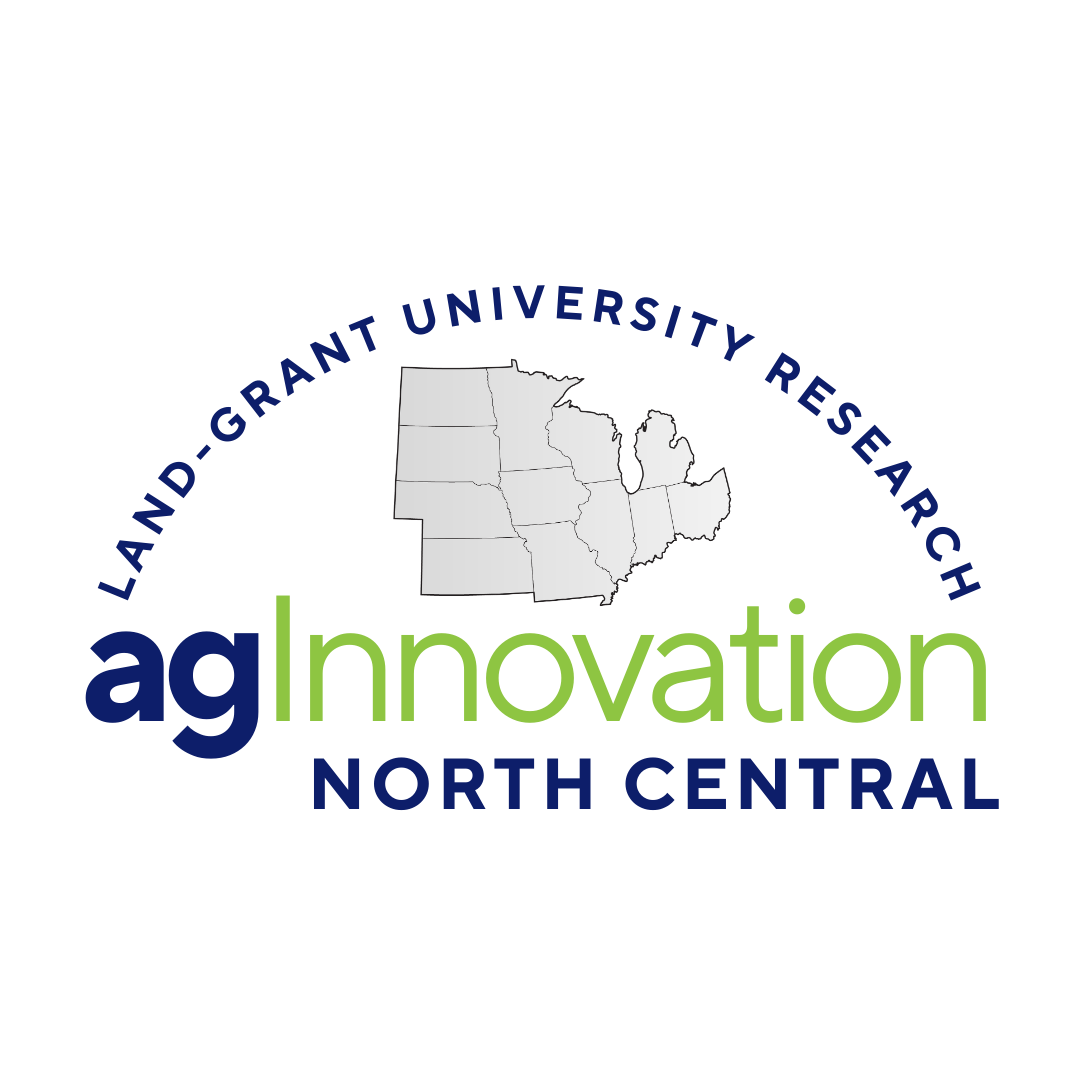
NC1178: Land use and management practice impacts on soil carbon and associated agroecosystems services
(Multistate Research Project)
Status: Active
NC1178: Land use and management practice impacts on soil carbon and associated agroecosystems services
Duration: 10/01/2024 to 09/30/2029
Administrative Advisor(s):
NIFA Reps:
Non-Technical Summary
Soils are important for maintaining crop productivity, and supporting plant, animal and human health. However, intensive agricultural and management practices, conversion of natural lands to production, and adverse weather events and climatic conditions can degrade soil properties and reduce crop production. Therefore, we need to protect and improve the quality of our soils, which requires understanding the positive and negative impacts of existing agricultural practices, understanding when and where to implement more sustainable soil management practices, selecting the best management practices available, and developing and testing new tools. Because soil properties, agricultural practices, climate, and other variables are location-specific, we will conduct this investigation in a standardized manner at multiple regions and locations to inform the decisions of landowners and managers.
Soil organic carbon plays a central role in both responding to changes in soil management and land use decisions, and in translating these decisions into improved soil quality and health, through soil organic carbon’s positive impact on soil physical, chemical, and biological properties. We designed our project to improve our understanding of how soil health changes under intensive management and to optimize decision making related to alternative climate-smart practices to enhance soil health and soil organic stocks. We will study plots managed intensively or using practices that promote soil health and climate resilience, such as cover cropping, reduced till, residue management, and monitor changes to soil carbon and soil health under adverse soil conditions caused by changing weather and climate, specifically drought and excessive wetness.
Statement of Issues and Justification
Soils play important functions in sustaining crop productivity, maintaining plant, animal and human health, and providing various ecosystem services. However, intensive management practices, land use conversion, and adverse climatic conditions can negatively impact soil properties and crop production. Therefore, there is a strong need to protect and improve soil quality, which requires identifying the costs and benefits of existing agricultural practices, understanding the optimal conditions for implementation of more sustainable soil management practices, selecting the best management practices available, and developing and testing new tools. The overall aim of this project is to clarify location-specific and general challenges to maintain soil health under intensive management, evaluate how soil management and land use decisions impact soil health under current and future climate conditions, and inform on practices that enhance soil health through maintaining or increasing soil organic carbon (SOC) stocks, reduction of greenhouse gas emissions, improved productivity and other ecosystem services. Of particular interest are agricultural practices that increase or maintain SOC stocks since SOC is tightly associated with multiple soil health parameters.
