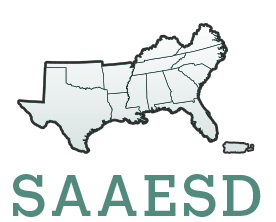
S1092: Biology, ecology, and management of emerging nematode threats in the Southern United States.
(Multistate Research Project)
Status: Active
S1092: Biology, ecology, and management of emerging nematode threats in the Southern United States.
Duration: 10/01/2022 to 09/30/2027
Administrative Advisor(s):
NIFA Reps:
Non-Technical Summary
Statement of Issues and Justification
Climate change, drought, increase production costs and chemical resistant weeds/pathogens challenges agricultural production each year. In addition, customers are demanding accountability and more sustainable, environmentally conscious agricultural practices. To accomplish more efficient food and fiber production, disease management cannot be overlooked. Plant-parasitic nematodes (PPN) causes on average 10% losses (USDA, 2020). Not only do PPN causes yield and economical losses, but they are also a venue used by secondary pathogen to cause disease, resulting in greater losses. The report, dissemination and increase in incidence of new nematodes may threat the production of many crops in the USA.
The research in this proposal addresses important agricultural crop management issues as identified by the REE Advisory Board. In the Report on Agriculture Research Needs of Commodity Crops, the REE Advisory Board states that sustainable, efficient production of these staples (including cotton, soybean, and peanut) is critical to the US food supply and economy, and that “productive efficiency” must be a top priority in order to meet the demands of a growing population in the US and the world. Movement of agricultural goods across the nation and internationally has brought new challenges. The dissemination, dispersal, and movement of new PPN can represent a limitation to major agricultural production in the nation. Further, climate change is likely to affect the distribution of nematode species, as warmer weather allows nematodes to infect crops earlier in the season causing greater damage.
Nematode species such as Heterodera glycines, Meloidogyne hapla, M. floridensis and M. enterolobii have been reported in the last decade in new crops and locations (Rutter et al., 2019; Westphal et al., 2019; Ye et al., 2013; 2019). Emerging nematode species can spread to new geographical regions by self-dispersal, natural and human-assisted dispersal (Jones, 1980; Lehman, 1994). The microscopic size, rapid life cycle, high fecundity, broad host range of some species, and development of non-descript foliar symptoms allow for movement of PPNs to frequently go unnoticed until population establishment is well advanced (Ferris et al., 2003; Singh et al., 2013). Several plant disease management strategies may be considered for limiting dissemination of PPNs to new geographic areas, discouraging their establishment, or reducing endemic populations. These strategies include exclusion, containment and eradication, and management of established populations (Ferris et al., 2003; Barker, 1997). Robust and successful nematode management programs frequently incorporate more than one tactics from several control strategies (Bridge, 1996; Barker and Koenning, 1998; Verdejo-Lucas and McKenry, 2004). Cultural practices, the use of resistant varieties and chemical control can be combined to manage nematodes.
Increasingly, there are emerging threatens to crop production from PPN damage. These emerging threats result from geographic range expansion of nematodes—including invasive nematodes—, infestation of endemic nematodes on new crops due to nematode adaptation or introduction of new crops, and break-down of traditional management tactics due to loss of management products or nematode adaptation. Examples of emerging nematode threats in each category follow. In the case of invasive nematodes, Meloidogyne enterolobii (M.e.) is a critical concern. Meloidogyne enterolobii was discovered in China (Yang and Eisenback, 1983), earned its name of "guava root-knot nematode" by devastating guava orchards in Brazil (Gomes et al., 2012), and was discovered in Florida in 2001 (Brito et al., 2004). Since that time, it has spread to other states in the Southeast including North Carolina in 2011, and Louisiana and South Carolina in 2018 (Rutter, Skantar et al., 2019; Ye et al., 2013). M.e. is of great concern because it is more aggressive—symptoms may be more severe and damage potential is greater—than other root-knot nematodes (Brito et al., 2004; Gomes et al., 2012). Additionally, it can reproduce on many cultivars with resistance to root-knot nematodes, a primary management tactic for root-knot nematodes (Kiewnick et al., 2009; Rutter, Wadl et al., 2019). Southern farmers face substantial risk from this nematode due to direct damage to crops and related economic pressures due to quarantines. A more mature example of nematode range expansion is the reniform nematode (Rotylenchulus reniformis). Reniform nematode was detected in the continental US in the 1940s. Since then, it spread to Mississippi and South Carolina in the 1960s, Arkansas and North Carolina in the 1980s, and is now the most limiting nematode pest in US cotton production (Robinson, 2007).
Nematode populations have changed over time, such is the case of endemic nematodes reported in new crops, and emerging nematode in new geographical areas due to change in management practices. An example of an endemic nematode affecting a new crop is the sting nematode (Belonolaimus longicaudatus) affecting peanut production in Florida and Georgia. Sting nematode is highly damaging to a wide range of crops, but until recently it was not known to affect peanut, severely reducing shoot and root growth to the point of killing plants. In 2015, severe damage in commercial peanut fields in Levy County, Florida due to sting nematode was reported (Kutsuwa et al., 2015). An example of emerging nematode problems due to changing management practices or crop production is the southern root-knot nematode (Meloidogyne incognita, SRKN) in Mid-South states like Arkansas. In Arkansas, SRKN was of little importance in the 1980 as it was only detected in an average of 3.2% of samples received from 1978-1986 to the nematode diagnostic lab, compared to 36% in a more recent survey (Robbins et al., 1987; Kirkpatrick and Sullivan, 2018). An increase in SRKN-susceptible soybean cultivars and the addition of corn into Mid-South cropping system are two important factors that have made southern root-knot nematode an emerging threat there.
Related, Current and Previous Work
There are currently three other multistate research projects that focus on plant parasitic nematodes: NC1197, NE1040, and W3186. These multistate projects differ in the crops of primary interest, the specific nematode species investigated, and the research approaches used and/or specific goals sought. NC1197 focus on corn and soybean in the North Central Region, while NE1040 focus on horticultural crops in the North East region.
