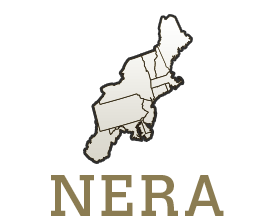
NE2443: Biology, Ecology & Management of Emerging Disease Vectors
(Multistate Research Project)
Status: Active
NE2443: Biology, Ecology & Management of Emerging Disease Vectors
Duration: 10/01/2024 to 09/30/2029
Administrative Advisor(s):
NIFA Reps:
Non-Technical Summary
Nearly 20 percent of the infectious diseases reported worldwide today are caused by vector-borne pathogens, posing a major threat to farmers, forestry workers, and families living in urban, suburban, and rural areas in the US. With few available vaccines and new vector-borne diseases emerging at a steady rate, minimizing human exposure to arthropod disease vectors (e.g., mosquitoes, sandflies, kissing bugs, ticks) and managing vector populations remain the primary methods for reducing vector-borne disease risk. Our ability to control vector-borne disease threats is limited by our understanding of vector ecology, vector physiology, vector behavior, and the biology of vector-pathogen interactions. Thus, our research will (1) develop and strengthen effective surveillance and monitoring of disease vectors and their associated pathogens at local and regional scales; (2) determine the ecology, physiology, genetics and/or geographic and temporal distributions of historical, extant, and emerging disease vectors and the pathogens they transmit; and (3) discover, develop, and integrate interventions to manage vector-borne pathogen transmission and pesticide resistance. Our team is composed of top entomologists, microbiologists, ecologists, wildlife biologists, and veterinarians who study vector and vector-borne pathogen biology. This work cannot be completed without a multistate effort. In a rapidly changing landscape, the experience and guidance of one region is critical to others. The project will benefit all US residents by understanding, assessing, and mitigating the threat posed by arthropod vectors of health, veterinary, and wildlife importance, and provide for and encourage environmentally sound, scientifically based, and professional control by public health and pest control agencies.
Statement of Issues and Justification
The need as indicated by stakeholders. According to the World Health Organization (WHO), around 17% of the infectious diseases reported worldwide are caused by vector-borne pathogens. In the US, outbreaks of vector-borne diseases are aided by human travel and the introduction of infected vectors into new areas. For example, in 2023, cases of locally acquired malaria were reported in Maryland, Texas, and Florida caused by Plasmodium vivax and Plasmodium falciparum, leading the US Centers for Disease Control and Prevention (CDC) to issue a national alert. Dengue outbreaks have been reported in Florida, where several serotypes of the virus have been detected in mosquitoes, increasing the risk of severe dengue (Coatsworth et al. 2022). Likewise, autochthonous transmission of leishmaniasis occurs in Texas and Oklahoma (Curtin and Aronson 2021). Expanded geographic distributions of vector-borne diseases endemic to the US, such as Lyme disease and other tick-borne diseases, have been attributed to the spread of vectors into new locations (Eisen and Eisen 2023). According to the CDC, Lyme disease affects around 476,000 Americans each year primarily in the northeast with average treatment costs of $1,200 USD per individual (Hook et al 2022). Thus, vector-borne diseases represent an important risk and burden to the public, including farmers, forestry workers, and families living in urban, suburban, and rural areas in the US.
Invasive vector species threaten to alter the epidemiology and transmission dynamics of existing and new vector-borne diseases, and ultimately the health of humans, companion animals, wildlife, and livestock. Mosquito invaders include the yellow fever mosquito (Aedes aegypti) and the Asian tiger mosquito (Aedes albopictus), that have become established in the southeastern US and are increasingly reported in California (Metzger et al. 2015). While Ae. albopictus is moving northward, additional invasive mosquito species have also arrived. For example, the bush mosquito (Aedes japonicus) is a cold weather adapted species that has spread in suburban landscapes along the eastern seaboard, the northwestern US, the Florida panhandle, across the Hawaii volcanoes as well as Canada (Kaufman and Fonseca 2014, Jackson et al. 2016, Peterson et al. 2017), and the Australian Aedes notoscriptus has spread into southern California (Paterson and Campbell 2015). Over the last few years, we have witnessed the invasion and spread of the Asian longhorned tick (Haemaphysalis longicornis), which was first discovered on an Icelandic sheep in New Jersey in 2017 (Rainey et al. 2018) but was detected in archived records as far back as 2010, remaining misidentified and undetected for nearly a decade, if not longer (Beard et al. 2018). Through increased surveillance, it has since been detected in 19 states: Arkansas, Connecticut, Delaware, Georgia, Indiana, Kentucky, Maryland, Massachusetts, Missouri, New Jersey, New York, North Carolina, Ohio, Pennsylvania, Rhode Island, South Carolina, Tennessee, Virginia, and West Virginia (USDA APHIS, October 2023). US populations of H. longicornis are parthenogenetic (clonal) and small infestations can quickly proliferate.. Although humans are not favored hosts of H. longicornis, opportunistic feeding is well documented both in the native and invasive ranges (Bickerton and Toledo 2020, Wormser et al. 2020). In East Asia, H. longicornis transmits severe fever with thrombocytopenia syndrome virus (SFTSV), an emerging human tick-borne disease recently reclassified as Dabie bandavirus (Liu et al. 2015, Luo et al. 2015, Li et al. 2021). Under laboratory conditions, US lineages of H. longicornis can transmit the closely related Heartland virus (Rainey et al. 2022a) and Powassan virus (Rainey et al. 2022b), two native pathogenic viruses emergent in parts of the US. While H. longicornis is currently not perceived as a major public health threat in the US, this status may change given the enormous densities it can reach in favorable habitats (Bickerton and Toledo 2020, Schappach et al. 2020, González et al. 2023, Rochlin et al. 2023).
