). Modeling impacts of climate change on arable crop diseases: progress, challenges and applications. Current opinion in plant biology, 32, 101- 109.
Niswonger, R. G., Prudic, D. E., & Regan, R. S. (2006). Documentation of the unsaturated-zone flow (UZF1) package for modeling unsaturated flow between the land surface and the water table with MODFLOW-2005 (No. 6-A19).
Norby, R. J., & Zak, D. R. (2011). Ecological lessons from free-air CO2 enrichment (FACE) experiments. Annual review of ecology, evolution, and systematics, 42, 181-203.
Novick, K. A., Ficklin, D. L., Baldocchi, D., Davis, K. J., Ghezzehei, T. A., Konings, A. G., ... & Wood, J. D. (2022). Confronting the water potential information gap. Nature Geoscience, 15(3), 158-164.
Ochsner, T. E., Cosh, M. H., Cuenca, R. H., Dorigo, W. A., Draper, C. S., Hagimoto, Y., ... & Zreda, (2013). State of the art in large‐scale soil moisture monitoring. Soil Science Society of America Journal, 77(6), 1888-1919.
Ojha, R., Corradini, C., Morbidelli, R. and Govindaraju, R.S., 2017. Effective saturated hydraulic conductivity for representing field-scale infiltration and surface soil moisture in heterogeneous unsaturated soils subjected to rainfall events. Water, 9(2), p.134.
Oki, T. and Kanae, S., 2006. Global hydrological cycles and world water resources. science, 313(5790), pp.1068-1072.
Or, D., & Ghezzehei, T. A. (2002). Modeling post-tillage soil structural dynamics: a review. Soil and Tillage Research, 64(1-2), 41-59.
Owens, P. N. (2020). Soil erosion and sediment dynamics in the Anthropocene: a review of human impacts during a period of rapid global environmental change. Journal of Soils and Sediments, 20, 4115-4143.
Paniconi, C., and M. Putti, 2015. Physically based modeling in catchment hydrology at 50: Survey and outlook, Water Resour. Res., 51(9), 7090–7129, doi:10.1002/2015WR017780.
Peng, J., Loew, A., Merlin, O., & Verhoest, N. E. (2017). A review of spatial downscaling of satellite remotely sensed soil moisture. Reviews of Geophysics, 55(2), 341-366. https://doi.org/10.1002/2016RG000543
Peng, W., Lu, Y., Xie, X., Ren, T., & Horton, R. (2019). An improved thermo‐TDR technique for monitoring soil thermal properties, water content, bulk density, and porosity. Vadose Zone Journal, 18(1), 1-9.
Pereira, P., Bogunovic, I., Muñoz-Rojas, M., & Brevik, E. C. (2018). Soil ecosystem services, sustainability, valuation and management. Current Opinion in Environmental Science & Health, 5, 7-13.
Perzan, Z., Osterman, G., Maher, K., 2023. Controls on flood managed aquifer recharge through a heterogeneous vadose zone: hydrologic modeling at a site characterized with surface geophysics. Hydrol. Earth Syst. Sci. 27, 969–990. https://doi.org/10.5194/hess-27- 969-2023
Potopová, V., Zahradníček, P., Štěpánek, P., Türkott, L., Farda, A., & Soukup, J. (2017). The impacts of key adverse weather events on the field‐grown vegetable yield variability in the Czech Republic from 1961 to 2014. International Journal of Climatology, 37(3), 1648-1664. Protopapas, A.L. R.L. Bras, (1991) The one-dimensional approximation for infiltration in heterogeneous soils, Water Resour. Res., 27 (6), pp. 1019-1027
Quiring, S. M., Ford, T. W., Wang, J. K., Khong, A., Harris, E., Lindgren, T., ... & Li, Z. (2016). The North American soil moisture database: Development and applications. Bulletin of the American Meteorological Society, 97(8), 1441-1459.
Radolinski, J., Le, H., Hilaire, S. S., Xia, K., Scott, D., & Stewart, R. D. (2022). A spectrum of preferential flow alters solute mobility in soils. Scientific Reports, 12(1), 4261.
Radolinski, J., Wu, J., Xia, K., & Stewart, R. (2018). Transport of a neonicotinoid pesticide, thiamethoxam, from artificial seed coatings. Science of the Total Environment, 618, 561-568.