Soils are the largest terrestrial carbon pools (Friedlingstein et al., 2019), making soil carbon sequestration a potential, though debated, tool in reducing atmospheric CO2 (Minasmy et al., 2017; Amelung et al., 2020). SOC also impacts multiple soil physical, chemical, and biological parameters and is therefore a cornerstone to various soil functions and ultimately crop productivity. Having been identified as a key soil health indicator, SOC has become a shorthand for soil health (Bagnall et al., 2023). Yet, intensive agricultural practices often (i) accelerate decomposition and loss of existing SOC stocks, (ii) limit the rate of C input, and (iii) reduce the persistence of C inputs. Thus, increasing SOC stocks to improve soil health requires adoption of sustainable soil management practices. Although the mechanisms for SOC change are increasingly understood, our ability to predict it in different ecosystems and under different climate forcing conditions remains limited.
One of the important functions of SOC is promoting aggregation, which facilitates soil water and nutrient holding capacity. In return, soil aggregation can increase SOC storage by physically protecting carbon from mineralization by microbes through encapsulation in smaller pores and by reducing soil erosion (Razafimbelo et al., 2008; Six et al., 2002). SOC dynamics and aggregate (including stability and size distribution) interactions are thus clearly important for microbially driven biogeochemical processes (e.g., greenhouse gas emissions) and climate change mitigation (Blaud et al., 2012; Rillig et al., 2016; Vos et al., 2013; Wang et al., 2019). Soil organic matter (of which ~50% is C) is also critical for soil fertility and plant nutrient availability as it provides an energy source for microbes and serves as a store for nutrients (e.g., N and S) used directly by plants or indirectly following mineralization and cycling by soil microbes. Studies have shown direct relationships between SOC and yield. For example, Oldfield et al. (2022) found positive correlations between soil organic matter and crop yields across 49 farms in Wisconsin and Minnesota. The authors suggested that SOC-driven nutrient availability was partially driving this relationship.
Agricultural management practices have important impacts on soil health through their effects on SOC storage and aggregation. However, long-term pedogenesis processes and short-term economic production objectives can at times conflict with each other. The management decisions in intensive agriculture production largely consider short-term goals based on machinery operation, seed bed preparation, and application of agrochemicals to maximize immediate grain or forage yields. These factors in conjunction with varying seasonal temperatures, water availability, and market demand determine which crop to plant and tillage system to use, and the farm’s potential productivity. However, in the long-term, intensively managed soils negatively impact SOC accrual and soil health (Dalal and Chan, 2001). At the same time, evidence is accumulating that different microbial guilds in the soil also facilitate SOC accumulation, and intensive management techniques appear to undermine the C-accrual process. For example, fertilization reduces plant dependence on mycorrhizal partner fungi, which in turn reduces competition in the soil between symbiotic and saprotrophic fungi, leaving more mineral resources for the latter and ultimately facilitating faster decomposition of SOM (Keiluweit et al. 2015; Pellitier et al. 2021; Carrara et al. 2021, 2022; Noormets et al. 2023). Accelerated decomposition and declining SOC undermine, in turn, the many functions provided by soil aggregation.
A number of climate-smart agriculture practices (e.g., no-tillage, cover cropping, residue retention) facilitate positive soil carbon balance by not only increasing detritus (and thus C) input to the soil, but by maintaining continuous live root crop, also maintain a continuous symbiotic microbial community, which contributes to stabilizing SOC stocks through competitive inhibition of the saprotrophs (Brzostek et al. 2015, Keiluweit et al. 2015, Peršoh et al. 2018). This interaction between plants and different microbial communities appears to be at the heart of the reduced greenhouse gas emissions and SOC accumulation associated with improved soil health (Paustian et al., 2016). The effectiveness of these climate-smart agriculture practices depends on environmental factors and management conditions. However, the high degree of uncertainty in the outcomes is a key limitation to adopting these management practices. This multi-state project provides an excellent opportunity to reduce this uncertainty by advancing our understanding of the agronomic and environmental benefits of climate-smart agriculture practices in diverse cropping systems across the United States, thus informing soil management decisions that enhance soil health and agroecosystem resilience across agroecological zones.
Enhancement of SOC stocks can be achieved through (i) decreasing SOC decomposition resulting from tillage and erosion (Cai et al., 2022), (ii) increasing C inputs through increased photosynthesis or application of exogenous C inputs (Berhane et al., 2020), and (iii) increasing the persistence of existing and new C, through leveraging (1) soil microbial competition as outlined above and (2) the application of C stabilizing amendments such as biochar, both of which modify the relative availability of C and N, and thus suppress SOC priming (Connan et al., 2020; Weng et al., 2022). This project will focus on evaluating the breadth of effects that current soil conservation practices have on SOC dynamics, with the objective of improving the predictive capability of the effects in different production systems and under different climatic conditions. Such practices in cropping systems include, but are not limited to, cover cropping, reduced or no tillage, residue retention, application of C-enriched amendments such as manure, compost, and biochar, and integration of livestock. In forest systems, the SOC response will be described under alternative site preparation, prescribed burning and competition control treatments. On grasslands, improved grazing management practices such as multi-paddock grazing (Kim et al., 2023; Teague & Kreuter, 2020) and regenerative practices such as pasture cropping, which integrates direct seeding of annual crops into dormant perennial grasses (Miller & Badgery, 2009), are found to increase SOC stocks and improve soil health.
Cover crops have become increasingly more widespread as a tool to mitigate soil erosion, retain or increase (with legumes) nutrients and increase carbon inputs (Robačer et al., 2016). Cover cropping has been shown to improve soil aggregate stability, increase SOC content, and improve nutrient utilization efficiency (Blanco-Canqui et al., 2015; Brennan and Acosta-Martinez, 2017), all of which result in less resource inputs with desirable environmental and economic outcomes. In addition, cover crops, either as living plants or dead plant residues, have direct and indirect impacts on soil microbial communities (Brennan and Acosta-Martinez, 2017; Finney et al., 2017). However, the exact mechanism of the effect of cover cropping on SOC dynamics remains incompletely understood. The current project aims to elucidate SOC response to root dynamics, plant-microbe interactions, , biomass production potentials, the quantity and quality of root exudates, the C:N ratio of the biomass and soil medium (Finney et al., 2017; Tiemann et al., 2015), and the taxonomic and functional diversity of the soil biota (e.g., Olson et al., 2014, Poeplau and Don, 2015). .