The importance of the work and consequences if not done. With few available vaccines and new pathogens emerging at a steady rate, minimizing human exposure to disease vectors and managing vector populations remain the primary methods for reducing vector-borne disease risks. Our ability to properly control vector-borne disease threats is limited by our understanding of vector ecology, vector physiology, vector behavior, and the biology of vector-pathogen interactions. The economic impact of arthropod-borne illness is devastating. Consider Lyme disease, the most prevalent vector-borne disease in the United States. It's been calculated to impose a substantial burden on the U.S. healthcare system, ranging from $712 million to $1.3 billion each year(Adrion et al 2015). This financial strain arises from extensive doctor visits and diagnostic testing, particularly evident in the year following initial diagnosis, as persistent symptoms like fatigue, musculoskeletal pain, and memory issues demand ongoing attention and care. . The total cumulative costs of reported WNV hospitalization cases in the US from 1999 through 2012 were around $800 million USD (about $77 million annually in today’s value after inflation adjustment; Staples et al. 2014). The estimated cost per human case of EEE is $3 million in 1995 dollars ($6.2 million after inflation adjustment; Villari et al. 1995). EEE and WNV both threaten the nation’s multi-billion-dollar equine industry. The mortality rate of horses infected with WNV is 34%; the rate for those with EEE approaches 100%. For example, in 2000, the estimated loss in New Jersey alone due to equine cases of WNV was $6 million ($11 million in 2024 value). Tourism, which increases human exposure to mosquitoes, is similarly impacted by outbreaks of mosquito-borne disease. The 2015-2017 outbreak of Zika virus, although generally mild in adults, was conclusively linked to severe birth defects, which carry a tremendous economic and emotional burden on affected families. Recent computational analyses estimate that even a mild Zika outbreak could cost the US in excess of $183 million in medical costs and productivity losses, whereas a severe outbreak could cost up to $1.2 billion.
The lessons learned from new and emerging mosquito- and tick-borne disease risks serve as an impetus for bolstering US research and translation to vector surveillance and management (Anyamba et al. 2014). Encouragingly, important advances are being made in areas that include new methods and tools for monitoring and the control of mosquitoes and ticks. However, the budgets for research and abatement programs follow a boom-and-bust cycle reactionary to emerging threats, which weakens the ability to sustain infrastructure necessary for managing vector-borne diseases (Kading et al. 2020). In a report released in early 2018, the CDC estimated that 80% of the nation’s vector control organizations lack the critical prevention and control capacities needed to combat the tripling of disease cases from mosquitoes, ticks, and sand flies reported over the past 13 years (CDC 2018). Improved sharing and coordination of research and translation to and standardization of monitoring and control tools are needed for researchers and control professionals to make informed decisions and better utilize limited resources.
The technical feasibility of the research. Our research will focus on critical gaps in our understanding of the vectors of disease agents in the U.S. These include mosquitoes, sandflies, kissing bugs, ticks, and other vectors of human pathogens. We will include research on the biology, spread, and control of other invasive vectors of regional importance. Our project will have three objectives:
- Develop and strengthen effective surveillance and monitoring of disease vectors (mosquitoes, ticks, etc.) and their associated pathogens at local and regional scales. Under this objective, project participants will leverage and strengthen existing surveillance programs in a coordinated fashion to yield robust comparable data across large geographic scales.
- Determine the ecology, physiology, genetics and/or geographic and temporal distributions of historical, extant, and emerging disease vectors and the pathogens they transmit. Under changing environmental conditions, these studies will enhance our ability to predict conditions leading to the spread or emergence of existing and novel diseases of public health importance.
- Discover, develop, and integrate interventions to manage vector-borne pathogen transmission and pesticide resistance.
Under each of these objectives, we have several sub-objectives to focus our project, which are described in the Methods section. Our objectives are technically feasible, but only if achieved by a coordinated team. Our team is composed of top entomologists, microbiologists, ecologists, wildlife biologists, and veterinarians who study vector and vector-borne pathogen biology, with the required experience and facilities and we are confident we can succeed with the proposed research.
The advantages of doing the work as a multistate effort. This work cannot be completed without a multistate effort. In a rapidly changing landscape, the experience and guidance of one region is critical to others. As a specific example, both Ae. aegypti and Ae. albopictus are new to California, and California abatement districts have benefited from the experience of those in the east coast states where these species are often the most common mosquito species. Conversely, researchers in Florida and Texas bring to the table experience working with populations affected with dengue and Zika, a set of skills that may be needed more broadly across the US. Finally, the biology that governs range expansion, vector competence, and ecology of different vector species will help to inform surveillance and management programs.
What the likely impacts will be from successfully completing the work. The project will build a highly collaborative network of experts to study existing and newly invasive vector species. The project will benefit all US residents by understanding, assessing, and mitigating the threat posed by arthropod vectors of health, veterinary, and wildlife importance. Our efforts will strengthen the capacity to detect and predict outbreaks of vector-borne diseases and evaluate the effectiveness of existing and novel control interventions under different environmental and social conditions. The project further provides for and encourages environmentally sound, scientifically based, and professional control by public health and pest control agencies. Our proposed research directly builds on a prior multistate project (NE 1943). Since 2019, we have met and exchanged published and unpublished results, have generated novel hypotheses from group participants and discussed coordination in research and sampling efforts. We have produced numerous accomplishments and deliverables and garnered competitive grants from newly formed collaborations. For this revision/replacement, we seek to expand our collaborative network to develop and strengthen a more coordinated region-wide and multi-state research effort.
Related, Current and Previous Work
Some of the key outcomes of the prior NE 1943 project include:
- Conducted active and passive surveillance of tick and tick-borne pathogen distributions at the county level across multiple states.
- Identified the first reported established population of Gulf Coast ticks (Amblyomma maculatum) infected with Rickettsia parkeri in Connecticut.
- Described the first occurrence of the soft tick Carios kelleyi (Cooley and Kohls) from New Jersey, based on larvae collected from big brown bats, Eptesicus fuscus.
- Described the regional spread of Culex coronator, an invasive mosquito species which has spread throughout the southern U.S. in the last 20 years.
- Conducted population genetic analyses of Borrelia burgdorferi in ticks and small mammals and avian malaria pathogen identification in native birds in Delaware.
- Examined environmental determinants of abundance of multiple disease vector species in their northern ranges.
- Conducted mosquito surveillance throughout the fall, winter, and spring to determine when mosquitoes enter and exit from their overwintering dormancy.
- Investigated how urban pollutants, like artificial light at night and higher temperatures associated with heat islands, affect mosquito dormancy in the lab and in the field.
- Evaluated the efficacy of several I. scapularis residential control strategies for homeowners, including perimeter treatments with a granular formulation of cyahalothrin, deployment of tick tubes,
- Assessed natural commercial alternatives to synthetic repellents with similar or better properties than DEET, evaluating the repellency of two extracts, CR3 and CR9, derived for newly developed catnip cultivars on two tick species, I. scapularis and H. longicornis.
- Tested the impacts of catch basin treatments, application of adulticides, and Autocidal Gravid Ovitraps on adult mosquito populations.
- Developed improved monitoring tools that combine internet-connected databases and quality control with the multiple trap types being used by mosquito abatement districts, e.g., B.G. traps, CDC traps, ovitraps, etc.
