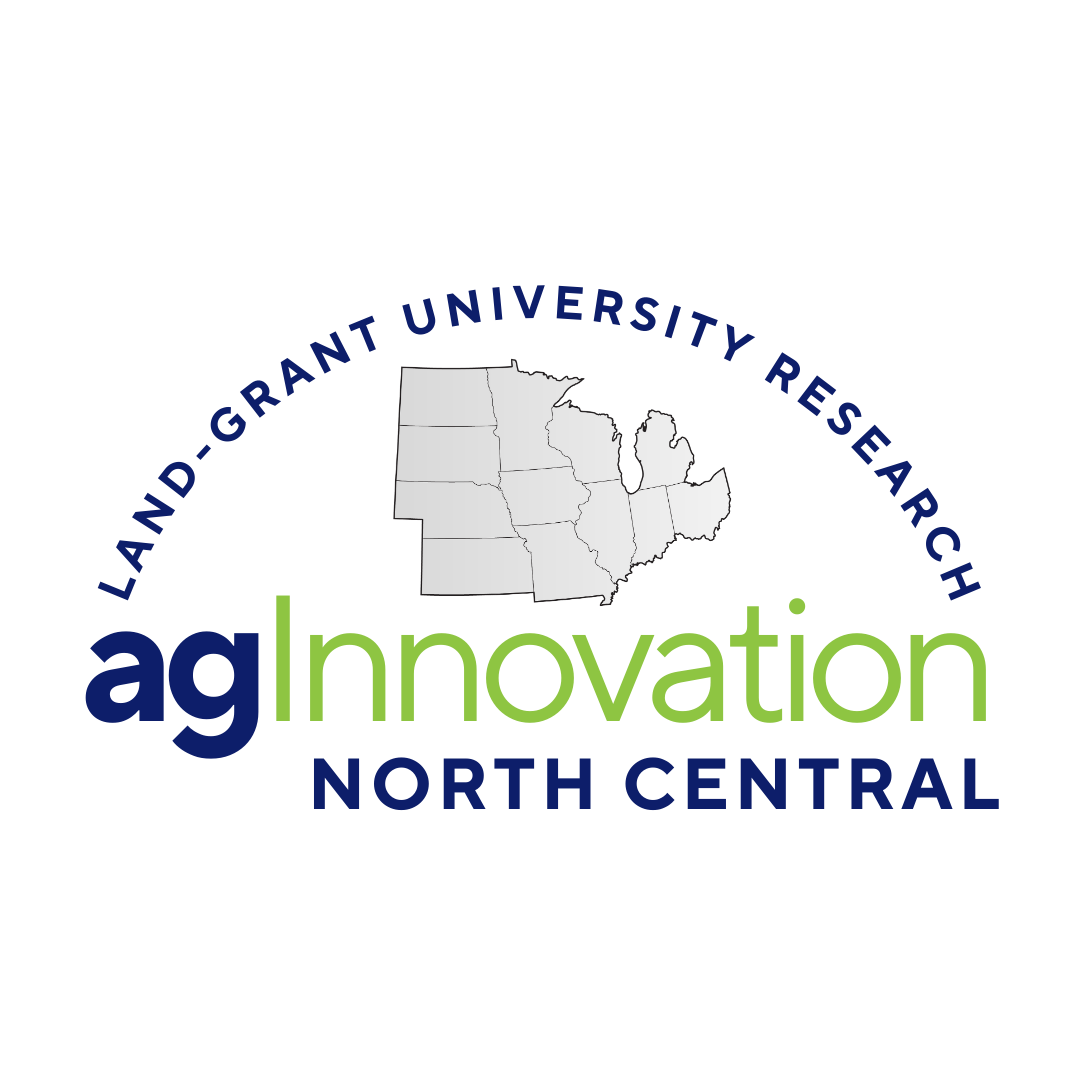
NC1187: Particulate Reactivity and Cycling in a Changing Environment: Implications for Agriculture and Human Health
(Multistate Research Project)
Status: Active
NC1187: Particulate Reactivity and Cycling in a Changing Environment: Implications for Agriculture and Human Health
Duration: 10/01/2020 to 09/30/2025
Administrative Advisor(s):
NIFA Reps:
Non-Technical Summary
Statement of Issues and Justification
Many ecosystems are becoming increasingly stressed, and resources needed for agriculture, such as fertilizers and water, are becoming more costly to access and process. To produce enough food for the increasing population, we must get more food from already strained soil and water resources. Exacerbating these challenges, with climate and environmental change, we are seeing more extreme events including flooding, sea level rise, and salt water intrusion of drinking water. These events are having profound impacts on both agricultural and non-agricultural areas. They affect biogeochemical processes in soils, including contaminant (metals, metalloids, nutrients, and microbial) mobility and cycling. One of the most promising ways to make significant technological breakthroughs in agricultural and environmental sciences is fundamental research on biological, physical, and chemical processes occurring in natural and managed ecosystems (Augustine and Lane, 2014; Huang et al., 1998; Sposito et al., 1992).