Diversification in terms of row crop rotation can also result in positive agronomic and ecological outcomes in production systems. Crop rotations affect the quantity and quality of crop residue returned to the soil and thus can influence microbial activities and functions pertinent to nutrient and C cycling (Ashworth et al., 2014; Bremer et al., 2011; Sainju et al., 2014). Based on published literature, annual corn–soybean rotations increase yield compared to their respective monoculture sequences (Mannering and Griffith, 2007; Wilhelm and Wortmann, 2004). However, a three-year rotation of corn-soybean-wheat did not result in yield advantage (Lund et al., 1993). Crop rotation can also reduce the occurrence of weeds, insects, and diseases (e.g., Higgs et al., 1990; Chen et al., 2001; Howard et al., 1998) and alter SOC accumulation (Halvorson and Schlegel., 2012). A study conducted in Tennessee showed that surface C storage can be enhanced by crop sequence diversity in no-till systems after 12 years, whereas subsurface C levels may require more time to be influenced (Ashworth et al., 2014).
A major risk in crop production in the United States is drought. The United States is experiencing increasing seasonal and annual variability in rainfall, which can lead to more frequent soil water deficits during the growing seasons, such as the droughts in 2023. The importance of soil water retention to agronomic productivity cannot be over-emphasized and soil organic matter is a critical determinant of water retention in the root zone (Stewart and Lal, 2018). Increasing soil organic matter can improve water infiltration and enhance water holding capacity by improving soil structure (Hudson, 1994; Franzluebbers, 2002; Lado et al., 2004; Minasny and McBratney, 2018). Soils with higher organic matter can retain more water under vapor pressure deficit, protecting crops from losses induced by drought better than low organic matter soils (Iizumi and Wagai, 2019; Carminati and Javaux, 2020). An increase in soil organic matter by 1% would increase plant available water capacity by 1-3% (Libohova et al., 2018). Every 1% increase in organic matter results in as much as 25,000 gallons of soil water storage per acre. Increasing soil organic matter content may increase tolerance to short-duration drought stress during the growing season. Adhikari et al. (2021) suggested that corn yield during a drought year in Texas could have been explained by differences in soil health. Many areas of the NC are affected by drought, and more frequent storm events. Therefore, building up soil organic matter through improved management practices to maintain soil moisture at levels that enable crops to withstand water deficit is a crucial component of increasing the resilience of agricultural systems and protecting crop yields (Lal, 2020).
An important test of the effectiveness of the climate-smart practices is their interaction with climate change factors like temperature and water availability. Higher mean temperatures and increasing frequency of droughts cause significant global disruptions in agricultural production (Wiebe et al. 2015). Often, though, high temperatures coincide with water limitation and the net effect on crop yield and SOC pools may be difficult to disentangle. However, there are signs that simplistic assumptions about the similarity of productivity and SOC responses to these interacting climate factors may not be justified. For example, Noormets et al. (2021) showed that the simultaneous suppression of carbon assimilation and respiration lead to increased net carbon balance (carbon sequestration) under drought. Furthermore, changes in carbon allocation to different tissues and their chemical composition under changing resource availability and stress levels may expose additional surprises and feedbacks in SOC dynamics (Adamczyk et al. 2019, Augusto and Boča 2022, Carrara et al. 2021, Finzi et al. 2015, Schmidt et al. 2011b).
Rationale
This proposal outlines a project designed to help better understand changes in soil health under intensive management and optimize decision making related to alternative climate-smart practices to enhance soil health and SOC stocks. The project participants have previously shown how soils can be conserved and their quality maintained or improved by timely decisions and adequate soil management interventions. We seek to build on and expand the work of the predecessors of this project over the past four decades of research to design, promote and implement best practices to build more resilient soil and agroecosystems under future climate change scenarios. We view this approach as a natural progression related to the past research of NC-174, NC-1017, and NC-1178. The focus will be on critically evaluating the evidence of land management techniques on different stages of SOC dynamics, and potential modifications to increase SOC stocks and improving soil structure to enhance soil function, reduce greenhouse gas emissions, and simultaneously devise locally relevant mitigation and adaptation strategies to future climatic scenarios. This multi-state project spans researchers from across several agroecological and climatic zones which positions us to generate insights and provide guidance relevant to multiple scenarios, which is a stepping stone for widespread adoption of climate smart practices. Knowledge gained from the proposed research will contribute to a more quantitative understanding of the effects of intensified vs. sustainable agroecosystem management on SOC stocks, soil structural changes, and soil health and ecosystem services over the varying climates and soil landscapes that occur in the participating states.
Related, Current and Previous Work
Previous Accomplishments:
Past and future NC-1178/NC-1017/NC-TEMP-1017/NC-174/NCT-199 research efforts are summarized in Table 1 (see attached). The sequence represents a natural progression from studying soil productivity-erosion relationships to determining C distributions, dynamics and sequestration, and changing management in eroded landscapes.
During the first 5-year phase of the NC-174 project (1983-1988) we identified and documented the effects of erosion on soil properties and corn or small grain yield for research sites located in 11 states (Table 1). Five years of data were collected to better document the effects of weather on the interaction between soil properties and corn yield in the north central United States. First phase achievements included 13 refereed journal articles and chapters in books. Once the database was enlarged, emphasis was placed on selection of management and restoration alternatives at either the initial or a new research site (Table 1, phase 2). The NTRM (Nitrogen, Tillage, Residue and Management) and EPIC (Erosion-Productivity Impact Calculator) models were used in conjunction with the existing data base collected during the initial phase of the NC-174 project to identify factors limiting crop productivity of each soil series investigated. The models were used to evaluate long-term effects of management and restoration alternatives prior to field testing.
The second phase (Table 1) of the project (1988-1993) was to field test the practices selected to maintain or enhance current productivity and to determine the extent to which productivity of eroded soils can be restored. Phase 2 outputs included 25 journal articles and chapters in books.
The third phase (Table 1) of the project (1993-1998) determined threshold soil property values for the restoration of productivity and quality of eroded soils to initial levels. Third phase accomplishments included 74 refereed journal articles and chapters in books.
