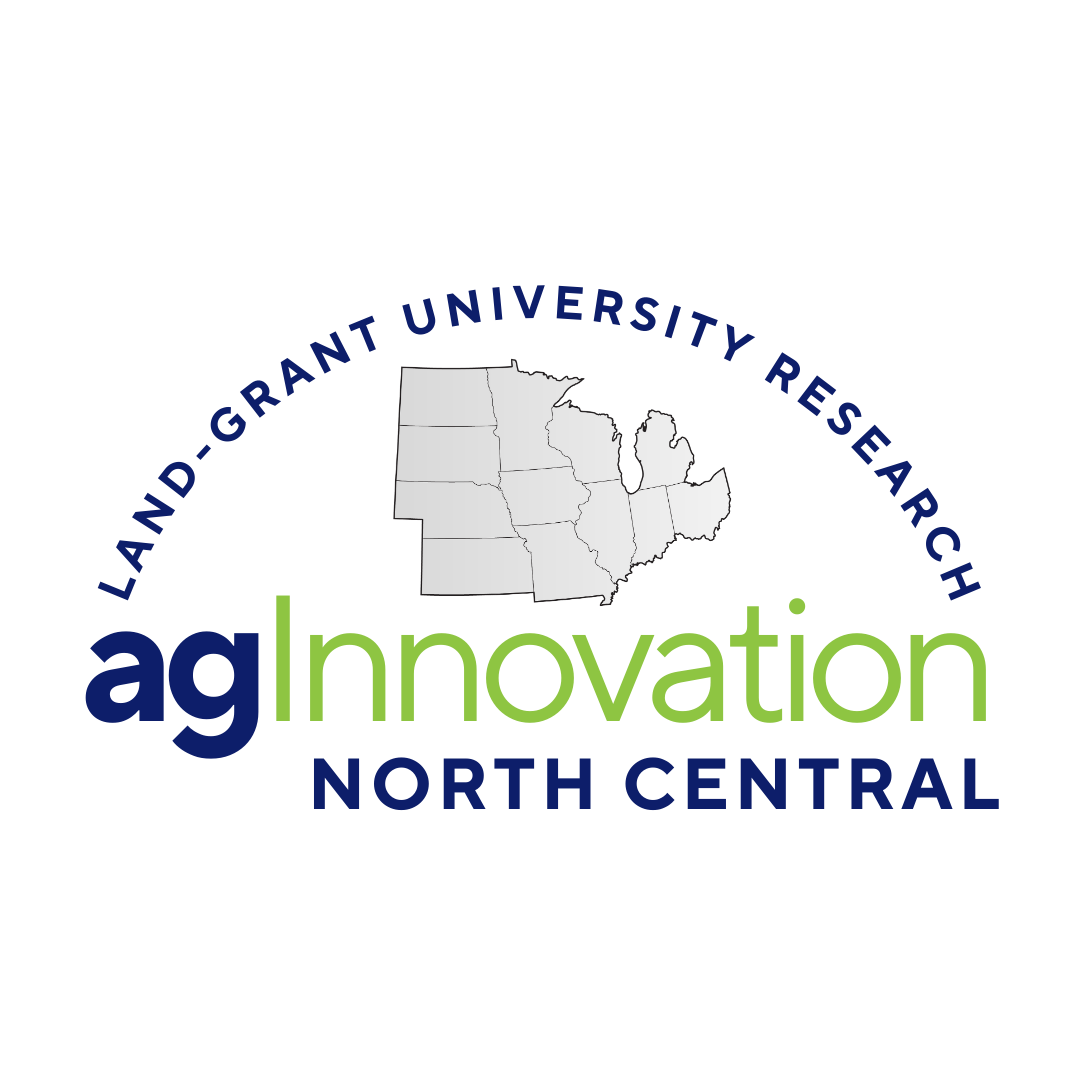
NC1184: Molecular Mechanisms Regulating Skeletal Muscle Growth and Differentiation
(Multistate Research Project)
Status: Active
NC1184: Molecular Mechanisms Regulating Skeletal Muscle Growth and Differentiation
Duration: 10/01/2020 to 09/30/2025
Administrative Advisor(s):
NIFA Reps:
Non-Technical Summary
Statement of Issues and Justification
The overall goal of this cooperative, multi-state, multidisciplinary, research project is to increase the efficiency and sustainability of meat production in the US and across the globe. Based on most predictions, world-wide meat production must increase nearly 50% by 2050 to meet the nutritional needs of a burgeoning world population. Moreover, meat animal production and allied industries contribute some $900 billion to the US economy each year. Meat production is not only necessary to support the health and well-being of a growing global citizenry but also a vibrant driver of the economy. In order to increase the efficiency and sustainability of lean muscle accretion, a more comprehensive understanding of the basic biological mechanisms underlying skeletal muscle accretion is necessary. Moreover, there needs to be technology developed so data-driven decision-making ability can be used to optimize further animal growth efficiency. While we have made great progress towards this goal under the current NC-1184 project, food security remains as one of six grand challenge areas identified by USDA-NIFA facing humankind. Food security or ensuring people across the globe have access to quality food, in this case meat and its importance to the well-being of US is best illustrated in our current pandemic. To that end, greater production efficiencies must be realized in growing meat animals and those mechanisms responsible for controlling muscle tissue growth need to be exploited to achieve this goal. Another grand challage that is addressed, albet to a lesser degree is childhood obesity. Skeletal muscle tissue represents some 40% of the body mass of most humans and as such is responsible for the bulk of glucose disposal by humans on a daily basis. Balancing glucose metabolism against fat deposition requires strict and complex communications between adipose tissue and skeletal muscle, a process heavily investigated by those trying to augment animal growth. At the same time, however, these same mechanisms are at play in the obesity epidemic plaquing a greater and greater number of growing children and adults. The interplay between insulin, glucose uptake and the metabolic syndrome development is at the heart of those mechanisms controlling growth. Therefore, knowledge created by members of NC-1184 are applicable to childhood obesity. Interest in the project continues to grow, suggesting a large number of scientists/investigators and institutions regard discovery activities in the area of muscle growth a viable target for increasing animal productivity. In fact, over 50 scientists claim membership in NC-1184 from some 26 states across the country, many outside the North Central region of the US. Regardless, this heightened level of interest coupled with a rejuvenated membership has spawned a real enthusiasm for understanding the nuances of animal growth and meat production. The goal of NC-1184 project is to utilize molecular and cellular tools to examine the processes that control skeletal muscle growth and function; thereby, providing opportunities to increase the efficiency of lean meat production in animals.
This renewal describes the collaborative effort of some 26 different Agricultural Experiment Stations that will explore the molecular and cellular mechanisms that regulate skeletal muscle growth and function. Major points that support the continuation of this important, fundamental research project for the next five years are:
- Meat is a significant source of protein for human consumption. Meeting the needs of a growing population will require significant growth in meat production world-wide. Though some of this growth will be realized by increasing animal units, greater outputs must be realized per animal unit. This will require a more thorough understanding of those mechanisms responsible for the efficiency of protein accretion.
- In 2012, the meat industry was responsible for nearly 6% of the GDP of the US. Ensuring the viability of this industry in times of elevated energy costs and concerns for the environment will require a better understanding of those inputs, and outputs, required by optimal meat animal production.
- The project continues to relate directly to the national agricultural research priorities set forth in the NIFA Strategic Plan (2014 – 2018) Goal 1: SCIENCE, subgoal 1.1 “Advance our nation’s ability to fight hunger and ensure global food security”. Performance Measures, as outlined in this same Strategic Plan include: 1.1.3 (conferences); 1.1.4 (peer-reviewed publications and 1.1.5 (research). “Improve understanding of fundamental animal physiological processes” (NIFA knowledge area 305) of this Objective is supported by the efforts of this re-written project. Improving the meat animal production efficiency based on a fundamental understanding of mechanisms controlling muscle growth, is directly related to this goal.
- One of the Actionable Strategies of NIFA Goal 1, subgoal 1.7.5 is "Number of graduate students post-doctorates engaged in NIFA-administered projects and programs with an integrated education component to improve educational opportunities in agriculture”. This is an important component of this project. Training undergraduate and graduate students, and postdoctorates in basic fields will relate to long-term improvements in agricultural productivity.
- The NC-1184 Committee continues to be highly productive. Many papers emanating from this group of scientists are published in top tier journals often reserved for reporting fundamental knowledge. This reflects well on the aptitude of the membership and the basic nature of their approach in creation of new knowledge.
- The NC-1184 project is both a multi-state and a multidisciplinary project, involving the effort of investigators at 26 different State Agricultural Experiment Stations. The Principal Investigators represent a variety of basic science disciplines that complement each other and provide the expertise necessary to complete the objectives.
- The project continues to enjoy a strong collaborative environment. During the current project, there have been exchanges of data, scientists, students, samples, cell lines, reagents, etc. In addition, there has been vast knowledge sharing of techniques and on occasion, use of equipment only available at particular stations. The fruits of these collaborations are best illustrated by the number publications jointly authored among the membership. 8. Members of the NC-1184 committee have been and will continue to be highly successful in obtaining outside support to fund this research. Funding from the USDA NRICGP (AFRI) Program, NIH, NSF, health-related granting agencies, and industry sources are used to maintain a high level of productivity.
In summary, this project describes a fundamental research approach to an important agricultural issue, providing the world a high quality source of protein. The current membership has grown substantially and is highly qualified for making immediate and unprecedented progress towards the goals outlined in this revised project proposal.
Related, Current and Previous Work
There are a number of active multistate projects when the USDA multistate data base is searched using the key words: muscle, muscle growth, and muscle growth and development. However, all projects focus on larger, ancillary disciplines, or are predominately focused on the end-product of muscle, or meat. A modest amount overlap that is expected given that muscle represents some 40% of the mass of most animals and forms the basis for meat production. The capacity to of muscle to regenerate using resident stem or stem-like cells makes the tissue ideal for the examination of the global cellular programing and stem cell biology research. Finally, muscle competes with adipose tissue and as such, it is often considered when studying adipose tissue growth and development(function). In fact, a number of the current NC 1184 project members are also members of NCC210 project entitled Regulation of Adipose Tissue Accretion in Meat-Producing Animals. This cross fertilization and multiplicity of projects is highly encouraged and helps foster interdisciplinary, problem solving, cross-institutional efforts.
MYOGENESIS: Muscle fibers are multi-nucleated muscle cells that are created by the fusion of muscle cell precursors or myoblasts (Buckingham, 2001). Furthermore, these myoblasts are derived from mesenchymal cells that migrate from the lateral plate mesoderm to form somites, and early embryonic structure containing myogenic precursors. Postnatal muscle growth is largely predicated on the amount of muscle fibers or cells created during embryonic and fetal stages of development (Dwyer et al., 1994). For example, double muscling, a mutation impacting muscle cell hyperplasia results in animals with extreme muscularity and in some parts of the world, these genetics are exploited to enhance meat production. Furthermmore, muscle development occurs in two waves, at least in most appendicular muscles, where primary muscle fibers form the architecture for a second wave of fiber formation that occurs mid-gestation (Dwyer et al., 1994). Understanding the temporal and spatial cues responsible for controlling this process may provide clues of how we may exploit muscle development as a means of increasing animal productivity and meat production. The Indiana, North Carolina, Ohio, Virginia, Michigan, and Washington Stations have contributed significantly to area by understanding the browning, or changing of myoblasts into fat storing phenotypes. Moreover, the Wyoming and Washington stations have showed the timing of bovine muscle cells during development. The Michigan and Ohio stations have discovered temperature to change myogeneis in developing chicks, which has caused the industry to reconsider temperatures in commercial incubators.
NUCLEOCYTOPLASMIC TRANSPORT: Nucleocytoplasmic transport (NCT) is a process that controls the exchange and cellular distribution of proteins and RNAs between the nucleus and the cytoplasm (Jamali et al., 2011). Several proteins are involved in the regulation of NCT, among which a central player is the nuclear pore complex (NPC). NPCs are composed of more than 30 different nucleoporins. They provide a controlled barrier for the shuttling of large proteins in and out of the nucleus (Hall et al., 2011). The structural integrity of the NPC and their density across the nuclear envelope modulate the efficiency of the NCT. Contrary to dividing cells that refresh their pool of NPCs at every division, postmitotic cells such as myocytes have low rates of synthesis and membrane insertion of new NPCs. Some nucleoporins are recognized as among the longest lived proteins in the cell, and they are never or very slowly replaced once the NPC is formed. It is thus likely that even subtle alterations to the structural stability of the NPC would accumulate over time, leading to late onset defects in the import and export of cargoes across the pore. Indeed, NPCs become increasingly leaky oxidate stress, leading to alteration in nuclear architecture and cell death (D’Angelo e tal., 2009). The role of NCT in muscle cells during development and growth are virtually unknown in meat animals. This area is new to NC-1184.
EXTRACELLULAR MATRIX: Muscle tissues consists predominately of muscle fibers whose primary purpose is to contract and facilitate movement. Yet, the muscle fibers are arranged through the connective tissue framework Connective tissue, and associated cells, are known as extracelluar matrix (ECM). While responsible for the aforementioned, the Ohio Station showed that ECM is also an active participate in the control of muscle growth and development (Velleman et al., 2012). Moreover, the structure of this connective tissue plays a significant role in the overall palatability of this tissue when transformed to meat. One such example of ECM’s profound significance in animal agriculture is in the poultry industry where the ECM is thought to be partially responsible for Wooden Breast Syndrome that results in widespread loss of muscle fibers in chicken breast muscle and subsequent replacement by connective tissue (Velleman, 2015). Identifying key features of how the collective ECM facilitates, maintains and contributes to the overall function and eating pleasure of fresh and processed meat products will dramatically affect to overall productivity of production agriculture. These developments will help the poultry industry select for genetics resistant to wooden breast syndrome.