Raviv, M., J.H. Lieth, and A. Bar-Tal, eds., 2019. Soilless culture: Theory and practice: Theory and practice. Elsevier.
Razafimbelo, T.M., Albrecht, A., Oliver, R., Chevallier, T., Chapuis-Lardy, L., & Feller, C. (2008).
Aggregate associated-C and physical protection in a tropical clayey soil under Malagasy conventional and no-tillage systems. Soil and Tillage Research, 98(2), 140-149.
Ren, T., Ochsner, T. E., & Horton, R. (2003). Development of thermo-time domain reflectometry for vadose zone measurements. Vadose Zone Journal, 2(4), 544-551.
Reyes, J., O. Wendroth, C. Matocha, J. Zhu, W. Ren, and A.D. Karathanasis. 2018. Reliably mapping clay content coregionalized with electrical conductivity. Soil Sci. Soc. Am. J. 82:578-592. doi:10.2136/sssaj2017.09.0327.
Richter, D. D., Bacon, A. R., Brecheisen, Z., & Mobley, M. L. (2015, June). Soil in the Anthropocene. In IOP Conference Series: Earth and Environmental Science (Vol. 25, No. 1, p. 012010). IOP Publishing.
Rillig, M.C., Lehmann, A., Aguilar-Trigueros, C.A., Antonovics, J., Caruso, T., Hempel, S., Lehmann, J., Valyi, K., Verbruggen, E., Veresoglou, S.D., & Powell, J.R. (2016). Soil microbes and community coalescence. Pedobiologia, 59(1), 37-40.
Rillig, M.C., Muller, L.A.H., & Lehmann, A. (2017). Soil aggregates as massively concurrent evolutionary incubators. The ISME Journal, 11(9), 1943-1948.
Ritsema, C.J., Dekker, L.W., Hendrickx, J.M.H. and Hamminga, W., 1993. Preferential flow mechanism in a water repellent sandy soil. Water Resources Research, 29(7), pp.2183-2193. Robichaud, P.R., Wagenbrenner, J.W., Pierson, F.B., Spaeth, K.E., Ashmun, L.E. and Moffet, C.A., 2016. Infiltration and interrill erosion rates after a wildfire in western Montana, USA. Catena, 142, pp.77-88.
Robinson, D. A., Abdu, H., Lebron, I., & Jones, S. B. (2012). Imaging of hill-slope soil moisture wetting patterns in a semi-arid oak savanna catchment using time-lapse electromagnetic induction. Journal of Hydrology, 416, 39-49. https://doi.org/10.1016/j.jhydrol.2011.11.034
Robinson, D. A., Campbell, C. S., Hopmans, J. W., Hornbuckle, B. K., Jones, S. B., Knight, R., ... & Wendroth, O. (2008). Soil moisture measurement for ecological and hydrological watershed- scale observatories: A review. Vadose zone journal, 7(1), 358-389.
Russo, Stochastic analysis of simulated vadose zone solute transport in a vertical cross section of heterogeneous soil during nonsteady water flow, Water Resour. Res., 27 (3) (1991), pp. 267- 283
Sadeghi, M., Babaeian, E., Tuller, M., & Jones, S. B. (2017). The optical trapezoid model: A novel approach to remote sensing of soil moisture applied to Sentinel-2 and Landsat-8 observations. Remote sensing of environment, 198, 52-68. https://doi.org/10.1016/j.rse.2017.05.041
Sadeghi, M., Ebtehaj, A., Crow, W.T., Gao, L., Purdy, A.J., Fisher, J.B., Jones, S.B., Babaeian, E. and Tuller, M., 2020. Global estimates of land surface water fluxes from SMOS and SMAP satellite soil moisture data. Journal of Hydrometeorology, 21(2), pp.241-253.
Sadeghi, M., Hatch, T., Huang, G., Bandara, U., Ghorbani, A. and Dogrul, E.C., 2022. Estimating soil water flux from single-depth soil moisture data. Journal of Hydrology, 610, p.127999. Schlenker, W., & Roberts, M. J. (2009). Nonlinear temperature effects indicate severe damages to US crop yields under climate change. Proceedings of the National Academy of sciences, 106(37), 15594-15598.