- Investigated the impacts of active forest management on the risk of exposure to I. scapularis.
- Explored the use of RNA interference (RNAi), a new technology that can provide highly specific control of insect pests, as well as established insecticides like Bacillus thuringiensis israelensis as new avenues of control of Culicoides midges and Aedes mosquitoes.
- Conducted research investigating the insecticidal and repellent activities of natural products derived from plants and microbes against mosquitoes, and elucidated the mechanism of action of how a plant-derived natural product binds to a biochemical receptor in mosquitoes that elicits repellent behaviors to facilitate the development of highly mosquito-specific repellents with minimal effects on humans.
- Investigated the effect of a potential novel biocontrol agent (the microsporidian parasite Edhazardia aedis) on the immune defense of Ae. aegypti mosquitoes.
- Performed research on the drug discovery for flavivirus infections, using in-silico screening approach, to screen compound libraries targeting the active site of viral RNA polymerase.
- Used genomic data to estimate population connectivity between locations and to inform various models aimed at forecasting mosquito and tick distribution and dispersal.
- Developed a next-generation sequencing platform for improved diagnostics for arthropods of medical importance to adapt existing technology for a platform for mosquito- and tick-borne diseases that can be used to identify up to 100,000 targets in a single reaction with a single sample.
- Built a reference database of Ae. aegypti mosquitoes worldwide based on microsatellite markers and genome-wide SNPs.
- Conducted research that sought to determine the role of epigenetics in the vectorial capacity of different tick populations, including the variation in methylation of I. scapularis collected in Minnesota (high-Lyme disease area) and Texas (low-Lyme disease area).
- Improved the characterization of the male-determining locus (M) in the dengue and Zika vector Ae. aegypti by determining the content of the ~160 Kilobase gap in the M-locus.
- Conducted entomological surveys coupled with knowledge attitudes and practices surveys to understand tick borne disease risk and perceptions of ticks and risk in park visitors on Staten Island as well as how ticks are monitored across public health and vector control districts in the USA.
- Developed an active surveillance citizen science project that seeks to understand the economic, environmental, and production factors that influence private forest landowners’ forest management decision-making processes and the implications for tick-borne disease exposure risk in Maine.
- Conducted a scoping review of Lyme disease provider-patient communication.
Objectives
-
1. Develop and strengthen effective surveillance and monitoring of disease vectors (mosquitoes, ticks, etc.) and their associated pathogens at local and regional scales. Under this objective, project participants will leverage and strengthen existing surveillance programs in a coordinated fashion to yield robust comparable data across large geographic scales.
-
2. Determine the ecology, physiology, genetics and/or geographic and temporal distributions of historical, extant, and emerging disease vectors and the pathogens they transmit. Under changing environmental conditions, these studies will enhance our ability to predict conditions leading to the spread or emergence of existing and novel diseases of public health importance.
-
3. Discover, develop, and integrate interventions to manage vector-borne pathogen transmission and pesticide resistance.
Methods
Objective 1: Develop and strengthen effective surveillance and monitoring of disease vectors (mosquitoes, ticks, etc.) and their associated pathogens at local and regional scales.
(1.1) Predict and model the distribution and density of the vectors of public and veterinary health importance in relation to habitat type and climate conditions using surveillance data within a One Health framework.
To better understand the interactions between pathogens, vectors, and hosts, it is critical to estimate habitat suitability for a given species. Referred to as species distribution models, multiple quantitative tools identify how environmental variables are most influential in predicting species occurrence, and as such create valuable tools for identifying high risk regions for vector-borne diseases or for predicting future changes in vector or pathogen geographic distributions given projected climate change. This sub-objective will utilize active tick sampling data obtained by drag sampling to associate environmental and climatic features of the landscape with the density of questing ticks or the density of infected ticks. This One Health project will estimate habitat suitability for tick-borne pathogens of human and animal health concern. In addition, we will leverage long-term datasets generated from statewide mosquito surveillance programs to develop descriptive and predictive models on the presence and abundance of mosquito vectors and their associated pathogens. For example, Connecticut maintains a network of 108 fixed mosquito-trapping stations throughout the state providing information that includes mosquito species composition and abundance in the community, seasonal and spatial distribution of mosquito vectors, and prevalence of virus infection in mosquito populations. Data from these efforts will be used to generate information on the habitat suitability of regionally important mosquito vectors and to assess potential risk of arbovirus epidemics by these vectors. We will explore the associations with climatic conditions, socio-economic factors, and land use patterns driving the persistence and abundance of both invasive and native vector species and their associated pathogens. This program of research will provide accurate information on the current and future distribution of medically important mosquito species and the spatial risk of arboviruses which is essential for targeting public health resources effectively. Finally, we will update the North American habitat suitability models for the soft tick Ornithodoros turicata (cite) given the threat of African swine fever virus that is spreading in Africa and Asia that emerged in the Caribbean in 2021. We will use multiple species distribution modeling approaches including MaxEnt, generalized linear models, and machine learning. Each approach will be evaluated based on multiple performance metrics. This is a multi-state effort as the updated occurrence data requires reaching out to collections at many institutions across the US.
(1.2): Improve the efficiency of surveillance programs vis-a-vis vector trapping and diagnostics.
Early detection ensures that vector control efforts are directed to the right location at the right time to avert an epidemic. A challenge with proactive surveillance needed for diverse vector and vector-borne disease threats is the need to identify unknown agents of concern (Holcomb et al. 2023). A significant limitation of most existing arbovirus surveillance is the reliance on qPCR for detection, which employs species-specific PCR primers for pathogens chosen a priori by investigators (Luce-Fedrow et al. 2015). With a rapidly changing vector-borne disease landscape, it may not always be possible to predict which pathogens are present as new threats spill over from zoonotic cycles, are introduced to unexpected places, or undergo genetic change (Kilpatrick and Randolph 2012, Vora et al. 2023). The advent of next generation sequencing has facilitated the ability to detect diverse arthropod vectors and both known and unknown (or undescribed) pathogens of concern. For example, new pipelines have been established for high-throughput screening of many tick-borne pathogens using next generation sequencing targeted multiplex PCR amplicon sequencing (Kingry et al. 2020, Hojgaard et al. 2020) ). Additionally, metagenomic sequencing of blood fed mosquitoes can reveal diverse human pathogens, a concept known as xenosurveillance (Batson et al. 2021). Finally, metabarcoding cannot only identify the vertebrates that vectors feed on (Fauver et al. 2017) but also identify the species composition of vectors in mixed pools (Mechei et al. 2021). This subobjective will advance surveillance pipelines capitalizing on high-throughput next generation (and further, single-molecule) sequencing to enumerate components of the vector holobiont – that is, the host and all associated microbial constituents that live on or in – identify pools of biting flies and ticks. using metagenomics. In addition, we will explore metagenomic sequencing pipelines for targeted and non-targeted screening of vectors for diverse agents of wildlife disease that include e.g., phlebotomine sand flies and midges of the genus Culicoides. Assessment of threats posed to both humans and animals by such vector arthropods properly frames our project in the context of a One Health approach – or the acknowledgement that human and animal health are inextricably linked in a tripartite manner to that of the environment in what is today a rapidly perturbed system. Finally, we will explore new surveillance techniques or modifications to existing traps that improve trap efficacy and improve operational vector control programs.