GROWTH FACTORS: Myogenesis and the subsequent hypertrophy of muscle are regulated by growth factors. These factors are produced by different cell types within the tissue, such as those found in the ECM and may orchestrate growth of cell types located adjacent to the cell. Control of these factors and their mode of action remain a pivotal point to augmenting meat animal growth. One such example of how growth factors modulate animal growth is through steroid-based implants, which have revolutionized beef production in the US. Investigators from the Minnesota and Utah Stations showed these implants change the abundance of insulin like growth factor 1 abundance, which ultimately increases protein deposition in growing cattle (Thornton et al., 2015). Moreover, a number of other growth factors modulate several steps in myogenesis, in vitro and in growing animals (South Dakota, Utah). Another area of great success is the propogation and exploitation of growth modifiers, specifically the beta-adrenergic agonist derivitives, such as but not restricted to Paylean, Optiflix and Zilpaterol. This multistate collectively has had a huge impact on the development and subsequent commercialization of these products. The Illinois Station and Texas Tech have worked with private organizations to identify yet new generations of these promotants. This is just one of several examples where this multistate has contributing to increasing the output and efficieny of animal agriculture. The Washington Station has also found that retinoic acid stimulates muscle development during the early embryonic development, times were dietary interventions are possible in commercial settings. Defining additional biological steps will result in yet further augmentation of animal growth that will increase the production of a highly coveted food, meat.
SATELLITE CELL BIOLOGY: Satellite cells are located between the cell membrane of muscle fibers, just beneath the basal lamina (Mauro, 1961). These undifferentiated cells are restricted to the muscle lineage but are held in a quiescent, non-replicating state until needed for muscle regeneration or growth (Grand and Rudnicki, 2007). Mechanisms controlling their quiescence, activation and subsequent replication and fusion into growing muscle fibers (cells) are quintessential to animal agriculture, as incorporation of these cells are considered a rate-limiting step to adequate DNA for expanded gene expression requisite to muscle cell hypertrophy. Niche and systemic factors include self-renewal signals (Notch), mitogens (FGF2), metabolic factors (IGF-1) and differentiation suppressors (TGF-β). The TGF-β superfamily of growth factors, which includes myostatin (MSTN), initiates signals through the canonical SMAD2/3 pathway and secondary signaling axes that lead to phosphorylation and activation of JNK, p38 and ERK1/2. An incomplete picture exists for the effects of TGF-β-mediated signals on the continuum of satellite cell myogenesis from quiescence through proliferation and differentiation. Identifying those genes, growth factors and nutritional cues that augment satellite cell performance in skeletal muscle will lead to enhanced lean tissue accretion (Allen and Boxhorn, 1989). Investigators from the Indiana, Minnesota, Ohio, Virginia, Kansas, South Dakota, Wyoming, Washington, Indiana, Idaho, Michigan and the North Carolina Stations have taken the aforemented mechanisms that were largely based on rodent data and translated and validated their existence in cattle, sheep, pigs, chickens, turkeys and horses. Defining the existence of these critical mechanisms, or lack thereof, translates the aforementioned discoveries into agriculturally relevant species, a necessary step to exploiting these pathways to improve animal production.
NUTRIENT AND ENERGY SENSORS: Muscle cells, like all cells monitor nutrient availability in their respective niches. Understanding how cells sense these changes may lead to development of different management strategies for optimizing animal growth efficiency, or possibly how to overcome adverse stressors to the homeostatic control of the tissue. One such factor in skeletal muscle is AMP kinase (AMPK). Once activated by AMP, this kinase activates mitochondrial biogenesis in an effort to respond to a decrease in energy availability (Garcia et al., 2008). At the same time, activated AMPK blunts energy consuming pathways in an attempt to conserve energy. One such anabolic pathway curtailed is protein synthesis, the foundation of meat animal growth. Defining the exact role of how muscle cells respond to changes in energy availability will aid development of more effective feeding regimes, either in times nutrient excess or scarcity (Scheffler and Gerrard, 2016). Another emerging, nutrient sensing pathway is known as the O-linked alpha-D-N-acetylglucosamine (O-GlcNAc). O-GlcNAc is cycled on and off various proteins depending on the collective inputs from the hexosamine biosynthetic pathway (Hart and Akimoto, 2009). Exploring how this pathway modulates muscle growth will help us better understand how animal growth may be enhanced. The Virginia, Florida and Wyoming Stations have exploited the natural occurring AMPK mutation in pigs (RN) to understand better the role energy metabolism and “energy charge have on protein synthesis.
MUSCLE PROTEIN ACCRETION: Muscle protein accretion is the difference between muscle protein synthesis and degradation in the tissue. Protein synthesis involves a myriad of steps including the aforementioned stimulation by extracelluar growth factors and (or) other hormones, and the subsequent signal transduction signaling cascades that may involve mammalian target of rapamycin (mTOR) and other associated kinases (Gordon et al., 2013). Protein degradation is equally complex although poorly understood. Targeting individual proteins within this highly organized and filamentous structure is first accomplished by the calpain system or caspases, both cysteine proteases (Goll et al., 2008). Once liberated, they are further degraded by the ubiquintin proteasome pathway using a family of ubiquitin-based ligases, or by the autophagy-lysosymal pathway; the latter involves the creation of autophagosomes and fusion to lysosomes. Post-transcriptional gene regulation involves extensive processes including gene splicing, modification, transportation, translation and eventual degradation. This process has been poorly studied in muscle growth, development and metabolism. Previous studies from the Wisconsin Station show RNA binding proteins are important regulators in each step of posttranscriptional process. Their studies demonstrate that RNA binding protein 20 (RBM20) specifically regulates skeletal muscle gene splicing that is associated with muscle growth, development and metabolism. Most interestingly, they found that another RNA binding protein 39 (RBM39) plays an important role in maternal obesity-induced fetal muscle growth and development. The entirety of the aforementioned is a highly dynamic and an evolving field of inquiry. The potential ramifications of better understanding the control of protein accretion for animal agrircuture are monumental, however.
MUSCLE FIBER TYPE AND PLASTICITY: Muscle is a collection of cells differing in their ability to contract and metabolize energy (Suzuki et al., 1976). The identity of a myofiber is controlled by several factors, including motor neuron innervation, hormonal regulation, progenitor cell origin, and myofiber type-specific gene expression (Schiaffino and Reggiani, 2011). Each mature myofiber is mono-innervated by a single motor neuron, which transmits electrical impulses to a subset of myofibers. Firing of slow motor neurons (motor neurons innervating slow myofibers) generates sustained and low-amplitude elevations in intracellular calcium concentrations, leading to the activation of calcineurin (Dolmetsch et al., 1997). In contrast, fast motor neuron firing leads to brief calcium transients of high amplitude, which are insufficient to activate calcineurin. Activated calcineurin activates Nuclear factor of activated T-cells (NFAT) and myocyte enhancer factor-2 (Potthof et al., 1997). Besides motor neurons, Twist Family BHLH Transcription Factor 2 (Twist2)-dependent progenitor cells are recently shown to maintain IIb myofibers (Liu et al., 2017). In addition, myofiber-type specific genes or microRNAs are shown to contribute to myofiber specifications (Stark et al., 2015). However, gene programs that regulate subtypes of fast myofibers are poorly understood.The relative proportion of each fiber type is associated with meat quality and growth rate. Exploring the impact of muscle fiber type on animal growth and meat quality could provide selection criteria for identifying superior genetics and management schemes for producing high quality meat. A number of stations, Kansas, Florida, Virginia, Utah, Mississipi and Alabama have documented significant differences in meat animal species compared to the aforementioned rodents. First, poultry has nearly all white, fast contracting fibers (Alabama). Pigs on the other hand, are a mix of red and white fibers, though white fibers dominate (Virginia). Fiber type in cattle and horse is much different that rodent in that it has no extremely fast contracting muscle, rather is very oxidative, more like humans (Kansas, Florida, Mississipi, Utah and Virginia). These translational discoveries help us understand better why rodent and human-based data differs so dramatically on occasion.
GENETICS: Genomic information is now routinely used to predict the complex phenotype of an individual with a specific genotype. As many advances in biology are driven by genome sequence information, animal genomics is on the cusp of a new era in predictive biology. An excellent, highly contiguous porcine genome assembly has recently been completed (Warr et al., 2019), and as costs continue to decrease, availability of genotype information is no longer the bottleneck of scientific progress. The next challenge is to read the complexity of these instructions across animals, and to predict the resulting phenotypes, i.e., to predict specific consequences encoded in specific genome sequences. The Michigan and Station is using a multi-omic approach to characterize the underlying biological mechanisms controlling pig skeletal muscle growth, carcass composition and meat quality. Skeletal muscle transcriptional profiles are integrated with genetic marker genotypes to identify genes associated with phenotypic traits. This effort includes determination of allele-specific expression patterns, and transcript splicing variants. Furthermore, the Texas Station has identified genes, gene variants, and genetic regulators such as microRNAs and other transcripts that confer variation in growth, development, and composition of skeletal muscle, as well as factors that influence the deposition of associated intramuscular fat and other meat phenotypes in beef cattle. This work was conducted via genetic, functional genomic, and high throughput sequencing analyses of crossbred beef cattle from a defined, multi-generation genetic mapping herd. identify genes and gene networks responsible for specific adult skeletal muscle characteristics. At least 60 different phenotypes collected from these animals are available in conjunction with genomic data, including meat carcass traits, quantitative and sensory panel analyses of steaks for tenderness, flavor, and juiciness, feed efficiency, and others. Identifying the genes and regulatory elements in skeletal muscle will increase our ability to annotate functional regions related to growth and other important phenotypes.
MITOCHONDRIAL BIOSYNTHESIS, FUNCTION AND AUTOPHAGY: Mitochondria form the basis for nutrient utilization in skeletal muscle cells. Mitochondria are not just oblong, individual organelles located in the subsarcolemmal space but rather these ATP generating powerhouses are intricately connected throughout the innermost workings of the muscle fiber and are now known to be intimately associated with the sarcoplasmic reticulum. As such, these organelles impact calcium homeostasis in skeletal muscle. Moreover, these electrostatic compartments create and dispense of reactive oxygen species, the balance of which can have a profound impact on mitochondria function (Bottje, 2019). When mitochondria are damaged, they are cleared by autophagy, involving LC3-II, p62, and autophagic flux (Saito and Sadoshima, 2015). Developing a better understanding of mitochondrial function/dysfunction is important for improving efficiency of meat production. The Iowa Station has shown during muscle atrohy that decreased autophagy and necessary lysosymal abundance is due to oxidative stress in the tissue. These novel mechanisms, even to the biomedical community, have been created using translational pig models with human health implications. At the same time, however, the opposite mechanism of hypertrophy which is hallmark of animal growth, may be impacted by similar mechanisms.
HEAT STRESS: Global changes in climate have and will continue to impact how meat animals are produced. Animal cooling and feeding low heat increment diets alleviate heat stress (HS), but HS events continue seasonally (Baumgard and Rhoads, 2012). Most animals, including pigs, when on a lower plane of nutrition, initiate metabolic adaptations to repartition nutrients towards muscle growth and away from adipose accretion. As heat-stressed pigs (which voluntarily reduces nutrient intake) have higher fat accretion at the expense of muscle growth, it is apparent that this typical response to hypophagia is opposite during a HS eveny. However, the mechanism that leads to this change has not been established. Developing etiological interventions to counter this loss of efficient meat production is limited by our poor understanding of the cascade of cellular dysfunction caused by chronic hyperthermia. Moreover, the Virigina Station with the Iowa Station have shown that heat stress alters whole body metabolism. These developments, thought quite early in their discovery, these developments may lead to changes in feeding during and immediately after a bout of heat stress.
FETAL PROGRAMMING: Fetal development is important as developing tissues are most sensitive to external stresses at this time (Du et al., 2010). The Wyoming and Washington Stations have documented improper early development due to maternal stresses sets the negative trajectory for long-term growth performance of meat animals. The common origins of muscle cells and adipocytes provide an opportunity to prime myogenic instead of adipogenic differentiation, which is postulated to enhance lean/fat ratio of offspring. Initial formation of muscle fibers starts at the embryonic stage and continues during the fetal stage. Conversely, postnatal muscle growth mainly occurs through hypertrophy of existing muscle fibers, where satellite cells play a critical role. Thus, increasing muscle fiber number and satellite cell density are two critical control points during the early development that could enhance lean growth. The Conneticut Station muscle fiber size in sheep differs with muscle and time of gestational development. Further they show that undernutrition alter the number of secondary fibers developed and it may be to inflammation status. Adipogenic progenitor cells also develop during the embryonic stage, while the differentiation and maturation of adipocytes occurs during fetal development and after birth. In addition, intramuscular fat develops later than other fat depots, which provides an opportunity to promote intramuscular adipogenesis with less impacts on other fat depots. However, the effect maternal stressors on the early development, and lineage commitment of myogenic and adipogenic cells remain poorly understood.