For both emerging and well-established nematode pests, management relies primarily on cultural control such as crop rotation, pesticide application (nematicides), use of resistant cultivars, and biological control (Timper et al., 2001; Faske et al., 2018; Grabau et al., 2019). Whereas the general principles are similar for all nematodes, the efficacy of specific management agents (specific sources of resistance, nematicides, or rotational crops) are unique to each nematode-cropping system combination. Additionally, whenever possible, combinations of these management strategies should be combined for maximum efficacy and these combinations are generally even more unique to a given cropping system. As such, when nematode problems emerge, further research is needed on specific management practices to counteract the emerging threat. Work needed to establish effective management strategies varies between emerging or re-emerging nematode threats. For pests that are re-emerging on crops due to a change in management practice, such as southern root-knot nematode on cotton in the Mid-South, foundational research is in place, but efficacy of new management agents (new nematicides, new cultivars, etc.) is needed. For invasive pests, such as guava root-knot nematode, the efficacy of standard nematode management practices against the nematode is not well-known and much research is needed.
Crop rotation that employs poor or non-hosts of the target plant-parasitic nematode(s) is the primary cultural control strategy employed. In principle, crop rotation is known to be effective, but may require rotation to a poor or non-host for multiple years to be effective on its own (Timper et al., 2001; Holguin et al., 2015). Thus, a primary limitation for use of crop rotation is finding enough economically viable rotation crops that are poor hosts of the target nematodes that growers are willing to employ a long enough rotation to help manage nematodes. For emerging or re-emerging nematode pests that have been established in the southern United States for some time, such as SRKN and reniform nematode, there is extensive research on traditional crop rotations. For example, in row crops, incorporating peanut into rotations with cotton, soybean or corn is a well-established method of managing SRKN (Fortunum and Currin, 1993; Johnson et al., 1998). However, as growers seek additional or more stable revenue streams from new crops, research is needed on the role these new crops may play in management of established nematodes on traditional crops and if these new crops (for example carinata for jet fuel) will be damaged by established nematodes. For invasive pests such as GRKN, much more work is needed to validate effective crop rotations. Prior work suggests peanut is a poor host of GRKN, whereas most vegetables, cotton, and soybean are hosts and would be poor choices (Brito et al., 2004; Gomes et al., 2012; Kiewnick et al., 2009). The host status of GRKN for some common crops in the South, such as corn, has not been published and there have been negligible field validation of potential rotations for managing GRKN. Additionally, recent preliminary reports suggests that host range of GRKN may vary by isolate, particularly for cotton, implying effective rotations may vary by location.
Across most crops, chemical control of nematodes using nematicides is often a primary method of nematode management. Growers often prefer nematicide application to other methods because it offers greater flexibility in that it can be used with elite susceptible cultivars and various crop rotations (including monoculture). Nematicide application often has efficacy against a wide range of nematode species (Faske and Hurd, 2015; Morris et al., 2015; Norshie et al., 2016), so it requires less knowledge of the nematode pests present. However, cost often limits practical choice of nematicides, particularly in lower value crops, and there is increasing pressure to limit pesticide use to reduce environmental impacts.
In high value horticulture crops (tomatoes, melons, carrots, etc.), most growers rely on fumigation (broad spectrum products that move through soil as a gas) for nematode control as well as control of other pests like weeds and soilborne fungal pathogens (Watson and Desaeger, 2019). In the post methyl-bromide era, growers cannot rely on a single product for management of all these pests, so growers are often left to combine multiple products or weigh the relative pressure of each pest when choosing a chemical management strategy (Noling, 2016; Zasada et al., 2010). The fumigant 1,3-dichloroprene is among the best nematode management products currently available, but has little efficacy against weeds or pathogens (Grabau et al., 2019; Locascio et al., 1997; Noling, 2016). Combinations with other fumigants such as Chloropicrin and Metam are often used, however efficacy is poor or inconsistent (Desaeger et al., 2008; Gilreath et al., 2004; Locascio et al., 1997; Noling, 2016).
In addition, non-fumigant (liquid or granular products) nematicides can be effective tools to help with nematode management in high value crops, particularly when supplementing fumigation. Older chemistries such as oxamyl have been tested extensively and are known to have some value for managing plant-parasitic nematodes in high value crops. Newer chemistries became available since 2010’s with more products expected to become available in future years. Fluensulfone has been effective for managing root-knot nematodes in various vegetable crops (Morris et al., 2015; Morris et al., 2016), Globodera pallida and sting nematode on potato (Grabau et al., 2019; Norshie et al., 2016). Fluopyram was originally used as a fungicide, however, nematicidal or nematistatic activity against southern root-knot nematode and reniform nematode has been demonstrated in vitro (Faske and Hurd, 2015; Oka and Saroya, 2019). Fluopyram has managed SRKN, to varying degrees, in greenhouse and field tests in vegetable crops, however it has limited mobility in the soil, so it only provides nematode control in a limited area of soil (Becker et al., 2019; Dahlin et al., 2019). Nematicides have limitations that require testing in specific cropping systems to maximize efficacy. For example, fluensulfone can be phytotoxic depending on the plant, application method, and rate (Morris et al., 2015; Morris et al., 2016).
In lower-value agronomic crops (cotton, peanut, soybean, etc.), fumigants may be effective but at reduced rates compared with horticulture crops (Kinloch and Dickson, 1991; Timper et al., 2012). Non-fumigant nematicides are often applied at planting furrow in a narrow band. Nematicides are often combined with other nematode management practices in agronomic crops. Lastly, many nematicides are applied as seed treatments intended primarily to produce some nematode control under low-moderate pressure and not to control heavy nematode pressure on their own (Beeman et al, 2019).While many of these nematicides have been tested against endemic nematode pests such as southern root-knot nematode and sting nematode, there is minimal published work on invasive nematode pests such as guava root-knot nematode. As discussed above, nematicide efficacy often varies by cropping system, nematode species, and application method, so work on specific emerging nematode threats is needed.
Biological control of nematodes is defined as the reduction of nematode population density through the action of living organisms other than nematode-resistant plant cultivars, which occur naturally or through the manipulation of the environment or the introduction of antagonists (Stirling, 1991). The main antagonists used for nematode biocontrol are fungi and bacteria including nematode-trapping fungi, endoparasitic fungi, cyst and egg parasites, bacteria such as Pasteuria as a hyperparasite of nematodes, predatory and endomopathogenic nematodes and microarthropods, plant growth-promoting rhizobacteria and endophytes (Chen, 2004; Stirling, 2014).