Sasidharan, S., Bradford, S.A., Simunek, J., De Jong, B., S, R.K., 2018a. Evaluating Drywells for Stormwater Management and Enhanced Aquifer Recharge. Adv Water Resour, 116: 167-177. DOI:10.1016/j.advwatres.2018.04.003
Schlögl, J., Wimmer, B., Cramaro, L., Wirsching, J., Poll, C., Pagel, H., ... & Haderlein, S. B. (2022). Heavy rainfall following a summer drought stimulates soil redox dynamics and facilitates rapid and deep translocation of glyphosate in floodplain soils. Environmental Science: Processes & Impacts, 24(5), 825-838.
Scholer, M., Irving, J., Binley, A., & Holliger, K. (2011). Estimating vadose zone hydraulic properties using ground penetrating radar: The impact of prior information. Water Resources Research, 47(10).
Schübl, M., Stumpp, C., & Brunetti, G. (2022). A Bayesian perspective on the information content of soil water measurements for the hydrological characterization of the vadose zone. Journal of Hydrology, 613, 128429.
Sharma, S., Verma, K., & Hardaha, P. (2023). Implementation of artificial intelligence in agriculture. Journal of Computational and Cognitive Engineering, 2(2), 155-162.
Šimůnek, J., Van Genuchten, M.T. and Šejna, M., 2016. Recent developments and applications of the HYDRUS computer software packages. Vadose Zone Journal, 15(7).DOI: 10.2136/vzj2016.04.0033
Šimůnek, J. (2005). 78: Models of Water Flow and Solute Transport in the Unsaturated Zone. Encyclopedia of Hydrological Sciences, (1), 1–10. https://doi.org/10.1002/0470848944.
Šimůnek, J. and M. T. van Genuchten (2008). "Modeling Nonequilibrium Flow and Transport Processes Using HYDRUS." Vadose Zone Journal 7(2): 782.
Šimůnek J. Jarvis N.J. van Genuchten M.Th. Gardenas A.. 2003. Review and comparison of models for describing non-equilibrium and preferential flow and transport in the vadose zone. J. Hydrol. 272:14–35. doi:10.1016/S0022-1694(02)00252-4
Six, J., Conant, R.T., Paul, E.A., & Paustian, K. (2002). Stabilization mechanisms of soil organic matter: Implications for C-saturation of soils. Plant and Soil, 241(2), 155-176.
Six, J., Paustian, K., Elliott, E.T., & Combrink, C. (2000). Soil structure and organic matter I. Distribution of aggregate-size classes and aggregate-associated carbon. Soil Science Society of America Journal, 64(2), 681-689.
Skendžić, S., Zovko, M., Živković, I.P., Lešić, V. and Lemić, D., 2021. The impact of climate change on agricultural insect pests. Insects, 12(5), p.440.
Sonnert, G., Fox, M.F. and Adkins, K. (2007), Undergraduate Women in Science and Engineering: Effects of Faculty, Fields, and Institutions Over Time. Social Science Quarterly, 88: 1333-1356. https://doi.org/10.1111/j.1540-6237.2007.00505.x
Stewart, R. D., Abou Najm, M. R., Rupp, D. E., Lane, J. W., Uribe, H. C., Arumí, J. L., & Selker, J. S. (2015). Hillslope run‐off thresholds with shrink–swell clay soils. Hydrological Processes, 29(4), 557-571.
Stewart, R. D., Abou Najm, M. R., Rupp, D. E., & Selker, J. S. (2016). Modeling multidomain hydraulic properties of shrink‐swell soils. Water Resources Research, 52(10), 7911-7930.
Stewart, R. D., Rupp, D. E., Abou Najm, M. R., & Selker, J. S. (2016). A unified model for soil shrinkage, subsidence, and cracking. Vadose Zone Journal, 15(3).
Stewart, R. D. (2019). A generalized analytical solution for preferential infiltration and wetting. Vadose Zone Journal, 18(1), 1-10.
Sullivan, P. L., Billings, S. A., Hirmas, D., Li, L., Zhang, X., Ziegler, S., ... & Wen, H. (2022). Embracing the dynamic nature of soil structure: A paradigm illuminating the role of life in critical zones of the Anthropocene. Earth-Science Reviews, 225, 103873.