INTRAUTERINE GROWTH RESTRICTION: Deficits in metabolic function and muscle mass result from fetal adaptations to an intrauterine stress, which are often termed IUGR since late fetal growth restriction is the chief diagnosis (Wu et al., 2006). Placental insufficiency and maternal obesity are common causes of IUGR, an aspect of fetal programming. Both are associated with maternofetal inflammation and neither can be directly mitigated. Thus, the only recourse is to manage the resulting fetal adaptations, which requires a better understanding of the underlying molecular mechanisms. In response to nutrient-limiting stressors, the fetus develops two major adaptations in skeletal muscle aimed at sparing nutrients for heart, brain, and endocrine tissues. The Virginia Station has shown IUGR skeletal muscle develops a metabolic shift whereby it reduces glucose oxidation rates in favor of glycolytic lactate production. They have also shown IUGR fetus restricts skeletal muscle growth. Skeletal muscle accounts for only ~40% of total mass but up to 85% of total glucose consumption, and thus limiting muscle mass allows re-appropriation of nutrients for vital organ growth and development. The Nebraska Station, using a sheep IUGR model have shown that inflammatory pathways up-regulate in these compromised fetus, namely TNF alpha, Interleukin and toll-like pathways. These IUGR adaptations disrupt glucose homeostasis and lead to metabolic dysfunction. Better understanding the growth of IUGR animals may allow us to optimize fetal growth and future growability of animals postnatal.
MEAT QUALITY AND ADIPOSITY: Marbling, intramuscular adipose tissue, remains one of the most important factors determining meat quality. Unlike excessive subcutaneous and visceral adipose tissues which have relatively low or detrimental value to the carcass, intramuscular adipose tissue directly influences the palatability meat through providing a lubrication effect, increased juiciness, and a source of lipophilic flavor compounds (Eastwood et al., 2017). Differentiation of adipoblasts is responsible for the formation of intramuscular adipose tissue (Joe et al., 2010). These progenitor cells are composed of individual cells with the ability to generate adipocytes in the skeletal muscle. Adipocyte progenitor cells also have fibrogenic capacity and can differentiate into fibroblasts/myofibroblasts. Fibroblasts/myofibroblasts express a high level of fibrillar collagens, as well as ECM modification enzymes including lysyl oxidase, matrix metalloproteinases (MMPs), and tissue inhibitors of MMPs, which leads to tissue fibrosis. Fibro/adipogenic progenitors (FAPs) differentiate into fibroblasts and adipocytes. The adipogenic and fibrogenic capacities of these cells vary, possibly due to differentially regulated lineage commitment. Understanding the molecular control of these FAPs may lead to our understanding of the differences in highly marbled beef occur. This is a new area for the committee and little has been accomplished to date by members of NC-1184.
2015 Outputs (First Year)
Collaborative Grants
- Collaborative NIFA-AFRI Grant between Ohio State University with the Michigan State Station (Gale Strasburg) Strasburg, G., Velleman, S.G. Reed, K., NIFA AFRI, “Influence of Thermal Challenge on Turkey Muscle Development and Meat Quality,” $975,000, 3/2014-2/2017.
- USDA 2014-67015-21627. Collaborative NIFA-AFRI Grant between the Iowa and Virginia stations. JT Selsby, L Baumgard, S Lonergan, JW Ross, and R Rhoads. The effect of heat stresses on porcine skeletal muscle. $499,881, 1/2014-12/2017.
Grants
- Wise Burroughs Fund. Selsby (PI). Au’14-Sp’15. Mechanisms and persistence of heat-stress-mediated factors that contribute to growth attenuation in swine: Graduate student support. $38,235.
- USDA/AFRI. S. A. Reed Co-PD (PD: S.A. Zinn, Co-PD: K.E. Govoni). 01/01/2014-12/31/2015. Effects of intrauterine growth retardation (IUGR) on fetal development in sheep. $150,000.
- NIH Eunice Kennedy Shriver National Institute of Child Health and Human Development 1R01 HD070487. T. G. Anthony (PI). 07/25/11 – 04/30/16. Molecular Mechanisms of Adverse Metabolic Events by Asparaginase. $250,000 per year direct.
- National Institute of Food and Agriculture, U.S. Department of Agriculture competitive grant: award number 2012-67015-19447. W. R. Dayton. 5/1/1012-4/30/2017. Novel role of the Epidermal Growth Factor Receptor in both Insulin-like Growth Factor-1 and Estradiol-stimulated bovine muscle growth. $500,000.
- Zoetis: W. R. Dayton. 1/1/2015-12/31/2015$14,000.
- Arkansas Biosciences Institute: Baum JI (PI) and Borsheim E. (2015-2018) Nutrition intervention to improve energy metabolism, energy intake, and metabolic response in overweight and obese school-aged children. $150,000.
- Arkansas Biosciences Institute: Proctor A (PI), Anthony NB, Baum JI, Lay JO, Shinn SE. Conjugated Linoleic Acid (CLA) Rich Traditional Egg Breakfast Foods to Combat Obesity Related Diseases in Arkansas. $150,000 (Baum - $50,000)
- University of Arkansas Honors College Research Grant Award. Inman T and Baum JI (PI). (2015-2016) Defining the role of leucine in skeletal muscle energy metabolism for prevention of type 2 diabetes. $3,500.
- University of Arkansas Honors College Research Grant Award. Kubas G and Baum JI (PI). (2015-2016). Nutrient modulation of the mammalian target of rapamycin (mTOR) to improve muscle function during aging. $3,500.
- University of Illinois Research Board. A. Dilger (PI). June 2014-December 2015. Creation and characterization of a novel mutation to increase postnatal expression of IGF2 in mice. $20,000.
- University of Illinois Division of Nutritional Sciences Vision 20/20. M. DeLisio (PI), N. Burd, N. Khan and A. Dilger (co-PIs). October 2014-October 2016. The effects of overweight/obesity and acute dietary protein ingestion on muscle stem cell function. $22,500.
- GBH Labs, LLC. J. M. Gonzalez. 05/01/15-04/30/15. Effect of a plasma product on fetal and neonatal myoblast activity. $46,869.
- Gates Foundation. petitte and Mozdziak. 02/01/2012-03/31/2015. Reducing Disease In Livestock. $100,000.00.
- Agriculture Foundation. Mozdziak. 07/01/2014-06/30/2017. 'Embryonic manipulations aimed at increasing muscle growth and meat yield'. $65,000.00
Publications
- Johnson JS, Abuajamieh M, Sanz Fernandez MV, Seibert JT, Stoakes SK, Keating AF, Ross JW, Selsby JT, Rhoads RP, and Baumgard LH. The impact of in utero heat stress and nutrient restriction on progeny body composition. Journal of Thermal Biology. In Press.
- Quindry JC, Ballmann CG, Epstein EE, and Selsby JT. Plethysmography measurements of respiratory function in conscious unrestrained mice. The Journal of Physiological Sciences. In Press.
- Hollinger K and Selsby JT. PGC-1α gene transfer improves muscle function in dystrophic muscle following prolonged disease progression. Experimental Physiology. 100:1145-1158, 2015. Designated an Editor’s Choice; Art chosen for cover
- Selsby JT, Ross JW, Nonneman D, and Hollinger K. Porcine models of muscular dystrophy. International Laboratory Animals Research Journal. 56:116-126, 2015.
- Reed S.A., E.K. LaVigne, A.K. Jones, D.F. Patterson, A.L. Schauer. The aging horse: effects of inflammation on equine satellite cells. J Anim Sci. 2015. Feb; 93:862-870. doi:10.2527/jas.2014-8448
- LaVigne, E.K., A.K. Jones, A. Sanchez Londoño, A.S. Schauer, D.F. Patterson, J.A. Nadeau, and S.A. Reed. Muscle growth in young horses: effects of age, cytokines, and growth factors. J Anim Sci, in press.
- Nie Y, Gavin TP, Kuang S. Measurement of resting energy metabolism in mice using Oxymax Open Circuit indirect calorimetry. Bio Protoc. 5(18): e1602.
- Li YH, Luo J, Mosley YY, Hedrick VE, Paul LN, Chang J, Zhang G, Wang YK, Banko MR, Brunet A, Kuang S, Wu JL, Chang CJ, Scott MP, Yang JY. 2015. AMP-activated protein kinase directly phosphorylates and destabilizes Hedgehog pathway transcription factor GLI1 in medulloblastoma. Cell Rep. 12(4):599-609.
- Zhang P, Liang X, Shan T, Jiang Q, Deng C, Zheng R, Kuang S. 2015. mTOR is necessary for proper satellite cell activity and skeletal muscle regeneration. Biochem Biophys Res Commun. 463(1-2): 102-8.
- Bi P, Kuang S. 2015. Notch signaling as a novel regulator of energy metabolism. Trends Endocrinol Metab. 26(5):248-55.
- Wang JH, Wang Q, Wang C, Reinholt B, Grant AL, Gerrard DE, Kuang S. 2015. Heterogeneous activation of a slow myosin gene in proliferating myoblasts and differentiated single myofibers. Dev Biol. 402(1):72-80.
- Shan T, Zhang P, Bi P, Kuang S. 2015. Lkb1 deletion promotes ectopic lipid accumulation in muscle progenitor cells and mature muscles. J Cell Physiol. 230(5): 1033-41.
- Yang X, Bi P, Kuang S. 2014. Fighting Obesity: When muscle meets fat. Adipocyte. 3(4): 371-80.
- Zhang P, Shan T, Liang X, Deng C, Kuang S. 2014. Mammalian target of rapamycin is essential for cardiomyocyte survival and heart development in mice. Biochem Biophys Res Commun. 452(1):53-9.
- Anthony, TG, Mirek, ET, Bargoud, AR, Phillipson-Weiner, L, DeOliveira, CM, Wetstein, B, Graf, BL, Kuhn, PE, Raskin I. (2015) Evaluating the Effect of 20-Hydroxyecdysone (20HE) on Mechanistic Target of Rapamycin Complex 1 (mTORC1) Signaling in the Skeletal Muscle and Liver of Rats. Applied Physiology, Nutrition, and Metabolism, DOI: 10.1139/apnm-2015-0301.
- Duan Y, Li F, Li Y, Tang Y, Kong X, Feng Z, Anthony TG, Watford M, Hou Y, Wu G, Yin Y. (2015) The role of leucine and its metabolites in protein and energy metabolism. Amino Acids. 2015 Aug 9. [Epub ahead of print] PMID: 26255285
- Layman DK, Anthony TG, Rasmussen BB, Adams SH, Lynch CJ, Brinkworth GD, Davis TA. (2015) Defining meal requirements for protein to optimize metabolic roles of amino acids. Am J Clin Nutr. 2015 Apr 29. pii: ajcn084053. [Epub ahead of print] PMID: 25926513
- Kamanga-Sollo, E., Thornton, K. J., White, M. E. and Dayton, W. R. 2014. Role of G protein-coupled estrogen receptor-1 matrix metalloproteinases 2 and 9, and heparin binding epidermal growth factor-like growth factor in estradiol-17beta-stimulated bovine satellite cell proliferation. Domest. Anim. Endocrinol. 49:20-26.
- Thornton, K. J., Kamange-Sollo, E, White, M. E., Dayton, W. R. 2015. Role of G protein-coupled receptors (GPCR), matrix metalloproteinases 2 and 9 (MMP2 and MMP9), heparin-binding epidermal growth factor-like growth factor (hbEGF), epidermal growth factor receptor (EGFR), erbB2, and insulin-like growth factor 1 receptor (IGF-1R) in trenbolone acetate-stimulated bovine satellite cell proliferation. J Anim Sci 93:4291-4301.
- Hergenreder, J. E., J. F. Legako, T. T. N. Dinh, K. S. Spivey, J. O. Baggerman, P. R. Broadway, J. L. Beckett, M. E. Branine, and B. J. Johnson. 2015. Zinc methionine supplementation impacts gene and protein expression in calf-fed Holstein steers with minimal impact on feedlot performance. Biol. Trace Elem. Res. doi: 10.1007/s12011-015-0521-2.
- Hosford, A. D., J. E. Hergenreder, J. K. Kim, J. O. Baggerman, F. R. B. Ribeiro, M. J. Anderson, K. S. Spivey, W. Rounds, and B. J. Johnson. 2015. Effects of supplemental lysine and methionine with zilpaterol hydrochloride on feedlot performance, carcass merit, and skeletal muscle fiber characteristics in finishing feedlot cattle. J. Anim. Sci. 93:4532-4544.
- Tokach, R. J., F. R. B. Ribeiro, K. Y. Chung, W. Rounds, and B. J. Johnson. 2015. Chromium propionate enhances adipogenic differentiation of bovine intramuscular adipocytes. Front. Vet. Sci. 2:26. doi: 10.3389/fvets.2015.00026.
- Choi, S.H., S.K. Park, C.W. Choi, X.Z. Li, K.Y. Kim, W.Y. Kim, J. Jung, B.J. Johnson, L. Zan, and S. B. Smith. 2015. The expression of adipogenic genes in adipose tissues of feedlot steers fed supplementary palm oil or soybean oil. Asian-Austr. J. Anim. Sci. doi.:http://dx.doi.org/10.5713/adas.15.0011.