Currently, management using biological control relies on either (1) employing cultural practices such as addition of organic amendments or crop rotation to increase endemic populations of antagonists to nematodes—termed conservation biological control (Timper, 2014), or (2) introducing new biological control agents to a system—inundative biological control. In general, conservation biological control as a nematode management strategy is not employed on a wide scale due to its site-specific nature, and the system-level planning required to ensure that practices disruptive to indigenous antagonists (tillage, pesticides, etc.) are avoided (Timper, 2014; Stirling, 2014). Further research identifying specific conservation biological control strategies that are effective and acceptable to growers in the South would be needed for this strategy to employed effectively against emerging nematode threats.
Inundative biological control is more commonly employed as a number of commercial products with live biological control agents are available such as Bacillus firmus (Bio-Nem-WP/BioSafe) (Agrogreen, Ashdod, Israel) (Keren-Zur et al. 2000), B. amyloliquefaciens strain IN937a and B. subtilis strain GB03 (BioYield) (Gustfson LLC, Plano, TX) (Burkett-Cadena et al. 2008), B. firmus GB-126 (VOTIVOTM) (Bayer CropScience, Raleigh, NC) (Castillo et al. 2013), and Paecilomyces lilacinus strain 251 (MeloCon WG) (Certis, Columbia, MD). Most of these products are formulated as seed treatments, which may provide some degree of nematode management, particularly in situations with low nematode pressure, but are not intended to provide outstanding control in high pressure situations. Therefore, these products are often integrated with other management methods such as chemicals, cultural practices, and different organic amendments, or other biological control organisms, and are expected to reduce the dependence on other management practices. For example, researchers reported that combinations of multiple biological control practices such as combination of the application of a biocontrol agent P. lilacinus with various practices such as soil solarization or the application of the biological B. firmus or the chemical oxamyl (Vydate®) (DuPont, Wilmington, DE) are effective for Meloidogyne spp. management (Anastasiadis et al. 2008). Much of the testing with these products has focused on Meloidogyne incognita, Rotylenchulus reniformis, and Heterodera glycines (Burkett-Cadena et al., 2008; Castillo et al., 2013; Xiang et al., 2017). For inundative biocontrol control strategies, including commercial products, to be successfully incorporated into management of other emerging nematode threats, such as for GRKN, further testing needs to done specifically on those nematodes.
The deployment of host-plant resistance has had some significant strides and unfortunate setbacks, both of which can be related to active programs screening and selecting for resistance to plant-parasitic nematodes. The narrow genetic base of soybean resistance to soybean cyst nematode (SCN) in current elite soybean cultivars is the key challenge facing the development of robust SCN resistance varieties. In addition, because of the continuous use of the same limited source of SCN resistance (RHg1 locus) in 95% of commercial soybean varieties, SCN was able to overcome the resistance and infect the majority of commercially cultivated soybean varieties in several states. Thus, identifying new sources of genetic resistance to the most dispersed and damaging pathogen to soybean production is indeed essential to sustain soybean yield and quality. Recently, new sources of epigenetically controlled genes with great potential to enhance soybean resistance to SCN have been discovered (Rambani et al., 2015; 2020).
Similarly, Peanut remains one of the few crop that is still selected by plant breeders within the university system and because of early work of identifying root-knot nematode-resistance genes in wild species of Arachis and developing a breeding program to incorporate such resistance lead to the release of several cultivars, COAN and NemaTAM that are resistant to M. arenaria (Burow et al., 1996; Simpson and Starr, 2001; Simpson et al., 2003). This led to the availability of a breeding line that has been incorporated into several other advanced, high-yielding cultivars, Tifguard, Georgia 14N, Webb, and TifNV (Holbrook et al., 2008; Simpson et al., 2013; Branch and Brenneman, 2015; Holbrook et al., 2017). However, these root-knot nematode resistant cultivars are susceptible to other emerging nematode threats to peanut production such as sting nematode and lesion nematode (Pratylenchus brachyurus).
In cotton, several southern root-knot nematode breeding lines have been identified (Shepherd et al., 1996; Robinson and Percival, 1997; Robinson et al., 2004; Starr et al., 2011), but these breeding lines have has been slow to transfer into commercially available cultivars. However, given a better understanding of the genes involved in resistance to the southern root-knot nematode (McPherson et al., 2004; Shen et al., 2006; Gutierrez et al., 2010) at least a few cultivars such as Deltapine DP 1558NRB2RF and Phytogen PHY 427 WRF, are marketed as having two genes and a high level of resistance, while PHY 487 WRF and Stoneville ST 4946 GLB2 have one gene and a moderate level of resistance are now available (Faske et al., 2018; Wheeler et al., 2018). In soybean there has been some success in later maturity groups (MG VI-VII) where a few genes for SRKN resistance has been identified, whereas few genes have been identified in the early maturity groups (MG III-IV) that are commonly grown in the Mid-South where SRKN yield-loss can be severe (Luzzi et al., 1987; Anand and Shannon, 1988; Luzzi et al., 1994). Currently, there are a few MG IV cultivars with a moderate level of resistance to the southern root-knot, which is the most common maturity group planted in the Mid-South (Emerson et al., 2018). Of the MG IV, GT Ireane, Pioneer P43A42X, GoSoy 49G16, and Delta Grow DG 4940 have expressed a moderate level of resistance to the southern root-knot nematode. Currently there is a lack of resistant cultivars with high-yield potential, desired herbicide technology and desired maturity group (soybean) or season-type (early- or mid-season in cotton) in the Southern crop production systems. Whereas some cotton germplasm is known to be resistant to GRKN (da Silva et al., 2014), the host status of commercial cotton and soybean cultivars for GRKN is largely unknown.