During the fourth phase (Table 1) of the project, from 1998 to 2003, we examined the erosional and landscape impacts on soil processes and properties as well as assessed the management effects on eroded soil productivity and the quality of soil, air, and water resources. Achievements during phase 4 of the project included 70 refereed journal articles and chapters in books.
In the fifth phase (2004-2009) (Table 1) the main focus was on C distribution and sequestration within the landscape. This focus was integrated with the previous phase by examining the impact of management and erosion on C distribution within the landscape and related to soil quality and productivity. Fifth phase achievements included 84 refereed journal articles and chapters in books. The fifth phase included representation external to the North Central region including participants from Guam and Manitoba.
The sixth phase (2009-2014) (Table 1) builds on previous achievements by examining the role of crop residue removal on factors affecting crop productivity and carbon sequestration. The findings from these studies are being linked to existing studies that relate management and erosion on C distribution within the landscape to soil properties associated with soil quality and productivity. The sixth phase achievements include 70 refereed journal articles and book chapters.
The seventh phase (2014-2019) (Table 1) built on work and achievements from the sixth phase but also included the impacts of utilizing cover crops and perennial crops in intensifying land use in agronomic systems that are being included in biofuels production. This work evaluated the impacts of continuous biomass removal on soil C distribution and soil properties that impact land management and soil erosion on landscapes common to the region. At this time, the seventh phase achievements include 78 refereed journal articles, 1 monograph and 5 book chapters.
The eight phase (2019-2023) (Table 1) built on the seventh and previous phases by including a broader approach to include soil health and productivity and the environmental footprints related to intensified agroecosystems. Data generated by the research team advanced an understanding of how management practices can be optimized to improve soil health and productivity. The resulting research data also demonstrated that the adoption of site-specific management recommendations and system-based conservation is necessary and critical to make agriculture a sound solution to climate change and other environmental issues. Eighth phase achievements include 57 refereed journal articles.
The ninth phase (2025-2029) (Table 1) will build on previous achievements and findings regarding to the effects of soil management on soil health and productivity, but will extend our scope to address the interactions between soil management and climate change phenomena, including developing practices that can improve the agroecosystem resilience in the face of changing climate and extreme climate events.
Related Work:
There is currently only one multistate project that is related to our proposed project. This project is NCERA-059 (Soil Organic Matter: Formation, Function and Management).
NCERA-059 is an unfunded committee that does not coordinate joint research across participants. The objectives of NCERA-059 are broad and diverse. These include: 1) Coordinating research collaborations and information exchange on the biochemistry, biological transformations, and physical/chemical fractions of soil organic matter; 2) Identifying and evaluating indicators that can be used to assess soils as a resource for ecosystems services; 3) Conducting outreach activities to scientists in related disciplines and practitioners to promote the ecological management of soils, including practices that repair or sustain functionally important SOM fractions in both managed and undisturbed systems; 4) Co-sponsoring symposia at national and international meetings; and 5) Interacting with other regional committees. NC-1178 currently has one individual who is also a member of NCERA-059. The two multistate committees have been in communication with each other and have jointly participated in combined meetings in the past.
At the present time, nationally, there is one project that has some relationship to our proposed project - NCCC-211 (Cover crops to improve agricultural sustainability and environmental quality in the upper Midwest). NCCC-211 is an unfunded coordinating committee with focus on the North Central Region. Its objectives include: 1) assess the impact of cover crops on agronomic production and profitability; 2) assess the impact of cover crops on water quality; 3) develop recommendations for plantings of cover crops (rates, timing, application methods) across production systems and across the North Central Region; 4), evaluate the benefit of cover crops as a dual-use crop (i.e. potentially harvested); and , 5) work with state and federal agencies, crop consultants, and seed dealers and other agricultural businesses to assist in implementation and demonstration of successful cover crop management practices. The NCCC-211 Committee also works closely with the Midwest Cover Crops Council. The objectives of our project does not overlap with those of NCCC-211 but rather complements their objectives particularly their Objectives 2, 3 and 4 in that it will evaluate soil and water impacts by cover crops in much greater detail. One of our committee members currently is a participant in NCCC-211. Another project which shares some interests with ours is W18846 (Implementing and Correlating Soil Health Management and Assessment in Western States), however W18846 focuses specifically on soil health assessment and implementation approaches but not on studying and implementing improved management practices.
Other regional projects that could potentially relate to this project are NC-1182 (Management and Environmental Factors Affecting Nitrogen Cycling and Use Efficiency in Forage-Based Livestock Production Systems), NC1195 (Enhancing Nitrogen Utilization in Corn Based Cropping Systems to Increase Yield, Improve Profitability and Minimize Environmental Impacts). However, these projects are narrowly focused on specific crops or crop uses and have only agronomic production practices or conceptual models as their central objective. Neither of these focus on managing the soil to improve ecosystem service and crop production as does our project.
Objectives
-
Evaluate the impact of soil management and land use decisions on soil carbon stocks, soil health, productivity, and other ecosystem services.
Comments: -
Understand and develop resilient soil-scapes for a changing climate and extreme climate events.
Methods
Objective 1. Evaluate the impact of soil management and land use decisions on soil carbon stocks, soil health, productivity, and other ecosystem services
Every state will participate in Objective 1. A minimum of one agroecosystem that is actively managed for enhancing soil health and ecosystem services will be sampled. This site will be compared to an adjacent site representing the least disturbed ecosystem. This will be native grass or timber, depending on the location in the NC and other Multi-State regions. An additional control site will be an intensively managed agroecosystem, e.g., continuous or rotational row crop system, or an intensively managed forest. All treatments will be established, if possible, in a randomized complete block design, with a minimum of three plots (replications) per treatment. SOC stocks will be determined prior to treatment establishment (Olson et al., 2014) and annually thereafter. At least three soil cores should be collected from the center of each plot/treatment to a recommended minimum depth of 1 meter (sampling depth may depend on thickness of soil root zone and presence or absence of carbonates). In the laboratory, the cores will be cut into 5-cm, 15-cm, or 30-cm sections and core bulk density will be determined on each section. Each core section will constitute one soil sample. Cores will be sectioned by depth increments rather than by horizon because it is simpler to later compile the data to calculate C mass in the soil. Horizons are not the same thickness from plot to plot or treatment-to-treatment. Ideally, the first one or two increments from the surface should be 5-cm because of the expected rapidly changing differences due to management practices. Deeper in the profile, the increments will be at least 15-cm. The soils will be air-dried and crushed to pass a 2-mm screen. Coarse fragment mass will be determined as needed. Samples will be finely ground and analyzed for total C using elemental analysis and inorganic carbonates using calcimetry. SOC stocks will then be calculated using the equivalent soil mass approach (Wendt and Hauser, 2013), after accounting for inorganic C and coarse fragments.