- Choi, S. H., S. K. Park, B. J. Johnson, K. Y. Chung, C. W. Choi, K. H. Kim, W. Y. Kim, and S. B. Smith. 2015. AMPKα, C/EBPβ, CPT1β, GPR43, PPARγ, and SCD gene expression in single- and co-cultured bovine satellite cells and intramuscular preadipocytes treated with palmitic, stearic, oleic, and linoleic acid. Asian Australian J. Anim. Sci. 28:411-419.
- Vipham, J. L., G. H. Loneragan, L. M. Guillen, J. C. Brooks, B. J. Johnson, A. Pond, N. Pond, and M. M. Brashears. 2015. Reduced burden of Salmonella enterica in bovine subiliac lymph nodes associated with administration of a direct-fed microbial. Zoonoses and Public Health. Doi: 10.1111/zph.12187.
- Blackwell, B. R., B. J. Johnson, M. D. Buser, G. P. Cobb, and P. N. Smith. 2015. Degradation kinetics of trenbolone acetate metabolites and estrogens in urine and feces of implanted steers. Chemosphere. 138:901-907.
- Blackwell, B. R., K. J. Wooten, M. D. Buser, B. J. Johnson, G. P. Cobb, and P. N. Smith. 2015. Occurrence and characterization of steroid growth promoters associated with particulate matter originating from beef cattle feedyards. Environmental Science and Technology 49:8796-8803.
- Johnson, B. J., S. B. Smith, and K. Y. Chung. 2014. Historical overview of the effect of β-adrenergic agonists on beef cattle production. Asian Australian J. Anim. Sci. 27:757-766.
- Parr, S. L., T. R. Brown, F. R. B. Ribeiro, K. Y. Chung, J. P. Hutcheson, B. R. Blackwell, P. N. Smith, and B. J. Johnson. 2014. Biological responses of beef steers to steroidal implants and zilpaterol hydrochloride. J. Anim. Sci. 92:3348-3363.
- Baum JI and Wolfe RR (2015) The link between dietary protein intake, skeletal muscle function and health in older adults. Healthcare (Review) 3(3): 529-543. doi:10.3390/healthcare3030529
- Baum JI, Gray M, Binns A (2015) Breakfasts higher in protein intake improve energy expenditure, increase fat oxidation and reduce hunger in overweight 8-12 year old children. The Journal of Nutrition in press. doi: 10.3945/ jn.115.214551
- Brown LA, Lee DE, Patton JF, Perry RA, Brown JL, Baum JI, Smith-Blair N, Greene NP, Washington TA (2015). Diet-induced obesity alters anabolic signaling in mice at the onset of muscle regeneration. Acta Physiologica accepted doi: 10.1111/apha.12537
Peer-reviewed Journals
- Harthan, L.B., McFarland, D.C., and Velleman, S.G. 2014. The effect of nutritional status and myogenic satellite cell age on proliferation, differentiation, and expression of myogenic transcriptional regulatory factors and heparan sulfate proteoglycans syndecan-4 and glypican-1. Poult. Sci. 93:174-186.
- Powell, D.J., McFarland, D.C., Muir, W., Cowieson, A., and Velleman, S.G. 2014. The Effect of Nutritional Status and Muscle Fiber Type on Myogenic Satellite Cell Fate and Apoptosis. Poult. Sci. 93:163-173.
- Maliala, Y., Carr, K.M., Ernst, C.W., Velleman, S.G., Reed, K.M., and Strasburg, G.M. 2014. Deep Transcriptome Sequencing Reveals Differences in Global Gene Expression Between Normal and Pale, Soft, and Exudative (PSE) Turkey Meat. J. An. Sci. 92:1250-1260.
- Velleman, S.G., Coy, C.S., and Emmerson, D.A. 2014. Effect of the Timing of Posthatch Feed Restrictions on Broiler Breast Muscle Development and Muscle Transcriptional Regulatory Factor Gene Expression. Poult. Sci. 93:1484-1494.
- Long, J.A., Purdy, P.H., Zuidberg, K., Hiemstra, S-J., Velleman, S.G., and Woelders, H. 2014. Cryopreservation of turkey semen: Effect of breeding line and freezing method on post-thaw sperm quality, fertilization, and hatching. Cryobiology http//dx.doi.org/10.1016/j.cryobiology.2014.04.003.
- Powell, D.J., McFarland, D.C., Muir, W., Cowieson, A., and Velleman, S.G. 2014. The Effect of Nutritional Status on Myogenic Gene Expression of Satellite Cells Derived from Different Muscle Types. Poult. Sci. 93:2278-2288. (selected by the Editor-in-Chief as a Choice Publication based on content)
- Velleman, S.G., Coy, C.S., and Emmerson, D.A. 2014. Effect of the timing of posthatch feed restrictions on the deposition of fat during broiler breast muscle development. Poult. Sci. 93:2622-2627.
- Velleman, S.G. 2014. Effect of growth selection on adipogenic gene expression during the development of the turkey breast muscle. Intl. J. Poult. Sci. 13:680-684.
- Dodson, M., Allen, R., Du, M., Bergen, W., Velleman, S., Poulos, S., Culver, M., Wheeler, M., Duckett, S., Young, R., Voy, B., Jiang, Z., and Hausman, G. 2015. Evolution of meat animal growth during the past 50 years: Adipose and muscle stem cells. J. Anim. Sci. 93:457-481. (invited review).
- S.B. Lee, S.K. Park, and Y.S. Kim. 2015. Production of bioactive chicken (Gallus gallus) follistatin-type proteins in E. coli. AMB Express 5:58 doi 10.1186/s13568-015-0142-3
- Martins TS, ML Chizzotti, W Silva, LN Renno, LMP Sanglard, NVL Serao, FF Silva, SEF Guimaraes, MM Ladeira, MV Dodson, M Du, and MS Duarte. (2015). Molecular factors underlying the deposition of intramuscular fat and collagen in skeletal muscle of Nellore and Angus cattle. PLOS One, in press.
- Fu, X., M. J. Zhu, M. V. Dodson, and M. Du. (2015). AMP-activated protein kinase stimulates Warburg-like glycolysis and activation of satellite cells during muscle regeneration. Journal of Biological Chemistry. In press.
- Fu, X., M. J. Zhu, S. Zhang, F. Marc, V. Benoit, and M. Du. (2015). Obesity impairs skeletal muscle regeneration via inhibition of AMP-activated protein kinase. Diabetes. In press.
- Zhang, H., M. Du, Q. Yang, and M. J. Zhu. (2015). Butyrate suppresses murine mast cell proliferation and cytokine production through inhibiting histone deacetylase. Journal of Nutritional Biochemistry, In press.
- Miao, Z. G., L. P. Zhang, X. Fu, Q. Y. Yang, M. J. Zhu, M. V. Dodson, and M. Du. (2015). Invited review: Mesenchymal progenitor cells in intramuscular connective tissue development. Animal, In press.
- Wang, S., M. J. Zhu, and M. Du. (2015). Prevention of obesity by dietary resveratrol: how strong is the evidence? Expert Review of Endocrinology and Metabolism, In press.
- Yang, G., Y. Xue, H. Zhang, M. Du, and M. J. Zhu. (2015). Favorable effects of GSE on intestinal epithelial differentiation and barrier function in IL10-deficient mice. British Journal of Nutrition, 2015:1-9.
- Chen, D., W. Li, M. Du, M. Wu, and B. Cao. (2015). Sequencing and characterization of divergent marbling levels in the beef cattle (Longissimus dorsi muscle) transcriptome. Asian Australasian Journal of Animal Science, 28: 158-165.
- Du, M., W. Bo, M.J. Zhu. (2015). Fetal programming in meat production. Meat Science, 109: 40-47.
- Wei, S., X. Fu, X. Liang, M. Zhu, Z. Jiang, S. M. Parish, M. V. Dodson, L. Zan, and M. Du. (2015). Enhanced mitogenesis in stromal vascular cells derived from subcutaneous adipose tissue of Wagyu compared with those of Angus cattle. Journal of Animal Science, 93: 1015-1024.
- Reis MM, Cooke RF, Cappellozza BI, Marques RS, Guarnieri Filho TA, Rodrigues MC, Bradley JS, Mueller CJ, Keisler DH, Johnson SE, Bohnert DW. 2015. Creep-feeding to stimulate metabolic imprinting in nursing beef heifers: impacts on heifer growth, reproductive and physiological variables. Animal 9:1500-1508
- Rodrigues MC, Cooke RF, Marques RS, Cappellozza BI, Arispe SA, Keisler DH, Bohnert DW. 2015. Effects of vaccination against respiratory pathogens on feed intake, metabolic, and inflammatory responses in beef heifers J Anim Sci 93: 4443-52
- Foote AP, Hales KE, Kuehn LA, Keisler DH, King DA, Shackelford SD, Wheeler TL, Freetly HC. 2015. Relationship of leptin concentrations with feed intake, growth, and efficiency in finishing beef steers. J Amin Sci 93:4401-7
- Rodrigues MC, Cooke RF, Marques RS, Arispe S, Keisler DH, Bohnert DW. 2015. Effects of oral meloxicam administration to beef cattle receiving lipopolysaccharide administration or vaccination against respiratory pathogens. J Animal Sci 93:5018-5027
- Treanor JJ, Geremia C, Ballou MA, Keisler DH, White PJ, Cox JJ, Crowley PH. 2015. Maintenance of brucellosis in Yellowstone bison: linking seasonal food resources, host–pathogen interaction, and life-history trade-offs. Ecology & Evolution 5:3783-3799.
- Anderson J, Kalscheur K, Clapper J, Perry G, Keisler D, Garcia A, Schingoethe D. 2015. Feeding fat from distillers dried grains with solubles to dairy heifers: II. Effects on metabolic profile. J. Dairy Sci. 98:5709-5719
- Stockwell-Goering MG, Benavides EA, Keisler DH, Daniel JA. 2015. Impact of visual, olfactory, and auditory cues on circulating concentrations of ghrelin in wethers. J Animal Sci 93:3886-3890
- Pulley SL, Keisler DH, Stevenson JS. 2015. Concentrations of luteinizing hormone and ovulatory responses in dairy cows before timed artificial insemination. J Dairy Sci. 98:6188–6201
- Silva PRB, Machado KS, Da Silva DNL, Moraes JGN, Keisler DH, Chebel . 2015. Effects of recombinant bovine somatotropin during the periparturient period on innate and adaptive immune responses, systemic inflammation, and metabolism of dairy cows. J. Dairy Sci. 98:4449–4464
- Alves BR, Cardoso RC, Prezotto LD, Thorson JF, Bedenbaugh M, Sharpton SM, Caraty A, Keisler DH, Tedeschi LO, Williams GL, Amstalden M. 2015. Elevated Body Weight Gain During the Juvenile Period Alters Neuropeptide Y-Gonadotropin-Releasing Hormone Circuitry in Prepubertal Heifers. Biol Reprod. 92:1-10
- Dellschaft NS, Alexandre-Gouabau MC, Gardner D, Antignac JP, Keisler DH, Budge H, Symonds ME, Sebert SP. 2015. Effect of pre and postnatal growth and post-weaning activity on glucose metabolism in the offspring. J Endocrinol. 224:171-182
- Kaminski SL, Redmer DA, Bass CS, Keisler DH, Carlson LS, Vonnahme KA, Dorsam ST, Grazul-Bilska AT. 2015. The effects of diet and arginine treatment on serum metabolites and selected hormones during the estrous cycle in sheep. Therio 83:808-16.
- 66. Field ME, Anthony RV, Engle TE, Archibeque SL, Keisler DH, Han H. 2015. Duration of maternal undernutrition differentially alters fetal growth and hormone concentrations. Dom Anim Endo. 51:1-7.
- Johnson JS, Bryant JK, Scharf B, Kishore DK, Coate EA, Eichen PA, Keisler DH, Spiers DE. 2015. Regional differences in the fescue toxicosis response of Bos taurus cattle. Int J Biometeorol. 59:385-396
- Clark, D.L., D.I. Clark, J.E. Beever, and A.C. Dilger. (2015) Increased prenatal IGF2 expression due to the porcine IGF2 intron3-G3072A mutation may be responsible for increased muscle mass. Journal of Animal Science 93:2546-2558.
- Clark, D.L., D.I. Clark, E.K. Hogan, K.A. Kroscher and A.C. Dilger (2015) Elevated insulin-like Growth Factor 2 Expression may contribute to the hypermuscular phenotype of myostatin null mice. Growth Hormone and IGF Research 25:207-218.
- Burnett, D. D., C. B. Paulk, M. D. Tokach, J. L. Nelssen, M. A. Vaughn, K. J. Phelps, S. S. Dritz, J. M. DeRouchey, R.D. Goodband, K. D. Haydon and J. M. Gonzalez. Effects of Added Zinc on Skeletal Muscle Morphometrics and Gene Expression of Finishing Pigs Fed Ractopamine-HCl. Animal Biotechnology. Accepted June 16, 2015.
- Stern, R. Srinivasan Dasarathy, Chris Ashwell, Paul Mozdziak. Effect of hyperammonemia on myostatin and myogenic regulatory factor gene expression in broiler embryos. Animal: An International Journal of Animal Biotechnology. Accepted.
Book Chapters
- Anderson, P. T., B. J. Johnson, and M. Dikeman. 2014. Growth of meat animals: Metabolic modifiers. In: Carrick Devine and Michael Dikeman, editors-in-chief. Encyclopedia of Meat Sciences 2e, Vol. 2, Oxford: Elsevier, 2014. pp. 62-69.