Objective 2: Determine the ecology, physiology, genetics and/or geographic and temporal distributions of historical, extant, and emerging disease vectors and the pathogens they transmit.
(2.1) Determine the phenology, habitat, and climatic requirements of historical, extant, and emerging vectors, hosts, and pathogens using historical and contemporary datasets.
Predicting when and where vectors, hosts, and vector-borne pathogens are present and interacting in the environment is critical for accurate and effective vector control and vector-borne disease prevention. Climatic and environmental variables that fluctuate across time and/or space can impact each of these factors. Changing temperatures as a result of global warming will result in mild winters and the expansion of vector populations into areas where they were not previously found. Additionally, alterations in land-use, including increased urbanization, will likely affect vector behavior and population dynamics, with downstream changes in disease risk. For this sub-objective, we will conduct field studies to characterize the current activity of vectors, hosts, and pathogens as well as the climatic and environmental predictors most closely associated with their presence and/or activity at a variety of spatial and temporal scales. We will also use historical datasets to gain a comprehensive understanding of how these systems have changed over time and develop predictive models describing how temperature and climatic changes may impact vector and vector-borne pathogen distributions in the near and distant future. Specific studies may examine how abiotic and biotic variables such as temperature, day length, artificial light, land use, vegetation, and stormwater infrastructure, influence the presence/absence, blood feeding behavior, egg-laying, and species composition of arthropod vectors. These studies may also determine how these variables interact with vertebrate host presence, population genetics, behavior, and infection rates.
(2.2) Define physiological, ecological, and behavioral drivers of transmission of vector-borne pathogens of public health importance.
Changing environmental conditions can alter physiological, ecological, and behavioral drivers of transmission of vector-borne pathogens of public health importance. Pathogens, vectors, and hosts are highly responsive to natural environmental variation, to human-induced environmental alterations, and to management interventions, yielding patterns that are often non-linear. Combining and iterating controlled laboratory studies and field studies that query this heterogeneity, therefore, is necessary to interpret and predict patterns of pathogen transmission by vector populations. In this sub-objective, we will complete laboratory and field studies to quantify effects of environmental factors (temperature, humidity, nutrient availability, changes in light:dark cycles), ecological contexts (symbiotic relationships, resource competition, habitat types), and interventions (drugs, pesticides, genetic strategies) on the physiology, behavior, and life histories of vector-host-pathogen interactions. In their studies of diverse vectors, pathogens and hosts, our members will measure vector lifespan, fecundity, circadian activity, host-seeking and feeding behaviors, vector competence, vector genotype x pathogen genotype interactions, and pathogen virulence in the context of variable environmental, ecological and intervention contexts. Further, we will investigate the effects of microbes (human pathogens and environmentally acquired microbiome) on life history traits of vectors that transmit pathogens to vertebrates. Using laboratory assays, field experiments, and field collections from previously established and expanding populations, our group will establish physiological, ecological, and behavioral factors that facilitate vector expansion, adaptation, and pathogen transmission.
(2.3) Evaluate the evolutionary relationships and genetic diversity of vectors and pathogens they transmit.
Vector-borne diseases represent a growing public health concern and are expanding in distribution within the US. The ability of vector-borne pathogens to widen their host range is dependent on genetic adaptations that allow them to infect new vector and host species, while their geographic expansion is linked to the capacity of their vectors to establish into new areas. Thus, pathogen evolution, spread, and transmission dynamics are tightly connected with the evolution and adaptation of their vectors. Likewise, pathogens can influence epigenetic mechanisms, such as DNA methylation, histone modifications, and alter small RNA expression, within vectors (De et al. 2021). These epigenetic modifications can in turn result in nucleotide changes, increasing mutation rates, or variation in gene expression (Ord et al. 2023). In this sub-objective, we will characterize and analyze the genetic diversity of vector and pathogen populations to gain a more complete understanding of their movement patterns, evolution, and overall population structure. In addition, we will explore the co-evolution of pathogenic microorganisms with their vectors, and the molecular determinants of vector-pathogen adaptations. To accomplish these aims, we will deploy Next-Generation Sequencing (NGS) pipelines to sequence pathogen genomes and develop high resolution markers (SNPs, rad-SEQ) to genotype more complex microorganisms or vectors and define epigenetic changes within vector populations (WBGS and Chip-Seq). Genetic data will be analyzed by phylogenetic approaches and population genetic methods in order to understand the origins, movement, population dynamics, and gene flow of emerging vectors and pathogens. Differences in epigenetic markers and small RNA expression will be used to identify areas in the genome and chromatin that correlate with vectorial differences in arthropod populations (e.g., northern versus southern Ixodes scapularis populations). This information will allow us to track patterns of pathogen and vector emergence, spread, and persistence and identify genetic and molecular changes that facilitate the transmission of vector-borne pathogens of public health importance. Newly developed CRISPR/Cas9 technologies allow for the methylation and demethylation of differentially methylated regions (DMRs) in humans. Although this technology is not available in vector studies yet, the identification of DMRs and genetic variants potentially influencing vectorial capacity (such as loci associated with host detection, vector immune responses, and others) can result in targets that can disrupted with these technologies to decrease vector-borne pathogen transmission. Likewise, the identification of genes or loci associated with bacterial or viral pathogenicity can lead to the discovery of targets to knock-out virulence for the development of vaccines, which has been explored with the tick-borne pathogen F. tularensis and Anaplasma phagocytophilum. These studies are expected to unlock translational insights for future vector-borne disease surveillance and control.
Objective 3: Discovery, development, and integration of interventions to manage vector-borne pathogen transmission and pesticide resistance.