Disturbed soil samples will be collected in triplicate from each plot and depth segmented as above to determine particle-size analysis, pH, EC, and available nutrients. Since some SOM pools respond more rapidly to management than total SOM, soils will be fractionated to isolate these pools, specifically the particulate organic matter fraction (POM) and the mineral-associated organic matter (MAOM) pool, and the total C and N in each pool will be determined (Cotrufo et al., 2019). Inorganic C will be quantified if carbonates are present and subtracted from total C to calculate organic C. More elaborate fractionation schemes focusing on aggregate size and particle density can be undertaken to address specific questions (Poeplau et al., 2018). In addition, the chloroform-fumigation-extraction procedure will be performed on soil samples to quantify dissolved and microbial biomass C and N (Vance et al., 1987), as these pools respond rapidly to change and can be useful indicators for SOM cycling. Next, to assess the effect of soil management on its physical properties we will determine, at the very least, aggregate stability using e.g., SLAKES (Flynn et al., 2020), a smartphone application that enables facile image analysis of aggregate slaking. This method has been found to reflect physical traits of soil health and is responsive to management practices (Bagnall et al., 2023). Additional analysis which may be pertinent to local conditions include soil compaction using a penetrometer and saturated hydraulic conductivity.
Greenhouse gas (GHG-CO2, N2O and CH4) emissions rates will be measured following the sampling protocol of GRACEnet Chamber-based Trace Gas Flux Measurement (Parkin and Venterea, 2010). Two PVC rings (30 cm diameter and 10 cm tall) will be installed in each plot to a depth of approximately 6 cm. Flux measurements will be performed by placing vented chambers (30 cm diameter and 10 cm tall) on the PVC rings and collecting gas samples into evacuated glass vials and taken to the lab for analysis, or by in-field continuous measurement by an infrared or gas analyzer. .
Objective 2. Understand and develop resilient soil-scapes for a changing climate and extreme climate events.
All states will participate in Objective 2. This objective will focus on the interactions between climatic conditions and management practices on soil health and productivity. In each participating state, a minimum of one actively managed agroecosystem will be sampled. This site will be compared to an adjacent site representing the least disturbed ecosystem. This will be native grass or timber, depending on the location in the NC and other Multi-State regions. An additional control site will be an intensively managed agroecosystem, e.g., continuous or rotational row crop system, or an intensively managed forest. All treatments will be established, if possible, in a randomized complete block design, with a minimum of three plots (replications) per treatment. Each state will focus on monitoring climatic events that are most relevant to the region, e.g., prolonged drought, extreme precipitation events, prolonged wetness. Controlled experimental plots that simulate drought conditions may be set up using e.g. rain-out shelters. Localized rainfall will be recorded using a data recording tipping bucket rain gauge to monitor precipitation patterns. Soil moisture will be determined using soil water potential or volumetric water content sensors connected to data loggers. Sensors will be installed at root zone depth. Soil water data will be compared across management practice treatments to evaluate their effects on soil water holding capacity, especially in periods of prolonged drought, and soil infiltration after heavy precipitation events. By leveraging the data from neighboring weather stations, the evapotranspiration will be estimated by the Penman-Monteith method and crop water use efficiency will be calculated. Soil sampling and analytical procedures will be conducted as described for objective 1. Changes in SOC pools, GHG emissions, and physical properties determined as for Objective 1 will be evaluated in terms of how management practices mediate the effects of weather and climate events and soil productivity.
Measurement of Progress and Results
Outputs
- Documentation of changes in soil C stocks and surface soil physical properties related to soil management and land use in the NC and other participating regions.
- Identification of SOC pool fractions that respond to and reflect changes in soil management practices.
- Enhanced understanding of climate-landscape processes thus contributing to improved management of soil and water resources.
- Scientific publications, guidebooks, and fact sheets that address the specific benefits of different management practices on soil and crop productivity as well as ecosystem services.
- Organization of workshops and meetings designed to extend information to land managers and policy makers.
Outcomes or Projected Impacts
- Improving our scientific knowledge and understanding concerning the impacts of soil management and land use decisions on soil properties, soil health, and related ecosystem services.
- Better understanding and prediction of the appropriateness of management practices to mitigate potentially disruptive climatic changes.
- Reduction in the uncertainty in the outcomes of adopting changes in soil management in diverse cropping systems across the United States.
- Dissemination of information that can be used by policymakers and land managers to make informed decisions on land use and soil management that apply to crop production and resource conservation.
Milestones
(2024):Site selection/treatment modifications at existing sites(2026):Mid-term Review
(2027):Completion of sample and laboratory analyses
(2028):Workshops and meetings; Begin posting of findings on project website and state agricultural experiment station and extension service web sites.
(2029):Project completion and publication of results. Prepare and submit new proposal for 5-year project renewal.
Projected Participation
View Appendix E: ParticipationOutreach Plan
The multi-state project members are dedicated soil, water and environmental scientists who excel in the communication of their research through different communications outlets, and who are active participants in soil and agricultural research at universities and federal agencies across the country. They also participate in undergraduate and graduate education.
Results will be presented in refereed publications and in posters and symposia at National Meetings of the American Society of Agronomy, Soil Science Society of America, and the Soil and Water Conservation Society. We will also provide the information to collaborating agencies such as the NRCS and various federal, state, and local agencies. Findings will be posted on websites devoted to the transfer of information to the general public including University Departmental and Extension websites and our project website. Additionally, committee members will extend this information at workshops, local field days, field tours, and through preparation of fact sheets. The Committee will also organize a symposium on soil management for climate-resilient agroecosystems at either the SSSA or SWCS annual meetings in 2028 and 2029, respectively.