- Inskeep EK, Purdy PH, Keisler DH. 2015. Reproduction. In: The American Sheep Industry Association - Sheep Industry Development Handbook
- Mozdziak. 2014. Meat Species-Poultry In: Carrick Devine and Michael Dikeman, editors-in-chief. Encyclopedia of Meat Sciences 2e, Vol. 2, Oxford: Elsevier.
Abstracts
- Beyers RJ, Ballmann C, Selsby JT, Salibi N, Quindry JC, and Denney TS. Whole-heart T2-mapping at 7T quantifies dystrophic myocardial pathology in mdx/utrn+/- mice. International Society for Magnetic Resonance in Medicine, Toronto, Ontario, Canada, May 30-31, 2015.
- Abuajamieh M, Laughlin EJ, Lei SM, Stoakes SK, Mayorga EJ, Seibert JT, Nolan EA, Sanz Fernandez MV, Ross JW, Selsby JT, Rhoads RP, and Baumgard LB. The effects of recovery time from heat stress on circulating bioenergetics variables and biomarkers of leaky gut. FASEB, Boston, MA March 215.
- Selsby JT, Ballmann CG, and Quindry JQ. Long-term dietary quercetin enrichment improves muscle function in dystrophic skeletal muscle. FASEB, Boston, MA March, 2015.
- Selsby JT and Sterle JA. Student perception of achievement influences student evaluation of teaching. FASEB, Boston, MA, March 2015.
- Ballmann CB, Beyer R, Denney T, Selsby JT and Quindry JC. Effect of chronic dietary quercetin enrichment on cardiac function in dystrophic mice. FASEB, Boston, MA, March 2015.
- Ballmann CB, Beyer R, Denney T, Selsby JT and Quindry JC. Effect of long term quercetin supplementation on dystrophic cardiac pathology in mdx/utrn+/-mice. FASEB, Boston, MA, March 2015.
- Zhao L, McMillan RP, Xie G, Zhang Z, Baumgard L, Selsby J, Ross J, Gabler N, Hulver M, Rhoads RP. Effect of heat stress on porcine skeletal muscle metabolism. FASEB, Boston, MA, March 2015.
- Peters B, Ballmann C, Selsby JT, and Quindry J. Quercetin feeding and spontaneous activity in the aged mdx mouse. South East American College of Sports Medicine, Jacksonville, FL, February 14th, 2015.
- LaVigne, E.K., A. Sanchez Londoño, S. A. Reed. Interleukin-6, tumor necrosis factor-α, insulin-like growth factor-1 and fibroblast growth factor-2 alter proliferation and differentiation of equine satellite cells. J Anim Sci. 2015. 93(E-Suppl 2):336
- McFadden, K. K., M. L. Hoffman, K. N. Peck, S. A. Reed, S. A. Zinn, and K. E. Govoni. Effects of poor maternal nutrition during gestation on protein expression in the liver of lambs. J Anim Sci. 2015. (E-Suppl 2):336-337
- Jones, A. K., R. E. Gately, K. K. McFadden, S. A. Zinn, K. E. Govoni, and S. A. Reed. Identification of early pregnancy and fetal landmarks via transabdominal ultrasound in sheep. J Anim Sci. 2015. (E-Suppl 2):292
- Raja, J. S., S. M. Pillai, A. K. Jones, M. L. Hoffman, K. K. McFadden, K. E. Govoni, S. A. Zinn and S. A. Reed. Poor maternal nutrition decreases longissimus dorsi cross sectional area of fetal offspring at d 45 of gestation. J Anim Sci. 2015. 93(E-Suppl 2):694
- Hoffman, M., K.Peck, J. Wegrzyn, S. Reed, S. Zinn, and K. Govoni. The effects of maternal under- and over-feeding on muscle development of lambs as determined by RNA-seq analysis. J Anim Sci. 2015. 93(E-Suppl 2):694
- Pillai, S. M., J. S. Raja, M. L. Hoffman, A. K. Jones, K. K. McFadden, S. A. Reed, S. A. Zinn and K. E. Govoni. Effects of under- and over-feeding during gestation on organ development of offspring at days 45 and 90 of gestation. J Anim Sci. 2015. 93(E-Suppl 2):293
- Thornton, K. J., E. Kamanga-Sollo, M. E. White, W. R. Dayton. 2015. Role of epidermal growth factor receptor and erbB2 in trenbolone acetate mediated increases in bovine satellite cell proliferation and protein synthesis and decreases in protein degradation. J. Anim. Sci. 93,Suppl. s3:696.
- Lu, Y., J.S. Bradley, S.R. McCoski, J.M. Gonzalez, A.J. Geiger, R.M. Akers, A.D. Ealy and S.E. Johnson. 2015. Caloric restriction reduces protein accretion in skeletal muscle by attenuating IGF-I signaling in young calves. ADSA/ASAS Joint Annual Meeting, July 12-16, Orlando, FL.
- Lu, Y., J.S. Bradley, S.R. McCoski, A.J. Geiger, R.M. Akers, A.D. Ealy and S.E. Johnson. 2015. Muscle fiber hypertrophy is associated with increased expression of key transcriptional and epigenome regulatory genes. ADSA/ASAS Joint Annual Meeting, July 12-16, Orlando, FL.
- Bradley, J.S., M.E. MacGhee, S.R. McCoski, A.M. Reeg, A.D. Ealy and S.E. Johnson. 2015. Plane of nutrition affects muscle fiber hypertrophy and satellite cell activity in neonatal bull calves. ADSA/ASAS Joint Annual Meeting, July 12-16, Orlando, FL.
- Baggerman, J.O., J.E. Hergenreder, and B.J. Johnson. Activity of skeletal muscle satellite cells and shift of skeletal muscle fiber types over time in finishing cattle. 2015, Reciprocal Meats Conference. 2nd place in M. S. Poster Competition.
- Baum JI, Shouse SA, Lassiter K, Bottje WG, Dridi S. (2015) Leucine and omega-3 fatty acids regulate cell bioenergetics via mTOR. FASEB Journal 29:742.16. Poster Presentation
- Baum JI, Smith-Blair N, French WW, Washington TA, Greene NP. (2015) Aerobic exercise (EX) training does not improve body composition or glycemic control in type 2 diabetic rats with pulmonary hypertension (PH). FASEB Journal 29:1044.6. Poster Presentation
- Sungkwon Park, Yongdae Jeong, Sangbeum Lee and Yongsoo Kim. 2015. Porcine myostatin propeptide promotes the proliferation of satellite cells isolated from porcine skeletal muscle. International Conference of the Korean Society for Molecular and Cellular Biology. Sep 21-23. Seoul, Korea.
- Yong Soo Kim, Sang Beum Lee, Sung Kwon Park, and Hyung-Joo Jin. 2015. Production of bioactive myostatin propeptide of various animal species in E. coli. 7th Asia-Pacific Biotech Congress. J. Biotechnol. Biomater Vol 5, Issue 2:29.
- Awat N Yousif, Yong S. Kim, Douglas L Vincent, and Jinzeng Yang. 2015. Transgenic expression of myostatin propeptide prevents muscle loss and fat accumulation in old ages. The Endocrine Society’s 97 Annual Meeting & Expo. March 5-8, San Diego.
- Peres RFG, Pohler KG, Graff HB, Rodrigues ADP, Smith MF, Keisler DH, Vasconcelos JLM. 2015. IGF-1 concentrations during early pregnancy in suckled Nellore beef cows. J. Anim. Sci 93:710.
- Manthey AK, Anderson JL, Perry GA, Keisler DH. 2015. Metabolic profile and onset of puberty in dairy heifers fed reduced-fat distillers grains in replacement of forage. J Anim Sci 93:735.
- Stockwell-Goering MG, Benavides E, Keisler DH, Daniel J. 2015. Impact of visual, auditory, and olfactory cues on circulating concentrations of ghrelin in wethers. Abstract #119 Southern Section ASAS Abstract Book p 48-49.
- Noel, J. A., T. J. Barstow, R. M. Broxterman, G. D. McCoy, K. J. Phelps and J. M. Gonzalez. 2015. Effect of ractopamine-HCl on muscle fiber types and finishing barrow exhaustion. 68th Recip. Meat Conf. Proc., Lincoln, NE.
Theses:
- “Muscle hypertrophy induced by myostatin inhibition is suppressed by rapamycin administration” Donghyuck Choi, Master degree, July 2015 – Yong Kim (advisor)
- Elizabeth Benavides, PhD Dissertation 2015. Development of a novel immunoassay for simultaneous quantification of endocrine parameters. – Duane Keisler (advisor)
- Rachel Gerrard Felton, M.S. Thesis, 2015 “Identification of California Condor (Gymnogyps Californianus) Estrogen Receptors 1 and 2 and their Activation by Suspected Endocrine Disrupting Contaminants ” - Duane Keisler (advisor)
Other publications and presentations:
- Selsby JT. Quercetin as a novel therapeutic approach for Duchenne muscular dystrophy. Duchenne Alliance International Meeting, March 6th, 2015.
- Selsby JT, Ballmann CG, and Quindry JQ. Long-term dietary quercetin enrichment improves muscle function in dystrophic skeletal muscle. FASEB, Boston, MA March, 2015.
- Ballmann CB, Beyer R, Denney T, Selsby JT and Quindry JC. Effect of long term quercetin supplementation on dystrophic cardiac pathology in mdx/utrn+/-mice. FASEB, Boston, MA, March 2015.
- Selsby JT. Heat stress mediated changes in skeletal muscle. Heat Stress Symposium, ISU, Ames, IA 4/17/15.
- Baum JI and Rodibaugh R (2014) Breakfast and Its Effect on Weight. FSFCS87. University of Arkansas Division of Agriculture Research & Extension. http://www.uaex.edu/publications/PDF/FSFCS87.pdf
- Baum JI and Rodibaugh R (2014) The Power of Protein at Breakfast for School-Aged Children. FSFCS86. University of Arkansas Division of Agriculture Research & Extension. http://www.uaex.edu/publications/PDF/FSFCS86.pdf
- Baum JI and Rodibaugh R (2014) The Power of Protein. FSFCS85. University of Arkansas Division of Agriculture Research & Extension. http://www.uaex.edu/publications/PDF/FSFCS85.pdf
- Baum JI Nutrition Unscrambled Blog, December 2014 - Physiological Impacts of Protein and Effects on Weight Management (Dallas Johnson, Undergraduate Student) http://www.nutritionunscrambled.org/2014/12/physiological-impacts-of-protein-and-effects-on-weight-management/
- Baum JI Nutrition Unscrambled Blog, December 2014 – The Link Between Nutrition, Exercise and Muscle (Brianna Neumann, MS Student) http://www.nutritionunscrambled.org/2014/12/the-link-between-nutrition-exercise-and-muscle/
- Baum JI YouTube Interview (2015b) Protein Breakfast Increases Fat Metabolism https://www.youtube.com/watch?v=omPnw-thd3U
- J. M. Gonzalez. Effect of Zilpaterol-HCl and Postmortem Aging on Muscle Structure and Cooked Meat Tenderness. 2015. The 101st Annual Kansas State University Cattlemen’s Day, Manhattan, KS.
- Mozdziak. Insights into Muscle Development Using Genetically Modified Chickens
- Charles University, Laboratory of Molecular Embryology. Prague North Carolina Czechoslovakia Start Date: 12/03/2014 End Date: 12/03/2014
2019 Outputs (Last Year)
Collaborative Grants:
- Strasburg, G. (PD), Velleman, S.G., and Reed K.N. AFRI. “Influence of Thermal Challenge on Turkey Muscle Development and Meat Quality.” $975,000. 3/2014 – 2/2019.
- Selsby, J. (PD), Baumgard, L. and Rhodes, R. (Co-PD). AFRI. “Therapeutic approaches to heat stress: targeting mitochondria.” $497,000. 01/2018 – 12/2021
Collaborative Publications:
- Apaoblaza A, Gerrard SD, Matarneh SK, Wicks JC, Kirkpatrick L, England EM, Scheffler TL, Duckett SK, Shi H, Silva SL, Grant AL, D.E. Gerrard. Muscle from grass- and grain-fed cattle differs energetically. Meat Sci. 2019 Nov 6;161:107996. doi: 10.1016/j.meatsci.2019.107996.
- Smith, Z.K., B.P. Holland, A.B. Word, G.I. Crawford, W.N. Nichols, B.L. Nuttleman, M.N. Streeter, J.P. Hutcheson, and B.J. Johnson. 2019. Effects of a single intial and delayed release implant on arrival compared with a non-coated initial implant and a non-coated terminal implant in heifers fed across various days on feed. Translational Animal Science doi: 10.1093/tas/txz127
- Smith, Z.K., J.K. Kim, and B.J. Johnson. 2019. Biological responses to coated and non-coated steroidal implants containing trenbolone acetate and estradiol benzoate in finishing steers. J Anim Sci Accepted on Aug. 26, 2019: JAS-2019-3369.R2
- Yang, Z., M.S. Hasan, J.K. Htoo, D.D. Burnett, J.M. Feugang, M.A. Crenshaw, and S.F. Liao. 2019. Effects of dietary supplementation of L-methionine vs. DL-methionine on performance, plasma concentrations of free amino acids and other metabolites, and myogenesis gene expression in young growing pigs. Transl. Anim. Sci. 3:113-123. https://doi.org/10.1093/tas/txy109.