(3.1) Screening of natural products for new chemical control tools with novel modes of action (insecticides/acaricides, repellents).
Insecticides presently used for mosquito and tick control are limited in their modes of action. The overuse of these control agents has led to the well-documented evolution of resistance in several mosquito and tick species of relevance to public health. Thus, a broader diversity of chemical control tools with novel modes of action is needed to mitigate resistance and limit transmission of mosquito and tick-borne pathogens. Natural products have been—and continue to be—an important resource and inspiration for developing biologically active chemicals with novel modes of action for controlling insect vectors and pests. First, we will exploit the diverse chemistry of plants and microbes to discover novel active ingredients and formulations for mosquito and tick control. Plant and microbe extracts will be screened for insecticidal, acaricidal, and repellent bioactivities against medically relevant mosquito (e.g., Aedes aegypti) and tick (e.g., Ixodes scapularis) species. Once extracts with potent insecticidal, acaricidal, and/or repellent activities are identified they will be fractionated using GC/MS and LC/MS approaches to identify active compounds. In addition, extracts and active compounds will be tested in conjunction with current control tools (e.g., pyrethroids) to identify potential novel natural product synergists. Thus, our proposed research is likely to lead to highly novel chemical tools for enhancing control of mosquito and tick vectors and their transmission of deadly human pathogens. Second, we will examine the degree of “avoidance proximity” to mosquitoes of plant derived repellents obtained from commercially harvestable plants in the Lamiaceae herb Family. Specifically, we will test GC/MS and LC/MS characterized oil and extracts from various mechanically harvestable cultivars of Nepeta cataria (catnip) with mixtures of mint, basil, and sage against Ae. aegypti and Anopheles quadrimaculatus. Additionally, we will use NGS (RNAseq) to investigate the mode of action of commercially available plant-derived acaricides.
(3.2) Discovery of novel control targets by investigating vector-microbe interactions and molecular/physiological mechanisms.
Reducing transmission of arthropod-borne pathogens can be achieved through limiting vector populations, disruption of acquisition of the pathogen by the arthropod, or disruption of transmission of the pathogen from arthropod to host. Each of these processes are governed by complex molecular, biochemical, physiological, or behavioral mechanisms that are often poorly understood. Accordingly, we aim to identify these mechanisms and their associated components to guide development of strategies for disrupting these processes in arthropod vectors.
First, blood feeding behaviors of arthropods typically occur within the tissues of the host, and so are difficult to impossible to directly observe. Electropenetrography (EPG) has been used for decades to study the probing behaviors of plant feeding insects (Backus et al., 2019). This technique can measure electrical signals associated with probing by passing a current through a wired insect attached to the EPG system through a host during probing. EPG can therefore be used to indirectly measure mouthpart movements, salivation events, and ingestion of fluids that occur when an arthropod vector takes a blood meal. We will use EPG to record electrical waveforms and correlate them with specific feeding behaviors. Subsequent experiments will then use molecular techniques (RNA interference, RNAi) and feeding disruption experiments to identify the specific activities associated with the different waveform types. This data will enhance our understanding of arthropod probing behaviors and allow conceptualization of new strategies for disrupting blood feeding and/or pathogen transmission by arthropod vectors.
Second, basic physiological and biochemical processes govern arthropod vector survival, feeding, and host seeking. A more thorough understanding of these mechanisms will identify novel targets for arthropod vector control. Accordingly, we will use RNAi and CRISPR technology in pharmacological and biochemical studies to explore intercellular communication, excretory physiology, sensory physiology, and seasonal physiology of tick and mosquito vectors. Intercellular communication in insects, particularly through extracellular vesicles (EVs), is poorly understood; however, recent evidence suggests that EVs play important roles in insect immunity, arthropod feeding, RNA interference, and pathogen transmission (Tessetto, et al. 2017, Atayde, et al. 2015, Vora, et al. 2018), and understanding the roles EVs play in arthropod physiology may inform development of new control approaches (Oliva Chavez et al. 2021, Butler et al. 2023). EVs can also be exploited to discover novel vaccine candidates for vaccine development. Similarly, excretory, sensory, and seasonal physiology are vital for arthropod vector survival. These processes rely on specific molecular components to function properly, and these molecular components can be identified using RNAi, CRISPR, and pharmacological techniques, and then the ramifications of limiting the expression or function of these components can be assessed in biochemical and physiological assays. For example, TRPA1 channels are involved in critical physiological processes in some arthropod species including sensing of noxious chemicals and excessive heat. Further characterization of the role of TRPA1 in arthropod vector physiology may show it to be an ideal target for limiting pathogen transmission or vector populations.
(3.3) Develop countermeasures against flavivirus infection and transmission.
Flaviviruses are important human and animal epidemic diseases pathogens, such as the well-known Dengue virus (DENV), West Nile virus (WNV), Zika virus (ZIKV), Japanese encephalitis virus (JEV) and Yellow fever virus (YFV)(Best, SM. 2016). All these five flaviviruses mentioned above also belong to mosquito-borne viruses which can cause dengue-like fever diseases. This objective is to develop small molecule-based drugs for treating Flavivirus infections by targeting viral RNA dependent RNA polymerase (RdRp) which is a key enzyme for viral RNA replication(Barrows et al. 2018). Especially, the novelty of this proposed research is to develop common drugs that can be effective for all or several different flaviviruses based on the finding of a conserved three aspartic acids, D535, D665 and D666 (DDD) motif in the active sites of viral RdRp, which has an electrostatic negative charged binding surface. Therefore, this conserved triple-D motif is an excellent common drug target against flaviviruses.
To accomplish this objective, we will use structure-based approaches and incorporate Artificial Intelligence (AI) to conduct drug design and development. The major methods will include as follows. (1). Building a consensus RdRp structure for virtual compound screening from all five protein sequences of RdRps. Then, conduct In-Silico compound screening (Murgueitio et al. 2012) against the Triple-D motif. (2). In vitro evaluation of the compounds that have activity to inhibit flavivirus infections in a cell-based system (such as Vera E6 cells) in a 96-well plate. Plaque reduction assay and Quantitative RT-PCR (qRT-PCR) will be used for identifying the potent compounds (IC50s) against flavivirus infections. (3). Structure-based design and modification of compounds to increase potencies. (4). Inhibition control in mosquitoes. Because the mosquitoes are the intermediate hosts, the compound inhibition activity in the mosquitoes will be evaluated using the larval-based approach. (5). Pre-clinical evaluation of compounds including In vivo activities, toxicities and pharmacokinetic (PK) studies in mice. (6). Artificial Intelligence (AI) will also be used for drug design and development.