Fate and availability of plant nutrients and environmental toxins depends on reactions with particles with diameters of tens of micrometers or less (silt, clays, microbes, nanoparticles) contained in a very complex mixtures within soils. Because of this complexity, it is often difficult to gain a mechanistic understanding of processes governing the retention and mobility of chemicals. Mechanistic understanding of chemical reactions in soils that control nutrient and contaminant mobility and bioaccessibility requires using advanced spectroscopic and microscopic instruments. The situation may be likened to the current ability to characterize microbial communities in natural systems by the analysis of genetic material. Without the application of molecular tools, we will not have the required knowledge to advance agricultural systems to minimize inputs while optimizing production and economy.
Soil degradation is a serious worldwide challenge affecting land productivity, water quality, and soil erosion (Brussaard and van Faassen, 1994; Schrader et al., 2007; Roose et al., 2016; Udawatta et al., 2008a). During soil degradation, microstructure is damaged and soil aggregates are consolidated decreasing soil porosity; and subsequently altering water, heat, and gas transport as well as soil productivity (Kim et al., 2010; Udawatta et al., 2008a, 2008b, 2012, 2016). Assessment of geometrical soil pore parameters and their direct effects on water transport is a fundamental way to evaluate environmental impacts of land management operations on soils and land productivity. Soil scientists examine microstructure of the soil system to better predict water, chemical, and gas movement; water quality; root nutrient uptake, and soil erosion to assess the effects of management on soil pore parameters and microbial habitats, as well as to evaluate treatment effects on land productivity (Anderson and Hopmans, 2013). Microstructure governs the flow of resources through the pore space of the soil media and creates spatial and temporal variations in the media (Young and Crawford, 2004; Zhang et al., 2005). A good understanding of pore parameters including pore dimension, pore size distribution, connectivity, shape factors, and tortuosity as well as distributions or probabilities of these parameters are critically important to issues related to movement of microfauna, water, solutes, and gases as well as soil erosion (Ioannidis and Chatzis, 2000; Lindquist, 2002; Udawatta et al., 2008a, 2008b, 2012, 2016; Roose et al., 2016).
Society in general will benefit through a better understanding of how soil health improves using integrated soil physical properties and processes as well as assessing soil management systems for improved water use efficiency. When we are better able to understand how soil health influences water infiltration and soil plant water availability, we will be better able to predict the influence of soil management on sustainable plant productivity. The agricultural community will benefit directly through improvement of soil health from enhanced water infiltration and plant available water storage. Scientific knowledge will also be advanced with a better understanding of how pollutants move on a macropore-scale in soils.