In regard to the reniform nematode, most of the research and effort has focused on cotton and soybean. Currently, no cotton cultivar is resistant to the reniform nematode (Khanal et al., 2018). Breeding effort have been underway for several years to integrate resistance in closely related species of cotton into upland cotton (G. hirsutum), which is the most widespread cotton type grown in the south. Resistance identified in G. longicalyx was effective at killing the nematode as it began to mature, and was developed into the breeding line LONREN. However, unfortunately these breeding lines were severely stunted (hypersensitive response) when screened in areas with a high population density of reniform nematode (Bell et al., 2009; Bell et al., 2014). Resistance in G. barbadense was used to develop the BARBAREN lines; however, the lack of high levels of nematode resistance and poor agronomic traits across the U.S. cotton belt limited their use for U.S. cotton production (McCarty et al., 2012; McCarty et al., 2013). Some cotton cultivars may have tolerance to the attack of reniform nematode that can yield well in field trials (Stetina et al., 2009). The reniform nematode can reproduce well on soybean and there is a wide variation in host suitability in commercially available soybean cultivars (Robbins et al., 2016; Robbins et al., 2017). Soybean is more tolerant to reniform than cotton, however, growing a susceptible soybean can exasperate the nematode population density for the subsequent cotton crop. Currently, there no information on the susceptibility of commercially available soybean cultivars to the reniform nematode, thus producers are unaware if their cultivars are causing an increase in the reniform numbers in their fields. Thus, there is a need to evaluate soybean germplasm for susceptibility to the reniform nematode and characterize reniform resistant genes in soybean.
Some root-knot nematode-resistant cultivars are available in vegetable crops, but resistance against other nematodes is not available and these resistant cultivars are not effective against all root-knot nematode species or isolates. Additionally, production factors limit adoption of resistant cultivars for some vegetables. Tomatoes and peppers with resistance to the three major root-knot nematodes in the South (M. incognita, M. javanica, and M. arenaria) are available. This resistance is conferred by the Mi-1 gene and N gene in tomato and pepper, respectively (Kiewnick et al., 2009). Neither of these sources of resistance are effective against guava root-knot nematode (Brito et al., 2007; Kiewenick et al., 2009). Sweet potato cultivars that confer a moderate level of resistance to southern root-knot nematode are available and used in the majority of acreage in the South (Yencho et al., 2008). These resistant sweet potato cultivars are not effective against peanut, Javanese, or guava root-knot nematode (Rutter, Wadl et al., 2019). In conclusion, further research is needed to screen current commercial cultivars for resistance to emerging nematode threats and develop new cultivars as needed.
Objectives
-
Understand the fundamental biology and genetics that underlie the evolving nematode threats to agriculture in the Southern region.
-
Investigate the ecological and epidemiological factors contributing to the distribution, dissemination and pathogenicity of developing nematode diseases
-
Develop and evaluate integrated nematode management tactics for emerging nematode diseases.
Methods
1. Understand the fundamental biology and genetics that underlie the evolving nematode threats to agriculture in the Southern region.
Nematode samples will be collected from surveys in multiple states in the Southern region from growers, commercial outlets, state plant diagnosticians and other academic collaborators. Soil and plant samples will be collected from cotton, peanut, sweet potato, corn and soybean arbitrarily selected fields.
Investigations into temperature tolerances and reproductive potential of emerging and re-emerging PPN in the Southern U.S. will begin in the laboratory. Eggs and juveniles of PPN will be exposed to different freezing temperatures (for example 0°C, -5°C, and -10°C). The lowest temperature at which 50% of the exposed population survive (S50) will be quantified, measured as the number of eggs that hatch or the number of J2s showing movement after the treatment. Eggs of emerging and re-emerging PPN species will also be exposed to a range of moderate and elevated temperatures (representative of the climate typically encountered in the Southern U.S., to determine the effects of these temperatures on egg hatching rate. This may lead to key insights into the potential for competition among the species in the field.
Transcriptome and epigenome analysis of plant responses to parasitic nematodes in various accessions and genetic materials will be used in order to identify genetically and epigenetically regulated non-coding and protein-coding genes. Functional assays using gene overexpression and inactivation approaches will be used to validate the putative functions of resistance gene candidates.
2. Investigate the ecological and epidemiological factors contributing to the distribution, dissemination and pathogenicity of developing nematode diseases
Epidemiological factors such as, soil humidity, precipitation, and soil temperature will be recorded in the field.
Knowledge of PPN population densities, the damage they induce, and how they may be influenced by abiotic factors such as soil temperature, soil moisture, and local precipitation may assist in making important management decisions. Spatial analysis is a tool that may be used for investigating introduction events and describing the co-occurrence between PPN taxa or abiotic factors (Cambell and Noe, 1985, Goodell and Ferris, 1980, Pethybridge and Turechek, 2003). Spatial patterning of nematode taxa within the soil will be mapped through intensive sampling. Vegetable and field crop fields with high natural populations of nematodes will be identified in collaboration with county extension agents and grower cooperators. Candidate fields will be intensively sampled at a variety of spatial scales, and nematode populations quantified at each sampling point. Further, data on soil moisture, temperature, and texture will be collected and potential correlation in values with nematode counts will be explored. Data will be analyzed using standard statistical and geostatistical techniques, which is a subset of statistics used to assess spatially dependent variables (Chilès & Delfiner, 2012).
3. Develop and evaluate integrated nematode management tactics for emerging nematode diseases.
Through this multi-state project, integrated nematode management tactics for emerging nematode diseases will be developed and evaluated. Tactics will rely on the well-established core principles of crop rotation, nematicide application, biological control, and resistant cultivars used singly or in combination. The number of researchers coordinating on a specific effort will vary based on the extent of the problem. Since nematode management is employed at the field level, most assessments will be done in field conditions. In some cases preliminary work will be done in laboratory or greenhouse settings before being brought to the field. Specifically, replicated and repeated in vitro screening of nematicides at various rates (old and new chemistry) against emerging nematode pests such as guava root-knot nematode will be conducted. In turn, these screenings will be scaled up to greenhouse trials. These trials will be conducted in randomized complete block design (RCBD), where nematicides are applied to sterilized field soil inoculated with the nematode of interest and tomatoes, or other hosts as appropriate. Nematode populations, root galling, and plant growth will be measured to assess nematicide efficacy. In these greenhouse trials, cross-state cooperation will be employed to share nematode isolates as appropriate and increase the breadth of crops assessed. Other greenhouse tests include screening of cultivars and germplasm—particularly sweet potato—for resistance, host range tests, and rotational tests. These greenhouse tests are particularly to provide preliminary data on M.e. management as there are limited field sites for trials on this nematode.