- Barnes, N.E., K.M. Mendoza, G.M. Strasburg, S.G. Velleman and K.M. Reed. 2019. Thermal challenge alters the transcriptional profile of the breast muscle in turkey poults. Poult. Sci. 98:74-91.
- Ahmadpoura, A., C.C. Reichhardta, R.G. Christensena, N.E. Inecka, G.K. Murdoch, and K.J. Thornton Understanding the relationship between trenbolone acetate and polyamines relative to proliferation of bovine satellite cells. Submitted to Domestic Animal Endocrinology for review
- Chen Z., R. Maimaiti, C. Zhu, H. Cai, A. Stern, P. Mozdziak, Y. Ge, S.P. Ford, P.W. Nathanielsz, and W. Guo. 2018. Z-band and M-band titin splicing and regulation by RNA binding motif 20 in striated muscles. J Cell Biochem. 119:9986-9996. https://doi.org/10.1002/jcb.27328
- Zhao, L., Y. Huang, and M. Du. (2019). Farm Animals for Studying Muscle Development and Metabolism: dual purposes for animal production and human health. Animal Frontiers, 9:3.
- Zhang, S., Y. Zhang, X. Zhou, X. Fu, J. Michal, G. Ji, M. Du, and Z. Jiang. (2018). Alternative polyadenylation drives genome-to-phenome information detours in the AMPKα1 and AMPKα2 knockout mice. Sci Rep, 8: 6462.
- Wang, B., C. L. Harris, W. Nie, X. Fu, J. M. Deavila, M. J. Zhu, M. Maquivar, S. M. Parish, J. R. Busboom, M. L. Nelson, and M. Du. (2018). Neonatal vitamin A injection promotes cattle muscle development and increase oxidative muscle fibers. J Animal Sci Biotech, 9: 82.
- Li, X., X. Fu, G. Yang, and M. Du. (2019). Review: Enhancing intramuscular fat development via targeting fibro-adipogenic progenitor cells in meat animals. Animal, In press.
Abstracts, Posters, and Professional Presentations:
- Baumgard, LH, Rhoads RP, Ross JW, Keating AF, Gabler NK, and Selsby JT. The intestinal, metabolic, inflammatory and production responses to heat stress. Annual Meeting of the European Federation of Animal Science (EAAP), Ghent, Belgium August, 2019.
- Selsby JT, Ganesan S, Rhoads RP, and Baumgard LH. The heat is on: heat stress induces radical change in skeletal muscle. American Society of Animal Scientists, Austin, TX, July, 2019.
Theses/Dissertations:
- Lisa Armbruster: (MS) Myogeneic and Anabolic Gene Expression in Red and White Muscle of Sablefish during Grow Out. University of Idaho
- Stephen Tamm: (MS) Impact of adipocytes on the microniche, proliferation and fusion of cultured myoblasts. University of Idaho
- Antonetta Colacchio (MS) Association of carcass maturity grade and genes associated with growth in young heifers. University of Idaho
- Avani Gouru (MS) Myogeneic transcription factors and ornithine decarboxylase mRNA are influenced by polyamine supplementation in vitro. University of Idaho
- Racheal Lemire (MS) Mississippi State University
- Randi Owen (MS) Use of EconomasE to improve mitochondrial biogenesis and capacity in young performance horses. Texas A&M
- Hannah Valigura (MS) Influence of supplementation of Saccharomyces cerevisiae fermentation product on inflammation in young horses. Texas A&M
- Zhongyue Yang (MS) Effects of dietary supplementation of L-methionine vs DL-methionine on performance, plasma concentrations of free amino acids and metabolites, and myogenesis gene expression in young growing pigs. Mississippi State University
- Amanda Liefeld (MS) Effects of restricted maternal nutrition and re-alimentation on fetal muscle development during mid and late gestation in sheep. University of Connecticut
- Nicholas Margolies (MS) Role of ATF4 in Dietary Sulfur Amino Acid Restriction. Rutgers
- Robert Posont (MS) Understanding neonatal pathophysiology and intervention strategies after sustained maternal inflammation during late gestation. University of Nebraska
- Matthew Simmons (MS) A quantitative genetic analysis of the ancestry of Neil Trask line bred Hereford cattle. Texas A&M
- Oscar Tejeda (MS) Impact of in ovo thermal manipulation on broiler chicken growth performance, carcass yields, and satellite cell mitotic activity and fiber morphometrics. Auburn University
- Graham Williford (Master’s of Agriculture) Texas A&M
- Hannah Spaulding (PhD) Stimulation of PGC-1α to attenuate Duchenne muscular dystrophy disease pathology and activate autophagy. Iowa State University
- Amanda Brandt (PhD) Regulation of satellite cells by extrinsic factors during recovery from exercise in horses. Virginia Tech
- Caitlin Cadaret (PhD) Effects of fetal inflammatory adaptations to maternal stress on subsequent muscle growth and metabolic function. University of Nebraska
- Mingming Sun (PhD) Molecular mechanism of Rbm20 in pre-mRNA splicing and its new role in glucose metabolism in skeletal muscle. University of Wyoming
- Chaoqun Zhu (PhD) Molecular mechanisms of Rbm20 in titin splicing and heart failure. University of Wyoming
- Dylan Klein (PhD) Fit as a Horse: from Skeletal Muscle Metabolism to Whole Body Physiology. Rutgers
- Rachel Allyssa Stern (PhD) Myogenic Response to Ammonia Differs Between Avian and Mammalian Species. North Carolina State University
- Christine Latham (PhD) Delineating factors that impact lifetime musculoskeletal health in the horse. Texas A&M
Objectives
-
Characterize the molecular mechanisms controlling skeletal muscle tissue growth, development and composition.
-
Characterize the signal transduction pathways that regulate skeletal muscle metabolism.
-
Characterize mechanisms of protein synthesis and degradation in skeletal muscle.
Methods
Objective 1. Characterize the molecular mechanisms controlling skeletal muscle tissue growth, development and composition.
The PI3K-Akt-mTOR pathway regulates cell proliferation and growth in multiple cell types including skeletal muscles. Extrinsic growth factors, such as insulin like growth factor 1 activate this pathway. The Indiana Station will focus on the negative regulator of this growth pathway, phosphatase and tensin homolog (PTEN). Inhibition of PTEN leads to amplification of the Akt-mTOR signaling and muscle hypertrophy, at the expense of reduced number of satellite cells.
Hot and cold thermal challenge has serious physiological consequences for muscle growth and development, including reduced growth, altered lipid deposition, and myodegeneration, all of which have serious impacts on meat quality. Poults have poorly developed thermoregulatory systems, and as temperature extremes grow in frequency and intensity, breast muscle problems will intensify. As progenitors of new muscle growth in the hatchling, muscle satellite cells are highly responsive to thermal stress and to manipulation through management. The Ohio and Michigan Stations will be address how thermal stress affects the function of satellite cells and how these changes affect the growth and development of the turkey breast muscle. Research at the Ohio and Michigan Stations will first define the effects of thermal challenge on commercial turkey satellite cell function by analyzing changes in satellite cell development, transcriptome, non-coding RNAs including the novel class of circular RNAs, and the metabolome. Secondly, research will be extended to the live bird and they will analyze the effects of post-hatch thermal challenge on muscle structure, transcriptome, and metabolome in turkey poults.
Dietary vitamin A is converted into bioactive retinoic acids, which bind to retinoic acid receptors and retinoid X receptors to exert biological effects. In addition, liganded retinoid X receptors also partners with a range of other nuclear receptors to form heterodimers to regulate cell differentiation. The Washington Station plans to study the roles of retinoic acid in mediating myogenesis and muscle development during the early embryonic development. They are planning to use cultured mesenchymal progenitor cells, myogenic cells, and transgenic mice to explore causal relationship between retinoic acid signaling on myogenic commitment and differentiation. Identified mechanisms will be further tested in beef cattle. Furthermore, the Washington Station will also investigate the interaction between retinoic acid receptors with other nuclear receptors in regulating somite patterning, dermomyotome formation and initial myogenic commitment. Accumulating evidence points to the importance of early development in determining long-term performance of animal production.
Fibro-adipogenic progenitor cells (FAP) are a pool of cells that may become either adipose tissue or connective tissue. When this pool of cells is directed toward adipose tissue intramuscular connective tissues will be reduced, thereby both the marbling and tenderness of meat should be increased. The Georgia Station possesses a herd of cattle that display elevated marbling either early or at a typical time period of their life. Studies will be conducted with these cattle to understand the divergent myogenic and adipogenic events occurring with these two populations of cattle. Progenitor cell populations and their activity will be examined in addition to the resulting meat quality of these cattle in collaboration with investigators at the Mississippi Station. Moreover, the Louisana Station will study the gene expression profiles of individual bovine FAPs at different growth and development stages using single-cell RNA-sequencing. They will also explore the efficiency of FAP makers identified in in cell sorting. Sorted FAP subpopulations will be tested for adipogenic and fibrogenic efficiencies in vitro. In addition, the function of the identified marker genes will be tested in vitro by overexpression and knockdown experiments.
The Nebraska Station will identify and target the adaptive mechanisms underlying poor skeletal muscle growth capacity and metabolic function in IUGR/low birthweight fetuses and offspring. By performing studies in a couple sheep IUGR model, they will examine potential molecular targets for pharmaceutical and nutritional interventions to improve muscle-centric insulin responsiveness, myoblast function, and glucose metabolism in late gestation and throughout early growth. They will also use bioelectrical impedance and ultrasound techniques to track muscle growth in vivo. Another focal point of the planned research will be to investigate the adaptive changes in inflammatory signaling pathways and their role in dysregulation of myoblast proliferation and differentiation as well as muscle insulin sensitivity and oxidation. In addition, they will understand whether chronic systemic inflammation of the IUGR fetus is of fetal origin in response to placental insufficiency or is a product of the placenta proper. Their ex vivo cell culture work in myoblasts isolated from these IUGR fetal and neonatal sheep will demonstrate the intrinsic characteristics of the cells, which will allow better detection and evaluation of mechanistic targets for improved growth, which they will then evaluate in animals. The Nebraska Station also plans to investigate how the intrauterine environment of beef cows with high androstenedione (A4) leads to fetal muscle programming that can increase postnatal growth in calves. They will use ex vivo cell culture techniques to evaluate the intrinsic functional characteristics of myoblasts isolated from high A4 cows and calves. They will then compare their findings back to muscle growth metrics measured in animals at harvest. Their expectation is that the conditions responsible for high A4 also impair myoblast function in the cow, but exposure of the calf’s myoblasts to these inhibitory conditions in utero makes them less responsive to the inhibitory tone, allowing greater muscle growth from birth to weaning.
The Mississippi Station will also investigate the circadian regulation of bovine fetal skeletal muscle growth and metabolism. Supplementing gestating heifers and sows with melatonin increases the postnatal growth performance of their offspring though yet unknown mechanisms. Melatonin is a master regulator of circadian rhythmicity so using bovine and porcine models, The group will conduct serial collection of developing fetuses based on circadian clocks to determine the molecular and metabolic regulatory framework around muscle growth and development. Exploration of the protein regulatory networks and collagen content in these models will be in collaboration with scientists at the Georgia Station. Additional collaborations are planned with the Louisiana Station to explore intramuscular lipid deposition and metabolism in these models.
Both over- and restricted-feeding of gestating ewes affect systemic and local inflammation, oxidative status, and metabolism in fetal offspring and these changes likely contribute to the persistent negative effects of poor maternal nutrition in the offspring. The Connecticut Station hypothesizes that poor maternal diet during gestation alters postnatal offspring inflammatory status, which consequently alters skeletal muscle growth, development and composition. To test this hypothesis, they will collect muscle samples from 6-mo-old offspring of ewes fed control, restricted, or over-fed diets. Samples will be analyzed for measures of inflammation and oxidative stress. Further, samples will be tested for meat quality characteristics including shear force, drip loss, collagen content, proximate analysis, etc. to determine if maternal diet alters the quality of the resulting meat. This will be accomplished in conjunction with the Georgia Station.
As stated, prenatal development of muscle fibers is a major determinant of animal growth potential, and is influenced by the maternal environment during gestation. In particular, maternal infection during gestation can negatively affect muscle fiber formation. At the Illinois Station, the influence of maternal infection in pigs on muscle epigenetic programming and lifelong muscle growth potential will be characterized. Nutritional interventions to mitigate these detrimental effects will be explored as well.
The Idaho Station will evaluate the influence of nutrients on temporal and stage dependent myogenic transcription factor expression using molecular approaches. This will include both in vitro and possible ex vivo and in vivo analyses as to changes in determination, proliferation, differentiation, fusion and in contrast apoptosis and/or regression. The influence of bioactive compounds derived from nutrients, supplements or experimental treatments will be characterized in the context of muscle cell and tissue growth.
The South Dakota Station will investigate the utility of trenbolone acetate and estradiol on animal growth performance, carcass traits, and sera metabolites as indicators of tissue anabolism in feedlot finishing steers compared to non-implanted steers. Furthermore, and in collaboration with the Texas Station (Tech) group will study how varying types of steroidal implants (coated vs. non-coated) and finishing diets affect animal growth performance and carcass traits. Their working hypothesis is that altered dietary substrate and implant payout might influence cattle marbling scores.
A common theme in biology is the re-utilization of genes during development and again once muscle tissue maintenance ensues. A number of genes that control muscle development and homeostasis can easily be manipulated using genetics and/or biochemical approaches. The Kansas Station will identify and characterize new genes, including the upstream signaling and downstream effectors that govern muscle formation and growth.