Efficient use of soil water is critical for grain crop, pasture, and biofuel production and soil health in agriculture. Within soils, biopores are essential for enhancing water infiltration to recharge plant available water and for maximizing storage of plant available water. Thus, studying biopores as influenced by management will assist in recommending sustainable practices for optimizing water infiltration and efficient storage of plant available water. Soils are quite variable as to their physical properties and structure. Good soil structure, which includes biopores, enhances water infiltration and decreases surface water runoff thus improving the productive capacity of the soil and enhancing surface water quality. Gas transport in soils is also highly dependent upon soil structure. Use of the techniques developed in this proposal will assist land managers by identifying management techniques which improve soil structure and associated water transport properties.
Soil is a key component of terrestrial ecosystems that contains almost twice as much carbon (C) as atmosphere; and it is the C which can be easily lost under right environmental settings or, alternatively, can be increased by appropriate management. While research community has achieved substantial progress in deciphering the mechanisms driving soil C gains, abundant unexplained examples of unexpected outcomes signal that our understanding is incomplete. For example, some soils accumulate C even in the presence of aggressive tillage, while soils of some perennial systems can be slow to gain it despite the lack of soil disturbance and large belowground C inputs. Such discrepancies suggest a significant knowledge gap with respect to the plant-soil-microbial interactions that drive soil C accretion. This deficiency is especially unfortunate given globally changing climate, since soil C accrual strategies effective under new future environments can be efficiently developed only with a full understanding of underlying drivers. Better understanding of the micro-scale processes driving soil C and greenhouse gas production and emissions likely holds the key to developing management practices optimal for soil health and environmental sustainability.
Although phosphorus (P) is a limiting nutrient in many environmental systems, anthropogenic inputs have accelerated terrestrial P flows approximately three times their indigenous rates through the application of synthetic and animal based-fertilizer (Carpenter et al., 1998; Howarth et al., 2002; Liu et al., 2008; Smil, 2000). Long-term use of fertilizer amendments has resulted in the accumulation of P in intensively managed agricultural soils in the U.S. (Foley et al., 2005; Gronberg and Arnold, 2017; Sims et al., 1998). To reduce the occurrence of a variety of environmental issues, including the growth of populations of toxic cyanobacteria and Pfiesteria, eutrophication, and the development of hypoxic zones (Burkholder and Glasgow, 1997; Diaz and Rosenberg, 2008; Dodds, 2006), various management practices have been focusing on control technologies (e.g., buffer strips and cover crops) in key watersheds in each state. However, there is great uncertainty about the temporal distribution of particulate P at the soil-water/atmosphere interface under extreme weather conditions.
Elevated levels of metal(loid)s such as As, Cr, and Pb due to legacy industrial operations and other anthropogenic activities, and nutrients like P, due to intensive animal agriculture, are prevalent in coastal, sandy soils as well as urban areas. For example, in the Delmarva area, due to sea level rise and increased irrigation, salt water intrusion of groundwater and salt deposits on crop land are being observed. There is a lack of information on how cycling of contaminants are affected by salinity, due to sea level rise and salt water intrusion. There could be significant impacts on human health, land use, agricultural sustainability, and tourism. Fundamental processes that could drive the release and cycling of the contaminants and nutrients bound to soils and sediments in response to sea level rise are 1) salinization, 2) rising water tables, and 3) bottom stress induced by flooding and inundation of coastal areas. The salinity of soil and groundwater is likely to increase as saltwater fronts migrate inland, flooding becomes more widespread, and coastal storm surges increase in frequency and intensity. Although recent studies (Burton et al., 2008; LeMonte et al. 2017) have raised the alarm, very little research has been done to investigate the joint risk of sea level rise and soil contaminants. Saltwater intrusion could enhance the release and mobility of contaminants and lead to ion-exchange, sorption/desorption, and/or dissolution processes that alter contaminant speciation. Moreover, due to the bottom stresses induced by flooding, waves and currents, the sediments in coastal areas are often subject to episodes of physical disturbances and sediment resuspension (Kalnejais et al. 2007, Cantwell et al. 2002). When sediments are resuspended, changes in redox occur, resulting in potential alteration of many contaminants’ solid-phase speciation. Following resuspension of anoxic sediments into the water column, the potential exists for contaminants to be released from the particulate phase and increase in bioavailability due primarily to oxidation processes and changes in pH. Currently little is known about the effects that different resuspension energies have on the contaminants’ mobility and speciation in solution and solid phase. Our findings will provide valuable information regarding how contaminant cycling and speciation are impacted by sea level rise and flooding.