(3.4) Examining the integration of new tools with conventional control, e.g., SIT and Bti.
Initial efforts will focus on surveying for current and potential control tool integrations. Subsequently, one or more of the identified integrations will be selected for additional characterization. We anticipate that initial efforts will be laboratory and field cage based work, and progressing toward field activities. Key goals include the identification of potential synergism and antagonism between the integrated tools, which can affect safety, efficacy, cost and public acceptability.
(3.5) Develop high throughput methods to detect insecticide resistance in Culex vectors of West Nile virus that can be combined with virus surveillance
Insecticide resistance (IR) in mosquitoes can arise from disorganized, misinformed, or otherwise inefficient applications of insecticides. Insecticide resistance management (IRM, Roiz et al. 2018) requires cohesive and productive collaborations among academia and publicly funded mosquito control programs tasked with control. Professional mosquito control programs across the NE US but specifically in NJ, have signaled clear interest in assessing the existence, extent, and effect on their operations of insecticide resistance (IR) in their local mosquito populations. Significant levels of organophosphate and pyrethroid resistance were detected in NJ populations of Culex pipiens and Aedes albopictus (Burtis et al. 2021). However, county superintendents are often unclear what to do with the results, since they often take weeks to months to become available and refer to a small subset of populations. Furthermore, insecticide resistance in some species of potential or realized public health importance is not being examined. For pyrethroid insecticides, currently the go-to class of insecticides for urban mosquito control, IR commonly arises from target site mutations that modify the voltage-gated sodium channels (VGSC) making them refractory to the insecticide. Resistant mosquitoes often “faint” before recovering, which is why IR is called knockdown resistance (kdr) in mosquitoes. Prior work has identified knockdown resistance (kdr) mutation (L1014F) in NJ Cx. pipiens including an homozygote excess indicating strong selection (Johnson and Fonseca 2016). We propose to develop assays to detect point mutations that result in IR to pyrethroids in Cx. pipiens and Cx. restuans, the latter a often-neglected enzootic vector of West Nile virus (WNV). Tests will be optimized so they can be combined with WNV analysis. High infection rates with WNV in disturbed wetland sites with high populations of Cx. restuans suggest Cx. restuans may enable the introduction of WNV to urban environments where both it and Cx. pipiens contribute to transmission, potentiating disease risk (Johnson et al. 2015, 2016). Unlike Cx. pipiens, which is primarily an urban mosquito, Cx. restuans is likely exposed to toxins and insecticides from agricultural practices. Difficulties in correctly differentiating Cx. restuans mosquitoes from Cx. pipiens have left the spatiotemporal mechanisms underlying the epidemiology of West Nile virus (WNV) in the northeastern United States largely unresolved.
Measurement of Progress and Results
Outputs
- Increased capacity to predict vector distribution and density to operationalize better risk assessment (Objs. 1, 2)
- Recommendations for improved vector surveillance practices by local, regional, state and national entities (Obj. 1)
- Parameter estimates connecting vector physiology, behavior, and ecology to the transmission of human and animal pathogens (Obj. 2)
- Epigenomes and sequence variations in vectors of public health importance (Obj. 2)
- EV derived antigens that can be used for vaccine development, qPCR assays to detect IR associated mutations in the voltage-gated sodium channels of Culex pipiens and Cx. restuans (Obj. 3)
- New, natural product-based chemical tools for controlling mosquitoes and ticks (Obj. 3)
- Discovery of novel biochemical targets for guiding new chemical and/or genetic control strategies (Obj. 3)
- Identification of small molecules that can inhibit flavivirus infections (Obj. 3)
Outcomes or Projected Impacts
- An interactive and dependent network of scientific expertise to deal with new mosquito-, tick-, and other vector-borne disease outbreaks.
- Develop, test, implement, and encourage novel control (management) interventions that reduce transmission of human and animal diseases using environmental sound and scientifically-based approaches.
- Identification of novel plant and microbial sources as sources of pesticides and synergists
- Identification of novel biochemical targets and physiological mechanisms in mosquitoes and ticks
Milestones
Projected Participation
View Appendix E: ParticipationOutreach Plan
Several of the universities represented in this multistate group of researchers have formal extension programs while some do not. However, most researchers and all institutions interact closely with health officers, mosquito and tick professionals or the public at large. Many of us conduct a variety of outreach activities and create documents or web-based information.
We have identified four objectives to guide our outreach plan. First, a needs assessment will be conducted to identify key gaps in vector education, training, and evaluation for various audiences. This will involve surveying different stakeholder groups to assess their current knowledge, identify areas requiring more education, and determine preferred methods for receiving information. Focus groups and interviews will also gather qualitative data on specific needs. The findings will inform the development of tailored educational programming, training, and appropriate evaluation metrics. Second, outreach initiatives will promote awareness and understanding of vectors among students and the public. Strategies may include integrating vector biology and prevention into K-12 curriculum through engaging lesson plans, hosting public webinars on emerging vector-borne diseases, and establishing community science projects that empower community members to participate in vector surveillance. Third, specialized educational programming will be developed to build capacity among professionals on the frontlines of vector-borne disease. This includes in-person workshops, short courses, online modules, and field training for veterinarians, public health officials, and clinical health workers. The focus will be on advancing knowledge of vector biology and vector-borne diseases as well as integrated vector management strategies encompassing surveillance, prevention, and control methods. Pre- and post-assessments will evaluate changes in knowledge, attitudes, and competencies. Fourth, we will examine the usefulness of free and fee-for-service tick-testing programs as a conduit to educate the public on vector-borne pathogens, assess the usefulness of different outreach approaches, and increase the public’s familiarity with science and the scientific pursuit.
Additionally, we will hold an annual meeting to promote cooperation among participants, which can lead to enhanced research opportunities. Results of our meetings and activities will be available to all interested parties via the NIMSS website. All publications, both refereed and non-refereed, will be listed on NIMSS. Informational meetings will be held to brief appropriate state and county mosquito control personnel on our progress, particularly via oral presentations at national, regional, and local meetings (e.g., Entomological Society of America, Society for Vector Ecology, American Society for Tropical Medicine and Hygiene, American Mosquito Control Association), regional (Northeastern Mosquito Control Association) that are designed to bring together scientists and mosquito control practitioners.