The Mississippi Station will be to characterize the molecular mechanisms, by which dietary nutrients, especially amino acids (such as lysine, methionine, and tryptophan) affect: (1) growth and development of skeletal muscle tissue of pigs, (2) the chemical composition of the skeletal muscle tissue, including the contents of protein and intramuscular fat, and their association with pork quality, and (3) muscle protein turnover and animal growth performance (such as the average daily lean gain). Contemporary swine breeds (the crossbreds of Yorkshire, Landrace, Duroc, and/or Hampshire) from the local commercial farms will be used as research models. The metabolic and cell signaling pathways, as well as gene networks that regulate muscle protein synthesis and degradation in vivo will be explored (obj. 3). Various research techniques commonly used in animal nutrition and molecular biology studies, such as Microarray, RNA-seq, Real-time RT-PCR, proteomics, Western-blotting, etc. will be utilized as needed.
The Florida Station intends to exploit the disparity in heat susceptibility between Brahman and Angus cattle to understand the role of the mitochondria in modulating this difference. A combination of biopsies and ex vivo respiration studies will be used to evaluate nuances controlling energy metabolism in these cattle.
SMAD3 signals underlie maintenance of quiescence in satellite cells while SMAD2-directed signals are responsible for repression of myoblast fusion. Working with the Georgia Station, the Virginia Station will use cattle and mice studies to uncover the divergent roles of SMAD signals in satellite cell activation, replication, fusion and self-renewal.
The Texas Station will identify genes, gene variants, and genetic regulators such as microRNAs and other transcripts that confer variation in growth, development, and composition of skeletal muscle, as well as factors that influence the deposition of associated intramuscular fat and other meat phenotypes in beef cattle. This work will be conducted via genetic, functional genomic, and high throughput sequencing analyses of crossbred beef cattle from a defined, multi-generation genetic mapping herd. This approach will identify genes and gene networks responsible for specific adult skeletal muscle characteristics. At least 60 different phenotypes collected from these animals are available in conjunction with genomic data, including meat carcass traits, quantitative and sensory panel analyses of steaks for tenderness, flavor, and juiciness, feed efficiency, and others. Investigators from the Texas and Oklahoma Stations will collaborate on bioinformatic approaches to better understanding RNA-based regulation of skeletal muscle growth and composition attributes in beef and pork. Along those same lines of inquire, the Michigan Station is using a multi-omic approach to characterize the underlying biological mechanisms controlling pig skeletal muscle growth, carcass composition and meat quality. Skeletal muscle transcriptional profiles are integrated with genetic marker genotypes to identify genes associated with phenotypic traits. This effort includes determination of allele-specific expression patterns, and transcript splicing variants. Samples from fetal pig skeletal muscle are being evaluated to determine developmentally regulated DNA methylation patterns in order to elucidate gene expression regulatory mechanisms, and to identify allele-specific methylation. In addition, transcript abundance and DNA methylation patterns are being assessed to identify stress response mechanisms and the impact of mixing unfamiliar pigs into new social cohorts.
Finally, ammonia is a normal waste product in normal vertebrate metabolism. Hyperammonia is a common clinical consequence of cirrhosis, and hyperammonia has been directly linked to the severe un-recoverable sarcopenia that occurs in the severely affected patients. Interestingly the North Carolina Station has demonstrated that the levels of ammonia in cirrhotic patients are metabolised in an avian system, and similar levels cause a down regulation of myostatin, a major gene product controlling muscle development. The Hawaii and the North Carolina Stations propose to partner on to elucidate myostatin signaling pathways in relation to ammonia in the avian system.
Objective 2. Characterize the signal transduction pathways that regulate skeletal muscle metabolism.
The Indiana Station has identified several key regulators of myogenesis. These include transcriptional factors (such as Ascl2 and Bhlhe40), cell cycle regulators (such as Plk1) and signaling molecules (such as Dlk1, Lkb1, mTOR, Notch, PTEN and Mettl21) and non-coding RNA (miR-133). The station will further define the genetic network and novel signaling mechanisms that regulate embryonic and postnatal myogenesis. Using mouse models, the group will identify unique molecules that are key in controlling myogenesis. The role of these new regulators of myogenesis in large animal species will also be explored.
Post-transcriptional regulation involves extensive processes including gene splicing, modification, transportation, translation and eventual degradation. This process has been poorly studied in muscle growth, development and metabolism. RNA binding proteins are important regulators in each step of posttranscriptional process. The Wisconsin Station demonstrated that RNA binding protein 20 (RBM20) specifically regulates skeletal muscle gene splicing that is associated with muscle growth, development and metabolism and found that another RNA binding protein 39 (RBM39) plays an important role in maternal obesity-induced fetal muscle growth and development. The Wisconsin and Connecticut Stations plan to study the role of RBM39 in maternal modulation of off-spring growth in rodent an sheep models. The Wisconsin Station has already generated Rbm20 and Rbm39 rodent knockouts and the Connecticut Station has generated maternal over-nutrition and under-nutrition sheep models.
Interleukin-8 is a pro-inflammatory cytokine associated with oxidative stress and obesity, and increased muscle IL-8 is associated with pro-inflammatory conditions such as type 2 diabetes and intense, exhaustive exercise. Interleukin-8 can signal in skeletal muscle, but the effects on myoblast function are currently unknown. The Connecticut Station will determine the effects of IL-8 on myoblast proliferation and differentiation. They hypothesize that IL-8 will alter myoblast proliferation and differentiation via signaling through the MAPK and/or Jak/STAT signaling pathways. To test this hypothesis, they will culture myoblasts in the presence of IL-8, confirm signaling through the Jak/STAT and MAPK signaling pathways, assess proliferation, and quantify differentiation into multinucleated myotubes.
A better understanding of the modes of actions of exogenous growth promotors is needed to speed innovations in this area. New compounds have the potential to improve efficiency and reduce the environmental impact of meat production. However, the method by which growth promotion alters environmental emissions is poorly understood. The Illinois and Texas Tech Stations plan to determine and mechanisms underlying improved growth, efficiency and environmental effects of exogenous growth promotors. This work will take place primarily in cattle and make use of shared methods to determine the effects of exogenous growth promotors on muscle, adipose and whole body metabolism.
Nicotinamide riboside is a vitamin B3 analogue demonstrated to increase mitochondrial biogenesis and NAD+ production. In previous studies, this compound has increased pectoralis major weight and dimensions when it was injected at day 10 of incubation. To determine the mechanisms responsible for this increased growth and development, the Georgia Station will conduct to explore the effect of location and dose on muscle fiber development, the CDK and PI3K pathways at days 15, 18, and 21 of incubation. Additional studies will also be conducted to examine the effects of injection on subsequent growth and Wooden Breast incidence rate.
The Florida Station plans to investigate the impact of stress on mitochondria function/dysfunction in muscles varying in mitochondria content. Moreover, they will examine mitochondria function and substrate metabolism in the context of whole animal energy metabolism (metabolic rate) and efficiency of lean growth. In collaboration with the Alabama Station, they plan to translate this new information into chicken muscle. Moreover, the Iowa Station plans to explore the mechanisms that cause this impairment of degradation and have focused on a lysosomal insufficiency as a contributor caused by a selective exclusion of transcription factor EB (TFEB) from the myonuclei. Their plan is to stimulate TFEB activity via a variety of FDA approved drugs as well as through delivery of the TFEB transgene. Independently, they will determine the extent to which muscle cell health and function may be altered, positively or negatively, by obesity and related insulin resistance.
The Texas Station will analyze muscle from horses, cattle, and swine for mitochondrial number, function (including the novel mitochondrial capacity analysis, high-resolution respirometry), and health, as well as fiber type, fiber size, and cells involved in fiber regeneration or breakdown. Antioxidant status (glutathione peroxidase or superoxide dismutase activities, for example) will be determined in both blood and skeletal muscle. Skeletal muscle from horses, cattle, and pigs will be evaluated for similar measures as above: mitochondrial number, function, and health, as well as fiber type, fiber size, and cells involved in fiber regeneration or breakdown. Investigators from the Texas and Iowa Stations will collaborate to specifically identify the effects of environmental inputs on skeletal muscle mitochondria to determine molecular mechanisms of impaired performance in swine production.
Elevated glycolytic flux provides metabolic intermediates that can be utilized for anabolic muscle growth. A decrease in flux or mislocalization of glycolytic enzymes at their normal site of action within sarcomeres alters the ability of the muscle cell to produce biomass. The Kansas Station will determine the mechanism whereglycolytic enzymes maintain their subcellular distribution, which metabolic constituents are necessary for cell growth, and which upstream signals control this regulation.
The Idaho Station will assess the impacts of bioactive compounds on the regulation of cellular pathways associated with muscle growth. These bioactive compounds may include but are not limited to polyamines, antioxidants, amino acids and or growth promoters.
The New Jersey Station will conduct metabolomics and use stable isotopes to measure metabolic flux (i.e., the rate of turnover of molecules through a metabolic pathway) in the skeletal muscle of animals. These experiments will focus on amino acid metabolism in response to nutritional status and/or exercise. Nutritional status will be altered by diet, drug or genetics and exercise paradigms will include treadmill and running wheel. Measurements of relevant signaling proteins and their activity will be conducted as appropriate. Rodents models will primarily be used. As appropriate, the Standardbred horse will also be used as model organism to understand muscle metabolism during and after exercise.
The Michigan Station will use transcriptomics to characterize the influence of maternal gestational environment and calf management system on subsequent steer performance and carcass composition. A single genetic source will be used to determine the interaction of the genetic line and management system. Transcriptional profiles of two different muscles will be used with blood metabolites and ultrasonography to identify the timing and extent of muscle and fat accretion. Additionally, immunohistochemistry will be utilized to determine development of muscle fiber size and type.
Objective 3. Characterize mechanisms of protein synthesis and degradation in skeletal muscle.
The New Jersey Station will use of stable isotopes to measure of fractional muscle protein synthesis rate and estimate muscle growth. At the same time, a global view of the translatome will be captured by isolating and sequencing ribosome protected RNA as an indicator of what transcripts are being synthesized. This method, referring to as ribosomal profiling or Ribo-seq can be used in concert with metabolic tracers to construct the translatome in response to nutrients and environmental stress. Using these approaches, the New Jersey Station will explore mechanisms by which diet alters skeletal muscle protein synthesis and skeletal muscle protein degradation in the control of overall protein homeostasis. Experimental animals will ingest deuterium oxide-labeled water and the measurement of deuterium enrichment of alanine in muscle will be used to calculate skeletal muscle protein fractional synthesis rate as previously detailed. Muscle tissues will also be used to perform Ribo-seq to construct a global view of the translatome. Control of protein degradation will focus on the regulation of lysosomal processes (i.e., autophagy, chaperone mediated autophagy) and the ubiquitin proteasome pathway by diet and environmental stress. Biochemical measurements of mRNA and protein levels will be complimented by histochemical approaches and electron microscopy to visualize subcellular processes.
A balance between protein synthesis and degradation is crucial in muscle mass homeostasis and is mainly regulated by the ubiquitin-proteasome and autophagosome-lysosome pathways. To better understand how this balance is achieved, the Kansas Station will continue to identify muscle proteins that are turned over by the proteasome in normal physiological conditions and whose degradation may be compromised in atrophy or disease conditions. In a separate project, we will characterize target proteins that are subjected to autophagic disposal during normal muscle contraction. Anticipated collaborations with other stations include examining muscle hypertrophy or atrophy in meat production animals.
The Florida Station plans to evaluate reactive oxygen species production and oxidative stress and impact on muscle protein degradation and growth. Further, they plan to continue exploitation of the Brahman and Angus differences in heat tolerance and examine protein synthesis and degradation pathways under normal and long-term heat stress to determine breed-specific acclimatization to heat stress. Specifically, they will assess protein degradation by calpain-calpastatin system and other mechanisms, and its relationship to muscle growth, as well as its impact on postmortem protein degradation and tenderness development in meat.
Emphasis has been placed on identifying regions of the genome that harbor genes for traits that directly impact the consumer, such as marbling, tenderness, and other quality traits. Dark cutting beef cost the beef industry over 100 million dollars annually. While classically contributed to pre-harvest stress, some indications suggest the defect may not be as simply as an abbarrant bout of exercise. The Texas Station will investigate molecular mechanisms that affect post-mortem beef quality factors including meat tenderness and “dark cutting” occurrence through genomic and proteomic approaches. The Texas Station previously has identified differences in genetic pathways thought to be associated with differential rates of protein turnover in individuals that results in differential response to electrical stimulation of carcasses and subsequent differences in shear force. In addition, across contemporary groups of steers from the genetic mapping population, phenotype data related to dark cutting – pH, color, shear force, etc., and longissimus biopsies have been collected. The Virginia Station will work collaboratively with the Texas Station to validate molecular studies with tissue-based studies. Current and future studies involve examination of high throughput sequencing of microRNA and mRNA to characterize the role of RNA regulatory factors and stress response on carcass characteristics and post-mortem degradation.
Conventional beef production systems and dairy production systems exhibit differences in muscle fiber type, muscle fiber size, and extent of marbling development. However, the relative effect size of genetics and management system is unknown. Fed cattle originating from dairies compose nearly 20% of annual harvest (Eastwood et al., 2017), so greater attention has been placed on maximizing value of male dairy calves by crossbreeding low producing dairy cows to beef sires. The dairy production system (in comparison to range beef production) introduces concentrates to calves at a much earlier age and reduces mobility during calfhood. The Michigan Station is studying the long-term effects of these management practices on the growth curve and carcass performance of steers remain unknown.