Additionally, relevant cultivars and germplasm will be screened for novel resistance to the pathogenic nematodes. Genome sequence information will be obtained and employed to design molecular markers to map the resistance to assist the introgression of the identified resistance into elite varieties via MAS (Marker-assisted selection). The genetic resistance will be an important component of the integrated nematode management tactics for the emerging nematode diseases.
Field testing will include replicated small plot trials on University research stations and commercial farms. On the largest scale, researchers throughout the South will coordinate uniform cotton trials testing efficacy of nematicides and resistant cultivars for managing southern root-knot nematode as part of the Beltwide Cotton Nematode Research and Education Committee. For M.e., researchers in states where infested field sites can be located, starting with Florida and North Carolina, will coordinate trials in vegetables and agronomic crops first testing nematicides then expanding to crop rotation, resistant cultivars as they are identified, and combinations of management practices. For field trials, researchers will employ at least 4 replications in randomized designs. Nematode assessments will occur before planting and at midseason (30-60 days after planting as appropriate for the given crop) with additional sampling at harvest as needed. Yield will be collected for all trials. Additional trials in agronomic (cotton, peanut, etc.) and horticulture crops (tomato, potato, etc.) will be coordinated across states to address emerging nematode diseases as needed.
Measurement of Progress and Results
Outputs
- New information about the fundamental biology involved in emerging nematodes.
- Geographical distribution and spread of emerging nematode issues, for example Meloidogyne enterolobii
- Identification and characterization of host epigenetically and genetically regulated resistance genes for more durable resistance and improved variety diversity
- New information of near to release varieties and/or experimental lines with partial to high resistance to nematodes
- New information on new management tactics that integrate multiple approaches.
- Information about epidemiological components that are critical for nematode dispersal, dissemination and biology.
Outcomes or Projected Impacts
- Increase the understanding about nematode biology and critical epidemiological component in order to use the information for a more precise agriculture.
- Use the developed epidemiological models for decision making for farmers.
- Use of biological and epidemiological data to predict economical losses due to nematodes in multiple agricultural systems and states.
- More durable resistance should ultimately result from identification of host genes uniquely responsive to nematode infection and introgression of novel sources of nematode resistance to elite breeding lines.
- Determine more effective, efficient and sustainable management practices. Including new cropping systems, resistant variety selection and pesticide application.
- Decreased crop yield loss due to emerging nematode diseases due to more effective and efficient nematode management practices for these diseases.
- Develop extension and education material with an Integrated Pest Management (IPM) approach.
Milestones
(2023):Obj#1- Elucidate the biology and genetics of Heterodera and Meloidogyne spp. reported in new geographical areas or crops based on survey results in the area. Identify and evaluate plant genetic resistance for parasitic nematodes. Obj#2- Identify critical conditions that influence the dissemination and pathogenicity of developing nematodes. Obj#3- Establish laboratory and greenhouse trials for invasive nematodes, such as GRKN with initial focus on nematicides. Conduct small plot field trials and evaluate plant genetic resistance for more established emerging nematodes. Identify sites for field work on new invasive nematodes such as GRKN.(2024):Obj#1- Determine distributions of Meloidogyne spp. in different states. Functional validation of new genetic and epigenetic resources for nematode resistance. Obj#2- Evaluate the effect of epidemiological factors in the distribution of nematodes. Obj#3- Repeat small plot field trials for more established emerging nematodes. Establish small plot field trials for new invasive nematodes such as GRKN. Expand greenhouse trials on invasive nematodes to cultivar and germplasm screening and host studies.
(2025):Obj#1-. Detail the description of Meloidogyne spp. including species, races, morphotypes and variants. Continue the functional analysis of new genetic and epigenetic resources of nematode resistance. Obj#2- Investigate the ecological factors contributing to nematode establishment and spread. Obj#3- Repeat and expand small plot trials for all nematodes and repeat lab/greenhouse trials as needed and evaluate new genetic resources and varieties. Translate research from initial two years to stakeholders through extension and outreach efforts.
(2026):Obj#1- Finish the biology of Meloidogyne spp. Establish and validate the functions of newly discovered genetic and epigenetic resources of nematode resistance. Obj#2- Develop and validate epidemiological models to use on nematode pathosystems Obj#3- Continue field trials and testing of new breeding lines and varieties. Expand extension efforts as more results are available. Publish results in peer-reviewed journals.
(2027):Obj#1- Provide mechanistic understanding of the biology and generics of nematode infection process and plant resistance. Create detail publications that describe the species. Obj#2- Complete analysis of projects and continue publication in peer- review journals and extension efforts. Obj#3- Complete analysis of projects and continue publication and extension efforts.
Projected Participation
View Appendix E: ParticipationOutreach Plan
Throughout these efforts, researchers will interface with growers and other agricultural professionals through outreach efforts to partner in managing emerging nematode diseases. Surveys and experiments are conducted in collaboration with county agents, IPM agents and farmers. Outreach and extension aims include translating research findings into recommendations for agricultural professionals and engaging in dialogue with agricultural professionals to improve and direct research efforts. Results will be presented and distributed at county ag conferences, extension websites and publications on peer review journals. Presentations and publication will be presented and publish in commodity groups including but not limited to Beltwide Cotton Conferences, Southern Soybean Disease Workers, National Sweet potato Collaborators Annual Meeting, and the Southeastern Fruit and Vegetable expo, American Phytopathological Society and Society of Nematologists. Project leaders and personnel will provide regular updates on research findings on local commodity groups. Social media, blogs, and newsletter will be used to disseminate educational material and recent findings to a mass audience in the national and international community. Basic aspects of this research (i.e., resistance/parasitism gene discovery and characterization) will be published in refereed journals such as the Journal of Nematology, Nematology, Phytopathology, Molecular Plant-Microbe Interactions, Plant Molecular Biology, and PLoS ONE. Applied research will be published on referred journals such as Plant Disease Management Reports, and Plant Health Progress. Results from this project will be used to create workshops focus on training crop consultants, extension personnel and farmers on how to applied multiple management practices. At these events, the results of coordinate efforts will be translated to stakeholders and dialogue will occur.