Organization/Governance
This multistate research project will be established in accordance with the format suggested in the “Manual for Cooperative Regional Research”. One person at each participating institution or agency will be designated, with the approval of the institution’s or agency’s director, as the voting member of the Technical Committee. Other individuals and interested parties are encouraged to participate as non-voting members of the committee. There will be elections of a Chair and a Chair-elect. Officers are to be elected for overlapping three-year terms to provide continuity. Administrative guidance will be provided by an assigned Administrative Advisor and a CSREES Representative.
Note: This proposal has purposefully been written to define the project in broad research terms. This is intended to best position the Multistate Project to cast a broad net to secure an expanded membership, and therefore expanded opportunities for community building and collaboration. First, an expansion of membership will be sought geographically so that the Multistate Project can become a truly national project. Second, the intended expansion will be aimed at securing participants beyond the traditional agricultural experiment station and USDA-ARS mosquito biologists to involve mathematical modelers, community ecologists, and others with special skills regardless of whether they study mosquitoes and/or ticks.
Literature Cited
Atayde VD, Aslan H, Townsend S, Hassani K, Kamhawi S and Olivier M, Exosome secretion by the parasitic protozoan Leishmania within the sand fly midgut. Cell Rep 13: 957-967 (2015).
Backus, E.A., Cervantes, F.A., Guedes, R.N.C., Li, A.Y. and Wayadande, A.C. 2019. AC–DC electropenetrography for in-depth studies of feeding and oviposition behaviors. Annals of the Entomological Society of America. 112, 236–248. https://doi.org/10.1093/aesa/saz009.
Batson, J., Dudas, G., Haas-Stapleton, E., Kistler, A.L., Li, L.M., Logan, P., Ratnasiri, K., Retallack, H. 2021. Single mosquito metatranscriptomics identifies vectors, emerging pathogens and reservoirs in one assay. Elife. 27, 10:e68353.
Beard, C. B., Occi, J., Bonilla, D. L., Egizi, A. M., Fonseca, D. M., Mertins, J. W., … Halperin, W. (2018). Multistate infestation with the exotic disease-vector tick Haemaphysalis longicornis - United States, August 2017-September 2018. MMWR, 67(47), 1310–1313. https://doi.org/10.15585 /mmwr.mm6747a3
Bickerton, M., McSorley, K., Toledo, A., 2021. A life stage-targeted acaricide application approach for the control of Haemaphysalis longicornis. Ticks Tick Borne Dis. 12, 101581. https://doi.org/10.1016/j.ttbdis.2020.101581
Bickerton, M., Toledo, A., 2020. Multiple pruritic tick bites by Asian longhorned tick larvae (Haemaphysalis longicornis). Int. J. Acarol. 46, 373–376. https://doi.org/10.1080/01647954.2020.1805004
Burtis, J. C. et al. NEVBD Pesticide Resistance Monitoring Network: Establishing a Centralized Network to Increase Regional Capacity for Pesticide Resistance Detection and Monitoring. Journal of Medical Entomology 58, 787-797, doi:10.1093/jme/tjaa236 (2021).
Butler LR, Gonzalez J, Pedra JHF, Oliva Chavez AS. 2023. Tick extracellular vesicles in host skin immunity and pathogen transmission. Trends Parasitol. 39(10):873-885. doi: 10.1016/j.pt.2023.07.009.
Coatsworth H, Lippi CA, et al. 2022. A molecular surveillance-guided vector control response to concurrent dengue and West Nile virus outbreaks in a COVID-19 hotspot of Florida. Lancet Reg Health Am. 11:100231. doi: 10.1016/j.lana.2022.100231.
Curtin JM, Aronson NE. 2021. Leishmaniasis in the United States: Emerging Issues in a Region of Low Endemicity. Microorganisms. 9(3):578. doi: 10.3390/microorganisms9030578.
De S, Kitsou C, Sonenshine DE, et al. 2021. Epigenetic Regulation of Tick Biology and Vectorial Capacity. Trends Genet. 37(1):8-11. doi: 10.1016/j.tig.2020.09.012.
Diuk-Wasser, M.A., VanAcker, M.C., Fernandez, M.P. 2021. Impact of Land Use Changes and Habitat Fragmentation on the Eco-epidemiology of Tick-Borne Diseases. J Med Entomol. 58(4):1546-1564.
Eisen L, Eisen RJ. 2023. Changes in the geographic distribution of the blacklegged tick, Ixodes scapularis, in the United States. Ticks Tick Borne Dis. 14(6):102233. doi: 10.1016/j.ttbdis.2023.102233.
Fauver, J.R., Gendernalik, A., Weger-Lucarelli, J., Grubaugh, N.D., Brackney, D.E., Foy, B.D., Ebel, G.D. 2017. The Use of Xenosurveillance to Detect Human Bacteria, Parasites, and Viruses in Mosquito Bloodmeals. Am J Trop Med Hyg. 97(2):324-329.
González, J., Fonseca, D.M., Toledo, A., 2023. Seasonal dynamics of tick species in the ecotone of parks and recreational areas in Middlesex County (New Jersey, USA). Insects 14, 258. https://doi.org/10.3390/insects14030258
Holcomb KM, Khalil N, Cozens DW, Cantoni JL, Brackney DE, Linske MA, Williams SC, Molaei G, Eisen RJ. 2023. Comparison of acarological risk metrics derived from active and passive surveillance and their concordance with tick-borne disease incidence. Ticks Tick Borne Dis. 14(6):102243.
Johnson, B. J., Robson, M. G. & Fonseca, D. M. 2015. Unexpected spatiotemporal abundance of infected Culex restuans suggest a greater role as a West Nile virus vector for this native species. Infect Genet Evol 31, 40-47, doi:10.1016/j.meegid.2015.01.007.
Johnson , B. J. and Fonseca, D. M. 2016. Insecticide resistance alleles in weland and residential populations of the West Nile virus vector Culex pipiens in New Jersey. Pest Manag Sci. 72:481-488.
Kading, C. R., L. W. Cohnstaedt, K. Fall, G. L. Hamer. 2020. Emergence of arboviruses in the United States: The boom and bust of funding, innovation, and capacity. Tropical Medicine and Infectious Disease. 5:E96.