NC1178 members serve as top-tier, peer-reviewed journals editors and as manuscript reviewers, and therefore, enhance the overall quality of published research. Members also serve the scientific community by their leadership in SSSA divisions and ASA communities, engagement in federal grant review panels, and as reviewers for grant proposals. Results of our multi-state project will be available through the annual project report and periodic project meetings.
Organization/Governance
The Regional Technical Committee will follow the operational procedures listed in the State Agricultural Experiment Directors, CSREES (now known as NIFA) and ESCOP document entitled "Guidelines for Multistate Research Activities" revised and dated April 2002. The voting membership of the Regional Technical Committee includes one representative from each cooperating agricultural experiment station or institution appointed by the director and a representative of each cooperating USDA-ARS research unit or location. The administrative advisor and the CSRS representative are non-voting members. All voting members of the Technical Committee are eligible for office. The offices of the Regional Technical Committee include the Chair, the Vice-Chair and the Secretary. The chair, secretary, and secretary-elect will be elected by committee membership and serve for one year. The secretary will assume the chair position upon the completion of the term of the chair. The secretary-elect will assume the secretary’s position when the vice chair assumes the chair’s position and then the chair’s position. The annual meetings will be hosted by the chair at his/her location or at a location determined by the committee membership.
The duties of the Technical Committee are to coordinate work activities related to the project. The Chair, in accord with the Administrative Advisor, will notify the Technical Committee of the time and place of the meeting and will prepare the agenda and preside at meetings of the Technical Committee and Executive Committee. They will be responsible for preparing the annual progress report and coordinating the preparation of regional reports. The Vice-Chair assists the Chair in all functions and the Secretary records the minutes and performs other duties assigned by the Technical Committee or Administrative Advisor. The Chair appoints subcommittees as needed. Annual meetings will be held by the Technical Committee, as authorized by the Administrative Advisor, for the purpose of conducting business related to the project.
One of the tasks of the Committee is to seek external funding for strengthening research and teaching components. The Committee members will prepare and submit grant proposals for seeking funding support from several sources including USDA(NRI), USEPA, and industry. The focus of the grant proposal(s) will be conducting research on the impact of soil management and land use decisions on: (1) gain and loss of soil C stocks, (2) relation of soil health to soil physical, chemical, and biological soil properties, (3) GHG emissions, (4) resilience under a changing climate.
Literature Cited
- Adamczyk B, Sietiö O-M, Biasi C, Heinonsalo J (2019) Interaction between tannins and fungal necromass stabilizes fungal residues in boreal forest soils. New Phytologist 223 (1):16-21. doi:10.1111/nph.15729
- Adhikari, K., D. R. Smith, H. Collins, R. L. Haney, and J. E. Wolfe. (2021). Corn response to selected soil health indicators in a Texas drought. Ecological Indicators 125:107482.
- Amelung, W. et al. Towards a global-scale soil climate mitigation strategy. Nat Commun 11, 5427 (2020).
- Ashworth, A.J., Allen, F.L., Wight, J.P., Saxton, A.M., Tyler, D.D., and Sams, C.E. (2014). Soil organic carbon sequestration rates under crop sequence diversity, bio-covers, and no-tillage. Soil Science Society of America Journal 78: 1726-1733
- Augusto L, Boča A (2022) Tree functional traits, forest biomass, and tree species diversity interact with site properties to drive forest soil carbon. Nature Communications 13 (1):1097. doi:10.1038/s41467-022-28748-0
- Bagnall, D. K. et al. A minimum suite of soil health indicators for North American agriculture. Soil Security 10, 100084 (2023).
- Berhane, M. et al. Effects of long-term straw return on soil organic carbon storage and sequestration rate in North China upland crops: A meta-analysis. Global Change Biology 26, 2686–2701 (2020).
- Blanco-Canqui, H., Shaver, T.M., Lindquist, J.L., Shapiro, C.A., Elmore, R.W., Francis, C.A., Hergert, G.W., (2015)(. Cover crops and ecosystem services: Insights from studies in temperate soils. Agronomy Journal. doi:10.2134/agronj15.0086
- Blaud, A., Lerch, T.Z., Chevallier, T., Nunan, N., Chenu, C., & Brauman, A. (2012). Dynamics of bacterial communities in relation to soil aggregate formation during the decomposition of 13C-labelled rice straw. Applied Soil Ecology, 53, 1-9.
- Bremer, E., Janzen, H.H., Ellert, B.H., and Mckenzie, R.H. (2011). Carbon, nitrogen, and greenhouse gas balances in an 18-year cropping system study on the northern great plains. Soil Science Society of America Journal 75: 1493-1502.
- Brennan, E.B., Acosta-Martinez, V., 2017. Cover cropping frequency is the main driver of soil microbial changes during six years of organic vegetable production. Soil Biology and Biochemistry. doi:10.1016/j.soilbio.2017.01.014
- Brzostek ER, Dragoni D, Brown ZA, Phillips RP (2015) Mycorrhizal type determines the magnitude and direction of root-induced changes in decomposition in a temperate forest. New Phytologist 206 (4):1274-1282. doi:10.1111/nph.13303
- Cai, A. et al. Declines in soil carbon storage under no tillage can be alleviated in the long run. Geoderma 425, 116028 (2022).
- Carminati, A., Javaux, M., (2020). Soil Rather Than Xylem Vulnerability Controls Stomatal Response to Drought. Trends in Plant Science 25(9), 868-880.
- Carrara JE, Fernandez IJ, Brzostek ER (2022) Mycorrhizal type determines root–microbial responses to nitrogen fertilization and recovery. Biogeochemistry 157 (2):245-258. doi:10.1007/s10533-021-00871-y
- Carrara JE, Walter CA, Freedman ZB, Hostetler AN, Hawkins JS, Fernandez IJ, Brzostek ER (2021) Differences in microbial community response to nitrogen fertilization result in unique enzyme shifts between arbuscular and ectomycorrhizal-dominated soils. Global Change Biology 27 (10):2049-2060. doi:https://doi.org/10.1111/gcb.15523
- Casteel, M. J., M. D. Sobsey, and J. P. Mueller. (2006). Fecal Contamination of Agricultural Soils Before and After Hurricane-Associated Flooding in North Carolina. Journal of Environmental Science and Health, Part A 41:173-184.
- Chen, S., Porter, P.M., Reese, C.D., and Stienstra, W.C. (2001). Crop sequence effects on soybean cyst nematode and soybean and corn yields. Crop Science 41: 1843-1849.