Organization/Governance
Activities of the project will be coordinated by elected officers serving as Chair, Vice-Chair, and Secretary. Each officer will serve a two year term, with the Vice-Chair automatically moving up to the position of Chair after two years. Additionally, an Objective Coordinator will be appointed for each of the three principle objectives of the project, they will be responsible for summarizing research results for the objective for which they are responsible. Administrative guidance will be provided by an assigned Administrative Advisor and a NIFA Representative. Project members will meet annually to review research progress and to plan and coordinate future efforts. Additionally, annual meetings will be used to review and revise as needed common protocols for screening plant germplasm and nematode populations for virulence. The use of such common protocols will be essential for eventual publication of these data. Many decisions on exchange of germplasm will also be made at the annual meetings. Lastly a project web site will be established to facilitate communication and interim results among the participants.
Literature Cited
Literature Cited
Anand, S. C., and Shannon, J. G. 1988. Registration of 'Avery' soybean. Crop Science 28:1024.
Becker, J.O., Ploeg, A. and Nunez, J.J. 2019. Multi-year field evaluation of fluorinated nematicides against Meloidogyne incognita in carrots. Plant Disease 103:2392-2396.
Bell, A. A., Starr, J. L., Jones, J. E., Lemon, R. G., Nichols, R. L., and Overstreet, C. 2009. Nematode resistance and agronomic performance of LONREN and NEMSTACK line. Proceedings of the Beltwide Cotton Conferences; San Antonio, TX. National Cotton Council, Cordova. Pp. 178.
Bell, A. A., Robinson, A. F., Quintana, J., Dighe, N. D., Menz, M. A., Stelly, D. M., Zheng, X. T., Jones, J. E., Overstreet, C., Burris, E., Cantrell, R. G., and Nichols, R. L. 2014. Registration of LONREN-1 and LONREN-2 germplasm lines of upland cotton resistant to reniform nematode. Journal of Plant Registrations 8:187-190.
Branch, W. D., and Brenneman, T. B. 2015. Registration of ‘Georgia-14N’ Peanut. Journal of Plant Registrations 9:159-161.
Brito, J., T.O. Powers, P.G. Mullin, R.N. Inserra and D.W. Dickson. 2004. Morphological and molecular characterization of Meloidogyne mayaguensis isolates from Florida. Journal of Nematology. 36:232-240.
Burow, Mark D., Simpson, Charles E., Paterson, Andrew H., and Starr, James L. 1996. Identification of peanut (Arachis hypogaea L.) RAPD markers diagnostic of root-knot nematode (Meloidogyne arenaria (Neal) Chitwood) resistance. Molecular Breeding 2:369-379.
Burkett-Cadena M, Kokalis-Burelle N, Lawrence KS, Van Santen E, Kloepper JW. (2008) Suppressiveness of root-knot nematodes mediated by rhizobacteria. Biolological Control 47:55-59.
Castillo JD. (2012) Biocontrol studies of Rotylenchulus reniformis in cotton crops in Alabama. Auburn, Alabama, USA, Auburn University. PhD Dissertation.
Castillo JD, Lawrence KS, Kloepper JW. (2013) Biocontrol of the reniform nematode by Bacillus firmus GB-126 and Paecilomyces lilacinus 251 on cotton. Plant Disease 97:967-76.
Chen SY. (2004) Management with biological methods. In: Schmitt DP, Wrather JA, Riggs RD. (eds) Biology and management of soybean cyst nematode. Marceline, Missouri, USA: Schmitt & Associates of Marceline, pp 207-242.
Chen SY, Dickson DW. (2004) Biological control of nematodes by fungal antagonists. In: Chen ZX, Chen SY, Dickson DW. Nematology: Advances and Perspectives Vol II. Beijing, China: Tsinghua University Press and Wallingford, OX, UK: CAB International, pp 979-1040.
Dahlin, P., Eder, R., Consoli, E., Krauss, J. and Kiewnick, S. 2019. Integrated control of Meloidogyne incognita in tomatoes using fluopyram and Purpureocillium lilacinum strain 251. Crop Protection 124:UNSP 104874.
da Silva, E.H., V.d.S. Mattos, C. Furlaneto, M. Giband, P.A. Vianna Barroso, A.W. Moita, A. Jorge-Junior, V.R. Correa, P. Castagnone-Sereno and Dechechi Gomes Carneiro, Regina Maria. 2014. Genetic variability and virulence of Meloidogyne incognita populations from brazil to resistant cotton genotypes. European Journal of Plant Pathology 139:195-204.
Desaeger, J.A., K.W. Seebold and A.S. Csinos. 2008. Effect of application timing and method on efficacy and phytotoxicity of 1, 3‐D, chloropicrin and metam‐sodium combinations in squash plasticulture. Pest Management Science: Formerly Pesticide Science 64:230-238.
Emerson, M., Brown, K., Faske, T. R., and Kirkpatrick, T. L. 2018. Field performance of several glyphosate-resistant maturity group 4 and 5 soybean cultivars in a root-knot nematode infested field in Arkansas Agricultural Experiment Station Research Series 648: Soybean Research Studies 2016. University of Arkansas. Fayetteville.
Faske, T.R. and Hurd, K. 2015. Sensitivity of Meloidogyne incognita and Rotylenchulus reniformis to fluopyram. Journal of Nematology 47:316-321.
Faske, T. R., Overstreet, C., Lawrence, G., and Kirkpatrick, T. L. 2018. Important plant parasitic nematodes of row crops in Arkansas, Louisiana, and Mississippi. Pp. 393-431 in S. A. Subbotin and J. J. Chitambar, eds. Plant parasitic nematodes in sustainable agriculture of North America, vol. Vol. 2 - Northeastern, Midwestern, and Southern USA. New York: Springer.
Fortunum, B.A. and R.E. Currin. 1993. Crop rotation and nematicide effects on the frequency of Meloidogyne spp in a mixed population. Phytopathology 83:350-355.
Gilreath, J.P., B.M. Santos, P.R. Gilreath, J.P. Jones and J.W. Noling. 2004. Efficacy of 1, 3-dichloropropene plus chloropicrin application methods in combination with pebulate and napropamide in tomato. Crop Protection 23:1187-1191.
Gomes, V.M., R.M. Souza, G. Midorikawa, R. Miller and A.M. Almeida. 2012. Guava decline: Evidence of nationwide incidence in Brazil. Nematropica 42:153-162.