The Virginia Station is using IUGR in pigs as a model as well. Specifically, they are comparing low and normal birthweight pigs understand how myofiber numbers and fiber type influence protein turnover. Their hypothesis is that myofiber numbers and fiber types in low birthweight pigs limit muscle growth through an increase in protein turnover and results in increased residual feed intake. They will continue to explore how: 1) myofiber numbers and fiber type affect residual feed intake; and 2) myofiber characteristics influence residual feed intake in pigs.
Measurement of Progress and Results
Outputs
- • Research contained within this project will result in the education of future scientists and researchers in good animal-based scientific approaches to explore factors regulating skeletal muscle growth and development in meat-producing animals.
- • Increased knowledge of the physiological and molecular pathways responsible for the nutrient programming in meat animals.
- • Increased understanding of the role of the extracellular matrix in regulating meat animal growth and product quality.
- • Establish those autocrine, paracrine and endocrine mechanisms by which growth factors regulate proliferation and differentiation of skeletal muscle cells in meat animals.
- • Increased understanding of the role of protein accretion in facilitating skeletal muscle growth.
- • Increased understanding of how heat stress impacts muscle growth.
Outcomes or Projected Impacts
- • Knowledge, in the form of refereed publications, will remain the primary outcome of this project. Collectively, the impact of this knowledge will result in improved genetics and management strategies that should translate into greater lean meat production across the agricultural sector.
- • Graduate students with an indepth understanding of those mechanisms responsible for skeletal muscle growth will increase the capacity of land grant institutions and allied industries to support more effectively animal agriculture.
- • The Committee will sponsor a Symposium on Molecular Mechanisms Regulating Skeletal Muscle Growth and Differentiation. This symposium will provide an avenue to disseminate novel discoveries and increase the visibility of the project.
- • Increased understanding of the impact of nutrient programming on off-spring will result in biological targets for assessing and prescribing maternal nutrient requirements that will benefit producers.
- • Increased understanding of how skeletal muscle responds to a heat event will lead to more robust selection schemes for the creation of more resilent genotypes for meat animal production.
- • Increased knowledge of the cause(s) of Wooden breast will eliminate this biological defect and allow the poultry industry to recuperate millions of dollars lost annually.
- • Elucidation of mechanisms responsible for muscle protein degradation may provide information necessary to utilize molecular- or cell biology-based methods to reduce protein degradation in growing muscle. This will increase muscle growth without increasing nutrient inputs.
- • Multi-omic based results will reveal novel genes and their products, which will lead to additional areas of inquiry that will ultimately advance production efficiency well into the next decade and beyond.
Milestones
(2022):Sponsor a symposium at ASAS National Meeting on Cellular Mechanisms Regulating Skeletal Muscle Growth and the Impact on Sustainable Agriculture. This will allow us to convey research findings to interested individuals in these organizations.(2022):Greater federal funded of members.
(2022):Greater collaboration among members..
(2022):Resolution of the mechanism for Wooden Breast Syndrome for the poultry industry.
(2022):Discovery of alternative mechanisms for meat tenderization.
(2022):Greater membership.
Projected Participation
View Appendix E: ParticipationOutreach Plan
Dissemination of the results of the research will be accomplished through timely publication of refereed articles in established journals. Moreover, graduation of students with increased capacity to understand those biological pathways responsible for augmenting animal growth and productivity will lead to greater outreach to various stakeholders and(or) group of stakeholders. The combination of greater knowledge and enhanced capacity will ultimately lead to improved efficiency of lean meat production in domestic livestock and poultry. Additionally, the committee will organize a symposium on Cellular Mechanisms Regulating Skeletal Muscle Growth and the Impact on Sustainable Agriculture at the ASAS National Meeting that will convey recent research findings to the members of these organizations.
Organization/Governance
The Technical Committee will consist of at least one representative from each participating unit, appointed as described in the Manual for Cooperative Regional Research, with one representative from each unit designated as the voting member. The North Central Regional Association of Directors will appoint one Director to serve as Administrative Advisor and a representative of the USDA/CSREES will also serve as an Advisor; both will be ex officio member of this committee. The Technical Committee will elect a Chair and a Secretary to serve for a period of one year, and these officers will continue to be voting members. In succeeding years, the Secretary will become Chair, and only a new Secretary will be elected. The Chair, the Secretary, the immediate Past Chair and the Administrative Advisor will serve as the Executive Committee, and they will have the authority to act on behalf of the Technical Committee during the periods between meetings. Because this is a proposal for renewal of the NC-1184 project, the current Chair will serve as Past Chair for the first year of the revised project and the current Secretary will become Chair if the renewal is approved.
The Chair, with approval of the Administrative Advisor, will call yearly meetings of the Technical Committee and interim meetings of the Executive Committee if needed. A report of research results from each unit will be presented orally and in writing at the Technical Committee meetings. The written report should include a list of publications resulting from research related to the project for the year. Reports will be critically reviewed by the Technical Committee and recommendations will be made for future research and coordination of research between units to maximize attainment of the Objectives. The Secretary will prepare minutes and an Annual Report for distribution to Technical Committee members, Directors and Department Chairs of participating State Agricultural Experiment Stations and USDA Laboratories, and the Regional Research Office, CSREES. Because submission of a yearly progress report is essential to assess progress, ensure participation, and prepare the Annual Report, any unit that does not submit a written progress report for two consecutive years will be considered not to be participating and will be dropped from the project.
A station, agency, or institution not currently listed as a participant in the project can petition to join by submitting an addendum to the Methods section of this proposal. This addendum should describe the proposed work and how it contributes to the project. The Administrative Advisor will circulate the addendum to the voting members of the Technical Committee for their consideration and approval. If approved by a majority of the voting members of the Technical Committee, the addendum will be added to the official project outline.
Literature Cited
Allen RE and LK Boxhorn. 1989. Regulation of skeletal muscle satellite cell proliferation and differentiation by transforming growth factor-beta, insulin-like growth factor I, and fibroblast growth factor. J. Cell. Physiol. 138:311–315.
Bottje, WG. 2019. BOARD INVITED REVIEW: Oxidative stress and efficiency: the tightrope act of mitochondria in health and disease,, J. Anim. Sci. 97(8):3169–3179.
Bradley SG, Kelleher AR and SR Kimball. 2013. Regulation of muscle protein synthesis and the effects of catabolic states. Int. J. Biochem. Cell Biol. 45(10):2147-2157.
Buckingham M. 2001. Skeletal muscle formation in vertebrates. Curr. Opin. Genet. Dev. 11:440–448.
D’Angelo MA, Raices M, Panowski SH, and MW Hetzer. 2009. Age-dependent deterioration of nuclear pore complexes causes a loss of nuclear integrity in postmitotic cells. Cell. 136(2):284–295.
Dolmetsch RE, Lewis, RS, Goodnow CC, and JI Healy. 1997. Differential activation of transcription factors induced by Ca2+ response amplitude and duration. Nature 386:855–858.
Du M, Tong J, Zhao J, Underwood KR, Zhu M, Ford SP, and PW Nathanielsz. 2010. Fetal programming of skeletal muscle development in ruminant animals. J. Anim. Sci. 88(13):E51–E60.
Dwyer CM, Fletcher JM and NC Stickland. 1993. Muscle cellularity and postnatal growth in the pig. J. Anim. Sci. 71:3339–3343.
Dwyer CM, Stickland NC and JM Fletcher. 1994. The influence of maternal nutrition on muscle fiber number development in the porcine fetus and on subsequent postnatal growth. J. Anim. Sci. 72:911–917.
Eastwood LC, Boykin CA, Harris MK, Arnold AN, Hale DS, Kerth CR, Griffin DB, Savell JW, Belk KE, Woerner DR, et al. 2017. National Beef Quality Audit-2016: transportation, mobility, and harvest-floor assessments of targeted characteristics that affect quality and value of cattle, carcasses, and by-products. Transl. Anim. Sci . 1:229–238.
Garcia-Roves PM, Osler ME, Holmström MH, and JR Zierath. 2008. Gain-of-function R225Q mutation in AMP-activated protein kinase gamma3 subunit increases mitochondrial biogenesis in glycolytic skeletal muscle. J. Biol. Chem. 283(51):35724-34.
Goll DE, Neti G, Mares SW, and VF Thompson. 2008. Myofibrillar protein turnover: The proteasome and the calpains,, Journal of Animal Science, 86(14) E19–E35.
Hall MN, Corbett AH, and GK Pavlath. 2011. Regulation of nucleocytoplasmic transport in skeletal muscle. Curr. Top. Dev. Biol. 96:273–302.
Hart GW, and Y Akimoto . 2009. The O-GlcNAc Modification. In: Varki A, Cummings RD, Esko JD, et al., editors. Chapter 18. Essentials of Glycobiology. 2nd edition. Cold Spring Harbor (NY): Cold Spring Harbor Laboratory Press.
Jamali T, Jamali Y, Mehrbod M, and MRK Mofrad. 2011. Nuclear pore complex: biochemistry and biophysics of nucleocytoplasmic transport in health and disease. Int Rev. Cell Mol. Biol. 287:233–286.
Joe AW, Yi L, Natarajan A, Le Grand F, So L, Wang J, Rudnicki MA, and FM Rossi. 2010. Muscle injury activates resident fibro/adipogenic progenitors that facilitate myogenesis. Nat Cell Biol. 12(2):153-63.
Le Grand F, and MA Rudnicki. 2007. Skeletal muscle satellite cells and adult myogenesis. Curr. Opin. Cell Biol. 19(6):628–633.
Liu N, Garry GA, Li S, Bezprozvannaya S, Sanchez-Ortiz E, Chen B, Shelton JM, Jaichander P, Bassel-Duby R, and EN Olson EN. 2017. A Twist2-dependent progenitor cell contributes to adult skeletal muscle. Nat. Cell Biol. 19:202–213.
Mauro A, 1961. Satellite cell of skeletal muscle fibers. J. Biophys. Biochem. Cytol. 9:493–495.
Potthoff MJ, Wu H, Arnold MA, Shelton JM, Backs J, McAnally J, Richardson JA, Bassel-Duby R, and EN Olson. 2007. Histone deacetylase degradation and MEF2 activation promote the formation of slow-twitch myofibers. J. Clin. Invest. 117:2459–2467.
Saito T, and J Sadoshima. 2015. Molecular mechanisms of mitochondrial autophagy/mitophagy in the heart. Circ Res. 116(8):1477–1490.
Savas JN, Toyama BH, Xu T, Yates JR, and MW Hetzer. 2012. Extremely long-lived nuclear pore proteins in the rat brain. Science. 24:335(6071):942.
Scheffler TL and DE Gerrard. 2016. GROWTH AND DEVELOPMENT SYMPOSIUM: Adenosine monophosphate–activated protein kinase and mitochondria in Rendement Napole pig growth, J. Anim. Sci. 94(9):3601–3612.
Schiaffino S, and C Reggiani. 2011. Fiber types in mammalian skeletal muscles. Physiol. Rev. 91:1447–1531.
Stark DA, Coffey NJ, Pancoast HR, Arnold LL, Walker JP, Vallée J, Robitaille R, Garcia, ML, and DD Cornelison. 2015. Ephrin-A3 promotes and maintains slow muscle fiber identity during postnatal development and reinnervation. J. Cell Biol. 211:1077–1091.
Suzuki A, H Tamate, and M. Okada. 1976. The effect of a high plane of nutrition during a given period of growth on size and proportion of skeletal muscle fiber types in the cattle. Tohuko J. Agric. Res. 27:20–25.
Thompson J. 2004. The effects of marbling on flavour and juiciness scores of cooked beef, after adjusting to a constant tenderness. Aust. J. Exp. Agric. 44(7):645-52.
Thornton, KJ, Kamange-Sollo E, White ME, and WR Dayton. 2015. Role of G protein–coupled receptors (GPCR), matrix metalloproteinases 2 and 9 (MMP2 and MMP9), heparin-binding epidermal growth factor–like growth factor (hbEGF), epidermal growth factor receptor (EGFR), erbB2, and insulin-like growth factor 1 receptor (IGF-1R) in trenbolone acetate–stimulated bovine satellite cell proliferation, J. Anim. Sci. 93(9):4291–4301.
Velleman SG, Shin J, Li X and Y Song. 2012. Review: The skeletal muscle extracellular matrix: Possible roles in the regulation of muscle development and growth. Can. J. Anim. Sci. 92:1–10.
Sandra G. Velleman,1 Jonghyun Shin,1 Xuehui Li,2 Yan Song1 1Ohio Agricultural Research and Development Center/The Ohio State University, Department of Animal Sciences, Wooster, OH 44691, USA (email: ); Velleman.1@osu.edu 2University of Florida, Department of Anatomy and Cell Biology, Gainesville, FL 32610, USA Velleman, SG. 2015. Relationship of Skeletal Muscle Development and growth to breast muscle myopathies: A Review Avian Dis. 59(4):525-31.
Wu G, Bazer FW, Wallace JM, and TE Spencer. 2006. Board-invited review: intrauterine growth retardation: implications for the animal sciences. J. Anim. Sci. 84(9):2316-37.