- Coonan, E. C. et al. Microorganisms and nutrient stoichiometry as mediators of soil organic matter dynamics. Nutr Cycl Agroecosyst 117, 273–298 (2020).
- Cotrufo, M.F., Ranalli, M.G., Haddix, M.L. et al. (2019) Soil carbon storage informed by particulate and mineral-associated organic matter. Nat. Geosci. 12, 989–994. https://doi.org/10.1038/s41561-019-0484-6
- Dalal, R. C. & Chan, K. Y. Soil organic matter in rainfed cropping systems of the Australian cereal belt. Soil Res. 39, 435–464 (2001).
- Finney, D.M., Buyer, J.S., Kaye, J.P., (2017). Living cover crops have immediate impacts on soil microbial community structure and function. Journal of Soil and Water Conservation. doi:10.2489/jswc.72.4.361
- Finzi AC, Abramoff RZ, Spiller KS, Brzostek ER, Darby BA, Kramer MA, Phillips RP (2015) Rhizosphere processes are quantitatively important components of terrestrial carbon and nutrient cycles. Global Change Biology 21 (5):2082-2094. doi:10.1111/gcb.12816
- Flynn, K.D., Bagnall, D.K. and Morgan, C.L., (2020). Evaluation of SLAKES, a smartphone application for quantifying aggregate stability, in high‐clay soils. Soil Science Society of America Journal, 84(2), pp.345-353.
- Franzluebbers, A.J., (2002). Water infiltration and soil structure related to organic matter and its stratification with depth. Soil and Tillage Research 66(2), 197-205.
- Friedlingstein, P. et al. Global Carbon Budget (2019). Earth System Science Data 11, 1783–1838 (2019).
- Halvorson, A.D., and Schlegel, A.J. 2012. Crop rotation effect on soil carbon and nitrogen stocks under limited irrigation. Agronomy Journal 104: 1265-1273.
- Hesse, B. D., Gebhardt, T., Hafner, B. D., Hikino, K., Reitsam, A., Gigl, M., Dawid, C., Häberle, K.H., & Grams, T. E. E. (2023). Physiological recovery of tree water relations upon drought release—response of mature beech and spruce after five years of recurrent summer drought. Tree Physiology, 43(4), 522–538.
- Higgs, R.L., Peterson, A.E., and Paulson, W.H. (1990). Crop rotation: Sustainable and profitable. Journal of Soil and Water Conservation 45: 68–70.
- Howard, D.D., Chambers, A.Y., and Lessman, G.M. (1998). Rotation and fertilization effects on corn and soybean yields and soybean cyst nematode populations in a no-tillage system. Agronomy Journal 90: 518–522.
- Hudson, B.D., (1994). Soil organic matter and available water capacity. Journal of Soil and Water Conservation 49(2), 189.
- Iizumi, T., Wagai, R., (2019). Leveraging drought risk reduction for sustainable food, soil and climate via soil organic carbon sequestration. Scientific Reports 9(1), 19744.
- Keiluweit M, Bougoure JJ, Nico PS, Pett-Ridge J, Weber PK, Kleber M (2015) Mineral protection of soil carbon counteracted by root exudates. Nat Clim Change 5 (6):588-595. doi:DOI 10.1038/nclimate2580
- Kim, J., Ale, S., Kreuter, U. P., Teague, W. R., DelGrosso, S. J., & Dowhower, S. L. (2023). Evaluating the impacts of alternative grazing management practices on soil carbon sequestration and soil health indicators. Agriculture, Ecosystems & Environment, 342, 108234.
- Kundel, D., Meyer, S., Birkhofer, H., Fliessbach, A., Mäder, P., Scheu, S., van Kleunen, M., & Birkhofer, K. (2018). Design and Manual to Construct Rainout-Shelters for Climate Change Experiments in Agroecosystems. In Frontiers in Environmental Science (Vol. 6). https://www.frontiersin.org/articles/10.3389/fenvs.2018.00014
- Lado, M., Paz, A., Ben-Hur, M., (2004). Organic Matter and Aggregate Size Interactions in Infiltration, Seal Formation, and Soil Loss. Soil Science Society of America Journal 68(3), 935-942.
- Lal, R. (2004). Soil carbon sequestration impacts on global climate change and food security. Science, 304(5677), 1623-1627.
- Lal, R., (2020). Soil organic matter and water retention. Agronomy Journal 112(5), 3265-3277.
- Libohova, Z., Seybold, C., Wysocki, D., Wills, S., Schoeneberger, P., Williams, C., Lindbo, D., Stott, D., Owens, P.R., (2018). Reevaluating the effects of soil organic matter and other properties on available water-holding capacity using the National Cooperative Soil Survey Characterization Database. Journal of Soil and Water Conservation 73(4), 411.
- Lund, M.G., Carter, P.R., and Oplinger, E.S. (1993). Tillage and crop rotation affect corn, soybean, and winter wheat yields. Journal of Production Agriculture 6: 207–213
- Mannering, J.V., and Griffith, D.P. (2007). Value of crop rotation under various tillage systems. AY-230. Purdue Univ. Coop. Ext. Serv., West Lafayette, IN.
- Mazhar, S., E. Pellegrini, M. Contin, C. Bravo, and M. De Nobili. (2022). Impacts of salinization caused by sea level rise on the biological processes of coastal soils - A review. Frontiers in Environmental Science 10.
- Millar, G. D., & Badgery, W. B. (2009). Pasture cropping: a new approach to integrate crop and livestock farming systems. Animal Production Science, 49(10), 777-787.
- Minasny, B., McBratney, A.B., (2018). Limited effect of organic matter on soil available water capacity. European Journal of Soil Science 69(1), 39-47.
- Noormets, A., Bracho, R., Ward, E., Seiler, J., Strahm, B., Lin, W., McElligott, K., Domec, J.C., Gonzalez‐Benecke, C., Jokela, E.J., Markewitz, D., Meek, C., Miao, G., McNulty, S.G., King, J.S., Samuelson, L., Sun, G., Teskey, R., Vogel, J., Will, R., Yang, J., Martin, T.A. (2021) Heterotrophic Respiration and the Divergence of Productivity and Carbon Sequestration. Geophysical Research Letters 48 (7). doi:10.1029/2020gl092366
- Noormets, A., Miao, G., Kim, D., Ono, M., McNulty, S.G. (2024) Mitigation potential of forests: The integrated plant-microbe system In: McNulty SG (ed.) Future forests. Elsevier. pp: 75-94. doi: 10.1016/B978-0-323-90430-8.00013-7.