Li, A., Liu, L., Wu, W., Liu, Y., Huang, X., Li, C., Liu, D., Li, J., Wang, S., Li, D., Liang, M., 2021. Molecular evolution and genetic diversity analysis of SFTS virus based on next-generation sequencing. Biosaf. Health 3, 105–115. https://doi.org/10.1016/j.bsheal.2021.02.002
Liu, K., Zhou, H., Sun, R.-X., Yao, H.-W., Li, Y., Wang, L.-P., Mu, D., Li, X.-L., Yang, Y., Gray, G.C., Cui, N., Yin, W.-W., Fang, L.-Q., Yu, H.-J., Cao, W.-C., 2015. A National assessment of the epidemiology of severe fever with thrombocytopenia syndrome, China. Sci. Rep. 5, 9679. https://doi.org/10.1038/srep09679
Luce-Fedrow, A., Mullins, K., Kostik, A.P., St John, H.K., Jiang, J., Richards, A.L. 2015. Strategies for detecting rickettsiae and diagnosing rickettsial diseases. Future Microbiol. 10(4):537-64.
Luo, L.-M., Zhao, L., Wen, H.-L., Zhang, Z.-T., Liu, J.-W., Fang, L.-Z., Xue, Z.-F., Ma, D.-Q., Zhang, X.-S., Ding, S.-J., Lei, X.-Y., Yu, X., 2015. Haemaphysalis longicornis Ticks as reservoir and vector of severe fever with thrombocytopenia syndrome virus in China. Emerg. Infect. Dis. 21, 1770–1776. https://doi.org/10.3201/eid2110.150126
Mechai, S., Bilodeau, G., Lung, O., Roy, M., Steeves, R., Gagne, N., Baird, D., Lapen, D.R., Ludwig, A., Ogden, N.H. 2021. Mosquito Identification From Bulk Samples Using DNA Metabarcoding: a Protocol to Support Mosquito-Borne Disease Surveillance in Canada. J Med Entomol. 58(4):1686-1700.
Oliva Chávez AS, Wang X, Marnin L, Archer NK, Hammond HL, Carroll EEM, Shaw DK, Tully BG, Buskirk AD, Ford SL, Butler LR, Shahi P, Morozova K, Clement CC, Lawres L, Neal AJO, Mamoun CB, Mason KL, Hobbs BE, Scoles GA, Barry EM, Sonenshine DE, Pal U, Valenzuela JG, Sztein MB, Pasetti MF, Levin ML, Kotsyfakis M, Jay SM, Huntley JF, Miller LS, Santambrogio L, Pedra JHF. 2021. Tick extracellular vesicles enable arthropod feeding and promote distinct outcomes of bacterial infection. Nat Commun. 12(1):3696. doi: 10.1038/s41467-021-23900-8.
Ord J, Gossmann TI, Adrian-Kalchhauser I. 2023. High Nucleotide Diversity Accompanies Differential DNA Methylation in Naturally Diverging Populations. Mol Biol Evol. 4;40(4):msad068. doi: 10.1093/molbev/msad068.
Rainey T, Occi JL, Robbins RG, Egizi A. Discovery of Haemaphysalis longicornis (Ixodida: Ixodidae) parasitizing a sheep in New Jersey, United States. J Med Entomol 2018;55:757–9. https://doi.org/10.1093/jme/tjy006
Raney, W.R., Herslebs, E.J., Langohr, I.M., Stone, M.C., Hermance, M.E., 2022a. Horizontal and Vertical Transmission of Powassan Virus by the Invasive Asian Longhorned Tick, Haemaphysalis longicornis, Under Laboratory Conditions. Front. Cell Infect. Microbiol. 12. https://doi.org/10.3389/fcimb.2022.923914
Raney, W.R., Perry, J.B., Hermance, M.E., 2022b. Transovarial Transmission of Heartland Virus by Invasive Asian Longhorned Ticks under Laboratory Conditions. Emerg. Infect. Dis. 28, 726–729. https://doi.org/10.3201/eid2803.210973
Rochlin, I., Egizi, A., Narvaez, Z., Bonilla, D.L., Gallagher, M., Williams, G.M., Rainey, T., Price, D.C., Fonseca, D.M., 2023. Microhabitat modeling of the invasive Asian longhorned tick (Haemaphysalis longicornis) in New Jersey, USA. Ticks Tick Borne Dis. 14, 102126. https://doi.org/10.1016/j.ttbdis.2023.102126
Roiz, D. et al. Integrated Aedes management for the control of Aedes-borne diseases. PLoS neglected tropical diseases 12, e0006845, doi:10.1371/journal.pntd.0006845 (2018).
Schappach, B.L., Krell, R.K., Hornbostel, V.L., Connally, N.P., 2020. Exotic Haemaphysalis longicornis (Acari: Ixodidae) in the United States: Biology, Ecology, and Strategies for Management. J. Integr. Pest Manag. 11. https://doi.org/10.1093/jipm/pmaa019
Staples, J.E., Shankar, M.B., Sejvar, J.J., Meltzer, M.I., Fischer, M. 2014. Initial and long-term costs of patients hospitalized with West Nile virus disease. Am J Trop Med Hyg. 90(3):402-9. doi: 10.4269/ajtmh.13-0206.
Tassetto M, Kunitomi M and Andino R, Circulating immune cells mediate a systemic RNAi-based adaptive antiviral response in Drosophila. Cell 169: 314-325 e313 (2017).
Tufts, D.M., Goodman, L.B., Benedict, M.C., Davis, A.D., VanAcker, M.C., Diuk-Wasser, M. 2021. Association of the invasive Haemaphysalis longicornis tick with vertebrate hosts, other native tick vectors, and tick-borne pathogens in New York City, USA. Int J Parasitol. 51(2-3):149-157.
Tufts, D.M., Sameroff, S., Tagliafierro, T., Jain, K., Oleynik, A., VanAcker, M.C., Diuk-Wasser, M.A., Lipkin, W.I., Tokarz, R. 2020. A metagenomic examination of the pathobiome of the invasive tick species, Haemaphysalis longicornis, collected from a New York City borough, USA. Ticks Tick Borne Dis. 11(6):101516.
Vora A, Zhou W, Londono-Renteria B, Woodson M, Sherman MB, Colpitts TM, Neelakanta G and Sultana H, Arthropod EVs mediate dengue virus transmission through interaction with a tetraspanin domain containing glycoprotein Tsp29Fb. Proc Natl Acad Sci U S A 115: E6604-E6613 (2018).
Wormser, G.P., McKenna, D., Piedmonte, N., Vinci, V., Egizi, A.M., Backenson, B., Falco, R.C., 2020. First Recognized Human Bite in the United States by the Asian Longhorned Tick, Haemaphysalis longicornis. Clinical Infectious Diseases 70, 314–316. https://doi.org/10.1093/cid/ciz449