Talukder, R., D. Plaza-Bonilla, C. Cantero-Martínez, O. Wendroth, and J. Lampurlanés. 2023. Soil hydraulic properties and pore dynamics under different tillage and irrigated crop sequences. Geoderma 430: 116293, doi.org/10.1016/j.geoderma.2022.116293.
Tisdall, J.M., & Oades, J.M. (1982). Organic matter and water-stable aggregates in soils. Journal of Soil Science, 33(2), 141-163.
Tuli, A., Wei, J. B., Shaw, B. D., & Hopmans, J. W. (2009). In Situ monitoring of soil solution Nitrate: Proof of concept. Soil Science Society of America Journal, 73(2), 501-509.
Twarakavi, N. K. C., Šimůnek, J., & Seo, S. (2008). Evaluating interactions between groundwater and vadose zone using the HYDRUS-based flow package for MODFLOW. Vadose Zone Journal, 7(2), 757-768.
Usowicz, B., Lipiec, J., Usowicz, J. B., & Marczewski, W. (2013). Effects of aggregate size on soil thermal conductivity: Comparison of measured and model-predicted data. International Journal of Heat and Mass Transfer, 57(2), 536-541.
Vanwalleghem, T., Gómez, J. A., Amate, J. I., De Molina, M. G., Vanderlinden, K., Guzmán, G., ... & Giráldez, J. V. (2017). Impact of historical land use and soil management change on soil erosion and agricultural sustainability during the Anthropocene. Anthropocene, 17, 13-29.
Vaughan, K., Van Miegroet, H., Pennino, A., Pressler, Y., Duball, C., Brevik, E.C., Berhe, A.A. and Olson, C. (2019), Women in Soil Science: Growing Participation, Emerging Gaps, and the Opportunities for Advancement in the USA. Soil Sci. Soc. Am. J., 83: 1278-1289. https://doi.org/10.2136/sssaj2019.03.0085
Velásquez, A. C., Castroverde, C. D. M., & He, S. Y. (2018). Plant–pathogen warfare under changing climate conditions. Current biology, 28(10), R619-R634.
Vereecken, H., Kasteel, R., Vanderborght, J., & Harter, T. (2007). Upscaling hydraulic properties and soil water flow processes in heterogeneous soils: A review. Vadose Zone Journal, 6(1), 1-28. https://doi.org/10.2136/vzj2006.0055
Vergopolan, N., Chaney, N. W., Pan, M., Sheffield, J., Beck, H. E., Ferguson, C. R., ... & Wood, E. F. (2021). SMAP-HydroBlocks, a 30-m satellite-based soil moisture dataset for the conterminous US. Scientific data, 8(1), 264.
Vos, M., Wolf, A.B., Jennings, S.J., & Kowalchuk, G.A. (2013). Micro-scale determinants of bacterial diversity in soil. FEMS Microbiology Reviews, 37(6), 936-954.
Wang, B., Brewer, P.E., Shugart, H.H., Lerdau, M.T., & Allison, S.D. (2019). Soil aggregates as biogeochemical reactors and implications for soil-atmosphere exchange of greenhouse gases- A concept. Global Change Biology, 25(2), 373-385.
Wang, L., Z. Gao, and R. Horton (2010), Comparison of six algorithms to determine the soil apparent thermal diffusivity at a site in the loess plateau of china, Soil Sci., 175(2), 51–60, doi:10.1097/SS.0b013e3181cdda3f.
Warren, J. M., Jensen, A. M., Ward, E. J., Guha, A., Childs, J., Wullschleger, S. D., & Hanson, P. J. (2021). Divergent species‐specific impacts of whole ecosystem warming and elevated CO2 on vegetation water relations in an ombrotrophic peatland. Global Change Biology, 27(9), 1820-1835.
Weihermüller, L., Huisman, J. A., Lambot, S., Herbst, M., & Vereecken, H. (2007). Mapping the spatial variation of soil water content at the field scale with different ground penetrating radar techniques. Journal of hydrology, 340(3-4), 205-216. https://doi.org/10.1016/j.jhydrol.2007.04.013
Weisbrod, N., Dragila, M. I., Nachshon, U., & Pillersdorf, M. (2009). Falling through the cracks: The role of fractures in Earth‐atmosphere gas exchange. Geophysical Research Letters, 36(2).