- Olson, K., Ebelhar, S.A., and Lang, J.M. (2014). Long-term effects of cover crops on crop yields, soil organic carbon stocks and sequestration. Open Journal of Soil Science 4: 284–292.
- Parkin, T.B. and Venterea, R.T. (2010). Sampling Protocols. Chapter 3. Chamber-Based Trace Gas Flux Measurements. IN Sampling Protocols. R.F. Follett, editor. p. 3-1 to 3-39. Available at: www.ars.usda.gov/research/GRACEnet
- Pellitier PT, Zak DR (2021) Ectomycorrhizal fungal decay traits along a soil nitrogen gradient. New Phytologist 232 (5):2152-2164. doi:https://doi.org/10.1111/nph.17734
- Peršoh D, Stolle N, Brachmann A, Begerow D, Rambold G (2018) Fungal guilds are evenly distributed along a vertical spruce forest soil profile while individual fungi show pronounced niche partitioning. Mycological Progress 17 (8):925-939. doi:10.1007/s11557-018-1405-6
- Poeplau, C., and Don, A. (2015). Carbon sequestration in agricultural soils via cultivation of cover crops: A meta-analysis. Agricultural, Ecosystems, and Environment 200: 33–41.
- Poeplau, C., Don, A., Six, J., Kaiser, M., Nieder, R., Benbi, D., Chenu, C., Cotrufo, F., Derrien, D., Grand, S. and Gregorich, E. (2018). Isolating soil organic carbon fractions with varying turnover rates—a comprehensive comparison of fractionation schemes. Soil Biol. Biochem. 125, 10–26.
- Razafimbelo, T.M., Albrecht, A., Oliver, R., Chevallier, T., Chapuis-Lardy, L., & Feller, C. (2008). Aggregate associated-C and physical protection in a tropical clayey soil under Malagasy conventional and no-tillage systems. Soil and Tillage Research, 98(2), 140-149.
- Rillig, M.C., Lehmann, A., Aguilar-Trigueros, C.A., Antonovics, J., Caruso, T., Hempel, S., Lehmann, J., Valyi, K., Verbruggen, E., Veresoglou, S.D., & Powell, J.R. (2016). Soil microbes and community coalescence. Pedobiologia, 59(1), 37-40.
- Robačer, M., Canali, S., Kristensen, H.L., Bavec, F., Mlakar, S.G., Jakop, M., Bavec, M., (2016). Cover crops in organic field vegetable production. Scientia Horticulturae 208, 104–110. doi:10.1016/J.SCIENTA.2015.12.029
- Sainju, U.M., Barsotti, J.L., and Wang, J. (2014). Net global warming potential and greenhouse gas intensity affected by cropping sequence and nitrogen fertilization. Soil Science Society of America Journal 78: 248-261.
- Schmidt MW, Torn MS, Abiven S, Dittmar T, Guggenberger G, Janssens IA, Kleber M, Kogel-Knabner I, Lehmann J, Manning DA, Nannipieri P, Rasse DP, Weiner S, Trumbore SE (2011) Persistence of soil organic matter as an ecosystem property. Nature 478 (7367):49-56. doi:10.1038/nature10386
- Six, J., Conant, R.T., Paul, E.A., & Paustian, K. (2002). Stabilization mechanisms of soil organic matter: Implications for C-saturation of soils. Plant and Soil, 241(2), 155-176.
- Sjøgaard, K. S., T. B. Valdemarsen, and A. H. Treusch. (2018). Responses of an Agricultural Soil Microbiome to Flooding with Seawater after Managed Coastal Realignment. Microorganisms 6:12.
- Teague, R., & Kreuter, U. (2020). Managing grazing to restore soil health, ecosystem function, and ecosystem services. Frontiers in Sustainable Food Systems, 157.
- Tiemann, L.K., Grandy, A.S., Atkinson, E.E., Marin-Spiotta, E., Mcdaniel, M.D., (2015). Crop rotational diversity enhances belowground communities and functions in an agroecosystem. Ecology Letters. doi:10.1111/ele.12453
- Vance, E.D., Brookes, P.C. and Jenkinson, D.S., (1987). An extraction method for measuring soil microbial biomass C. Soil biology and Biochemistry, 19(6), pp.703-707.
- Vos, M., Wolf, A.B., Jennings, S.J., & Kowalchuk, G.A. (2013). Micro-scale determinants of bacterial diversity in soil. FEMS Microbiology Reviews, 37(6), 936-954.
- Wang, B., Brewer, P.E., Shugart, H.H., Lerdau, M.T., & Allison, S.D. (2019). Soil aggregates as biogeochemical reactors and implications for soil-atmosphere exchange of greenhouse gases-A concept. Global Change Biology, 25(2), 373-385.
- Wiebe, K., H. Lotze-Campen, R. Sands, A. Tabeau, D. van der Mensbrugghe, A. Biewald, B. Bodirsky, S. Islam, A. Kavallari, D. Mason-D’Croz, C. Müller, A. Popp, R. Robertson, S. Robinson, H. van Meijl, and D. Willenbockel. (2015). Climate change impacts on agriculture in 2050 under a range of plausible socioeconomic and emissions scenarios. Environmental Research Letters 10:085010.
- Wilhelm, W.W., and Wortmann, C.S. (2004). Tillage and rotation interactions for corn and soybean grain yield as affected by precipitation and temperature. Agronomy Journal 96: 425-432.
- Wendt, J. W., & Hauser, S. (2013). An equivalent soil mass procedure for monitoring soil organic carbon in multiple soil layers. European Journal of Soil Science, 64(1), 58-65.
- Weng Z, Van Zwieten L, Tavakkoli E, Rose MT, Singh BP, Joseph S, Macdonald LM, Kimber S, Morris S, Rose TJ, Archanjo BS (2022). Microspectroscopic visualization of how biochar lifts the soil organic carbon ceiling. Nat Commun 13, 5177.