Grabau, Z.J., J.W. Noling and P.A. Navia Gine. 2019. Fluensulfone and 1,3-dichloroprene for plant-parasitic nematode management in potato production. Journal of Nematology 51:UNSP e2019-38.
Gutierrez, O. A., Jenkins, J. N., McCarty, J. C., Wubben, M. J., Hayes, R. W., and Callahan, F. E. 2010. SSR markers closely associated with genes for resistance to root-knot nematodes on chromosomes 11 and 14 of upland cotton. Theoretical and Applied Genetics 121:1323-1337.
Holbrook, C. Corley, Timper, Patricia, Culbreath, Albert K., and Kvien, Craig K. 2008. Registration of ‘Tifguard’ Peanut. Journal of Plant Registrations 2:92-94.
Holbrook, C. Corley, Ozias-Akins, Peggy, Chu, Ye, Culbreath, Albert K., Kvien, Craig K., and Brenneman, Tim B. 2017. Registration of ‘TifNV-High O/L’ Peanut. Journal of Plant Registrations 11:228-230.
Holguin, C.M., P. Gerard, J.D. Mueller, A. Khalilian and P. Agudelo. 2015. Spatial distribution of reniform nematode in cotton as influenced by soil texture and crop rotations. Phytopathology 105:674-683.
Johnson, A.W., N.A. Minton, T.B. Brenneman, J.W. Todd, G.A. Herzog, G.J. Gascho, S.H. Baker and K. Bondari. 1998. Peanut-cotton-rye rotations and soil chemical treatment for managing nematodes and thrips. Journal of Nematology 30:211-225.
Keren-Zur M, Antonov J, Bercovitz A, Feldman K, Husid A, Kenan G, Markov N, Rebhun M. 2000. Bacillus firmus formulations for the safe control of root-knot nematodes. Proceedings of the Brighton Crop Protection Conference: Pests and Diseases, Brighton, UK, pp 47-52.
Khanal, C., McGawley, E. C., Overstreet, C., and Stetina, S. R. 2018. The elusive search for reniform nematode resistance in cotton. Phytopathology 108:532-541.
Kiewnick, S., M. Dessimoz and L. Franck. 2009. Effects of the Mi-1 and the N root-knot nematode-resistance gene on infection and reproduction of Meloidogyne enterolobii on tomato and pepper cultivars. Journal of Nematology 41:134-139.
Kinloch, R. and Dickson, D. 1991. Comparison of winter and spring soil fumigation with 1,3-D for the management of Meloidogyne arenaria on peanut. Journal of Nematology 23:682-685.
Kirkpatrick, T. L., and Sullivan, K. 2018. Incidence, population density, and distribution of soybean nematodes in Arkansas in Arkansas Agricultural Experiment Station Research Series 648: Soybean Research Studies 2016. University of Arkansas. Fayetteville.
Kutsuwa, K., D.W. Dickson, J.A. Brito, A. Jeyaprakash and A. Drew. 2015. Belonolaimus longicaudatus: An emerging pathogen of peanut in Florida. Journal of Nematology. 47:87-96.
Locascio, S.J., J.P. Gilreath, D.W. Dickson, T.A. Kucharek, J.P. Jones and J.W. Noling. 1997. Fumigant alternatives to methyl bromide for polyethylene-mulched. HortScience 32:1208-1211.
Luzzi, B. M., Boerma, H. R., and Hussey, R. S. 1987. Resistance to three species of root-knot nematode in soybean. Crop Science 258-262.
Luzzi, B. M., Boerma, H. R., and Hussey, R. S. 1994. A gene for resistance to the southern root-knot nematode in soybean. Journal of Heredity 86:484-486.
McCarty, J. C., Jenkins, J. N., Wubben, M. J., Hayes, R. W., and LaFoe, J. M. 2012. Registration of three germplasm lines of cotton derived from Gossypium hirsutum L. accession T2468 with moderate resistance to the reniform nematode. Journal of Plant Registrations 6:85-87.
McCarty, J. C., Jenkins, J. N., Wubben, M. J., Gutierrez, O. A., Hayes, R. W., Callahan, F. E., and Deng, D. 2013. Registration of three germplasm lines of cotton derived from Gossypium barbadense L. accession GB713 with resistance to the reniform nematode. Journal of Plant Registrations 7:220-223.
McPherson, M. G., Jenkins, J. N., Watson, C. E. , and McCarty, J. C. 2004. Inheritance of root-knot nematode resistance in M-315 RNR and M78-RNR cotton. Journal of Cotton Science 8:154-161.
Morris, K.A., Langston, D.B., Davis, R.F., Noe, J.P., Dickson, D.W. and Timper, P. 2016. Efficacy of various application methods of fluensulfone for managing root-knot nematodes in vegetables. Journal of Nematology 48:65-71.
Morris, K.A., Langston, D.B., Dickson, D.W., Davis, R.F., Timper, P. and Noe, J.P. 2015. Efficacy of fluensulfone in a tomato-cucumber double cropping system. Journal of Nematology 47:310-315.
Noling, J.W. 2016. Nematode management in strawberries. ENY-031. University of Florida Institute of Food and Agricultural Sciences, Gainesville, FL.
Norshie, P.M., Grove, I.G. and Back, M.A. 2016. Field evaluation of the nematicide fluensulfone for control of the potato cyst nematode Globodera pallida. Pest Management Science 72:2001-2007.
Oka, Y. and Saroya, Y. 2019. Effect of fluensulfone and fluopyram on the mobility and infection of second-stage juveniles of Meloidogyne incognita and M. javanica. Pest Management Science 75:2095-2106.
Rambani A, Pantalone V, Yang S, Rice JH, Song Q, Mazarei M, Arelli PR, Meksem K, Stewart CN, Hewezi T. (2020) Identification of introduced and stably inherited DNA methylation variants in soybean associated with soybean cyst nematode parasitism. New Phytol. 2020 Feb 28. doi: 10.1111/nph.1651.
Rambani, A., Rice, J.H., Liu, J., Lane, T., Ranjan, P., Mazarei, M., Pantalone, V., Stewart, C.N., Staton, M. and Hewezi, T., 2015. The methylome of soybean roots during the compatible interaction with the soybean cyst nematode. Plant Physiology, 168(4), pp.1364-1377.