Wellman, D.M., R.E. Gephart, M.J. Truex, M.B. Triplett, M.D. Freshley and T.C. Johnson. 2011. Implementation Plan for the Deep Vadose Zone-Applied Field Research Center. PNNL- 20209, Pacific Northwest National Laboratory, Richland, Washington.
Wendroth, O., Koszinski, S., & Pena-Yewtukhiv, E. (2006). Spatial association among soil hydraulic properties, soil texture, and geoelectrical resistivity. Vadose Zone Journal, 5(1), 341-355.
Wolbrecht, C. and Campbell, D.E. (2007), Leading by Example: Female Members of Parliament as Political Role Models. American Journal of Political Science, 51: 921-939. https://doi.org/10.1111/j.1540-5907.2007.00289.x
Wyatt, B.M., J.M. DeBruyn, M.K. Walia, M. Holzer, J. Montgomery, S. Chapman. 2022. Improving Soil Science Education through K-12 Teacher Workshops. ASA, CSSA, SSSA International Annual Meeting, Baltimore, MD.
Xu, M., Yao, N., Yang, H., Xu, J., Hu, A., de Goncalves, L. G. G., & Liu, G. (2022). Downscaling SMAP soil moisture using a wide & deep learning method over the Continental United States. Journal of Hydrology, 609, 127784. https://doi.org/10.1016/j.jhydrol.2022.127784
Yang, K., Koike, T., Ye, B. and Bastidas, L., 2005. Inverse analysis of the role of soil vertical heterogeneity in controlling surface soil state and energy partition. Journal of Geophysical Research: Atmospheres, 110(D8).
Yang Yang, Xintong Wu, Tao He, Ying Wang, Ole Wendroth, Xinyi Chen, Baoyuan Liu, and Guanghui Zhang. 2022. Factors controlling saturated hydraulic conductivity along a typical black soil slope. Soil Till. Res. 220: 105391. https://doi.org/10.1016/j.still.2022105391
Yin, S., Tursunniyaz, M., Huang, J., & Andrews, J. (2021, October). Dual-Printed Soil Sensors for Nitrate and Moisture Monitoring. In 2021 IEEE Sensors (pp. 1-4). IEEE.
Yu, Y., Huisman, J. A., Klotzsche, A., Vereecken, H., & Weihermüller, L. (2022). Coupled full- waveform inversion of horizontal borehole ground penetrating radar data to estimate soil hydraulic parameters: A synthetic study. Journal of Hydrology, 610, 127817.
Zhang, X., J. Zhu, O. Wendroth, C. Matocha, and D. Edwards. 2019. Effect of macroporosity on pedotransfer function estimates at the field scale. Vadose Zone J. 18:180151. doi:10.2136/vzj2018.08.0151.
Zhang, Y., & Schaap, M. G. (2017). Weighted recalibration of the Rosetta pedotransfer model with improved estimates of hydraulic parameter distributions and summary statistics (Rosetta3). Journal of Hydrology, 547, 39-53.
Zhou, T., Šimůnek, J., & Braud, I. (2021). Adapting HYDRUS-1D to simulate the transport of soil water isotopes with evaporation fractionation. Environmental Modelling & Software, 143, 105118.
Zhu, J., B.P. Mohanty, 1 (2002a) Spatial averaging of van Genuchten hydraulic parameters for steady-state fow in heterogeneous soils: a numerical study, Vadose Zone J., , pp. 251-272.
Zhu, J., B.P. Mohanty (2002b), Upscaling of soil hydraulic properties for steady state evaporation and infiltration, Water Resour. Res., 38 (9).
Zhu, Y., Chen, Y., Ali, M. A., Dong, L., Wang, X., Archontoulis, S. V., ... & Castellano, M. J. (2021). Continuous in situ soil nitrate sensors: the importance of high‐resolution measurements across time and a comparison with salt extraction‐based methods. Soil Science Society of America Journal, 85(3), 677-690.
Zipper, S.C., Qiu, J., & Kucharik, C.J. (2016). Drought effects on US maize and soybean production: Spatiotemporal patterns and historical changes. Environmental Research Letters, 11(9), 094021.
Zreda, M., Desilets, D., Ferré, T. P. A., & Scott, R. L. (2008). Measuring soil moisture content non‐ invasively at intermediate spatial scale using cosmic‐ray neutrons. Geophysical research letters, 35(21). https://doi.org/10.1029/2008GL035655