Robbins, R. T., Riggs, R. D., and Von Steen, D. 1987. Results of annual phytoparasitic nematode surveys of Arkansas soybean fields, 1978-1986. Journal of Nematology 19:50-55.
Robbins, R. T., Chen, P., Shannon, G., Kantartzi, S., Li, Z., Faske, T., Velie, J., Jackson, L., Gbur, E., and Dombek, D. 2016. Reniform nematode reproduction on soybean cultivars and breeding lines in 2015. Proceedings of the Beltwide Cotton Conferences; New Orleans, LA. National Cotton Council, Cordova. Pp. 131-143.
Robbins, R. T., Arelli, P., Chen, P., Shannon, G., Kantartzi, S., Fallen, B., Li, Z., Faske, T., Velie, J., Gbur, E., Dombek, D., and Crippen, D. 2017. Reniform nematode reproduction on soybean cultivars and breeding lines in 2016. Proceedings of the Beltwide Cotton Conferences; Dallas, TX. National Cotton Council, Cordova. Pp. 184-197.
Robinson, A.F. 2007. Reniform in US cotton: When, where, why, and some remedies. Annual Review of Phytopathology 45:263-288.
Robinson, A. F., Bridges, A. C., and Percival, A. E. 2004. New sources of resistance to the reniform (Rotylenchulus reniformis) and root-knot (Meloidogyne incognita) nematode in upland (Gossypium hirsutum) and sea island (G. barbadense) cotton. Journal of Cotton Science 8:191-197.
Robinson, A. F., and Percival, A. E. 1997. Resistance to Meloidogyne incognita race 3 and Rotylenchulus reniformis in wild accession of Gossypium hirsutum and G. barbadense from Mexico. Journal of Nematology 29:746-755.
Rutter, W.B., A.M. Skantar, Z.A. Handoo, J.D. Mueller, S.P. Aultman and P. Agudelo. 2019. Meloidogyne enterolobii found infecting root-knot nematode resistant sweetpotato in South Carolina, United States. Plant Dis. 103:775.
Rutter, W.B., P. Wadl, J.D. Mueller and P.A. Agudelo. 2019. Evaluation of sweetpotato germplasm for resistance to pathotypically diverse isolates of Meloidogyne enterolobii from the southeastern United States. Phytopathology 109:4.
Shen, X., Bacelaere, G. V., Kumar, P., Davis, R. F., May, O. L., and Chee, P. W. 2006. QTL mapping for resistance to root-knot nematode in teh M-120 RNR upland cotton line (Gossypium hirsutum L.) of the Auburn 623 RNR source. Theoretical and Applied Genetics 113:1539-1549.
Shepherd, R. L, McCarty, J. C., Jenkins, J. N., and Parrott, W. L. 1996. Registration of nine cotton germplasm lines resistant to root-knot nematode. Crop Science 36:820.
Simpson, C. E., and Starr, J. L. 2001. Registration of `COAN' Peanut. Crop Science 41:918.
Simpson, C. E., Starr, J. L., Church, G. T., Burow, M. D., and Paterson, A. H. 2003. Registration of 'NemaTAM' peanut. (Registrations Of Cultivars). Crop Science 43:1561.
Simpson, C. E., Starr, J. L., Baring, M. R., Burow, M. D., Cason, J. M., and Wilson, J. N. 2013. Registration of ‘Webb’ Peanut. Journal of Plant Registrations 7:265-268.
Starr, J. L., Smith, C. W., Ripple, K., Zhou, E., Nichols, R. L., and Faske, T. R. 2011. Registration of TAM RKRNR-9 and TAM RKRNR-12 germplasm lines of upland cotton resistant to reinform and root-knot nematodes. Journal of Plant Registrations 5:393-396.
Stetina, S. R., Sciumbato, G. L., Young, L. D., and Blessitt, J. A. 2009. Cotton cultivars evaluated for tolerance to reniform nematode. Plant Health Progress:PHP-RS.
Stirling GR. 1991. Biological control of plant parasitic nematodes. Wallingford, OX, UK: CAB International.
Stirling GR. 2014. Biological control of plant parasitic nematodes, 2nd Edition. Wallingford, OX, UK: CAB International.
Timper, P., Davis, R., Jagdale, G. and Herbert, J. 2012. Resiliency of a nematode community and suppressive service to tillage and nematicide application. Applied Soil Ecology 59:48-59.
Timper, P. 2014. Conserving and enhancing biological control of nematodes. Journal of Nematology 46:75-89.
Watson, T.T. and J.A. Desaeger. 2019. Evaluation of non-fumigant chemical and biological nematicides for strawberry production in Florida. Crop Protection 117:100-107.
Wheeler, T. A., Woodward, J. E., and Walker, N. R. 2018. Plant parasitic nematodes of economic importance in Texas and Oklahoma. Pp. 433-451 in S. Stubbotin and C. J., eds. Plant Parasitic Nematodes in Sustainable Agriculture of North America. Sustainability in Plant and Crop Protection. Cham: Springer.
Xiang N, Lawrence KS, Kloepper JW, Donald PA, McInroy JA, Lawrence GW. 2017. Biological control of Meloidogyne incognita by spore-forming plant growth-promoting rhizobacteria on cotton. Plant Dis.101:774-784.
Yang, B. and Eisenback, J.D. 1983. Meloidogyne enterolobii n. sp. (Meloidogynidae), a root-knot nematode parasitizing pacara earpod tree in China. Journal of Nematology 15: 381-391.
Ye, W.M., S.R. Koenning, K. Zhuo and J.L. Liao. 2013. First report of Meloidogyne enterolobii on cotton and soybean in North Carolina, United States. Plant Dis. 97:1262.
Yencho, G.C., K.V. Pecota, J.R. Schultheis, Z. VanEsbroeck, G.J. Holmes, B.E. Little, A.C. Thornton and V. Truong. 2008. 'Covington' sweetpotato. HortScience 43:1911-1914.
Zasada, I.A., J.M. Halbrendt, N. Kokalis-Burelle, J. LaMondia, M.V. McKenry and J.W. Noling. 2010. Managing nematodes without methyl bromide. Annual Review of Phytopathology, Vol 48 48:311-328.