Particulate matter in the air of rural and agricultural regions has a complex array of sources that are both primary (direct emissions) and secondary (formed in the atmosphere from reactions of gaseous chemicals). In order to understand the context of agricultural emissions contributing to particulate matter and the effects of particulate matter on agricultural production, air quality, human health, and climate, more information is critically needed on the primary and secondary particle sources. Some of our members focusing on studying particulate matter in the air including airborne microorganisms. Investigation of the presence of airborne microorganisms (bioaerosols) in the ambient air is of interest due to their environmental and human health effects (Burge, 1990; Douwes et al., 2003). Numerous studies have shown that increased exposure to bioaerosols is positively correlated with incidence of negative respiratory health effects, including lung irritation, asthma, rhinitis, allergy, and cough (Burge, 1990; Douwes et al., 2003; Fung and Hughson, 2003; Herr et al., 2003). Bioaerosol related issues could be especially acute in agricultural environments, where high airborne microorganism concentrations could be encountered. The presence of bioaerosols in agricultural environments is different from their presence in other environments, because here bioaerosols are known to transmit plant diseases (Parker et al. 2013; West and Kimber, 2015), and, likely, animal diseases.
To determine the presence of any pathogenic or non-pathogenic microorganism in the air of agricultural facility, airborne microbiological particles must be sampled, followed by their analysis by culturing (Herr et al. 2003), microscopy, flow cytometry, ATP-based bioluminescence, quantitative polymerase chain reaction (qPCR), gene sequencing and other methods (Górny 2020; Reponen et al. 2011). It has long been recognized that the sampling process itself negatively affects the microorganism culturability, viability and cell-wall integrity because of the sampling stress to the microorganisms. While numerous bioaerosol collectors exist, it has long been recognized that the current bioaerosol sampling and assessment techniques have limitations and need improvement and development (Walser et al., 2015).
The goals of this multistate project will be multiple: one, help our multistate group members and scientific community members to access and use of advanced analytical techniques, second, advance sample collection and/or preparation methods, data collection methods, data analysis, and interpretations. Third, is to characterize the physical, chemical, biological and morphological properties of particulate matter and their agricultural, environmental, human health and economic impacts over a wide range of spatial and temporal scales, including their potential effects on ecological sustainability, food and energy production, climate change, air and water quality, soil health, and human health. Working within a multistate research group while performing the work under one or more objectives offers a unique advantage to our members through formal and informal collaborations and continuous information and knowledge sharing.
A common thread that runs through much agricultural and environmental research is the importance of understanding processes that operate on different spatial and temporal scales. The technical feasibility of applying advanced analytical methods to a wide range of sample sizes and chemical compositions is amply supported by the current scientific literature. The utilization of a combination of techniques to accomplish full characterization of natural systems and to relate these properties to behavior in complex systems has become increasingly important and successful. To do this, NC1187 is a workgroup composed of scientists specializing in utilization of advanced analytical tools to investigate particles that occur in air, soil, and water.
Significant advances in technologies related to spatially resolved microscopic and spectroscopic molecular characterization methods have resulted from advances in optics, focusing devices, and detectors; and because of greater availability of high brilliance synchrotron facilities world-wide (Fenter et al., 2002; Kelley et al., 2008; Lombi and Susini, 2009; Singh and Grafe, 2010), such as the Advanced Photon Source (APS), the Advanced Light Source (ALS), Stanford Synchrotron Radiation Lightsource (SSRL), the National Synchrotron Light Source II (NSLS-II) and others around the world. At these facilities, spatially resolved synchrotron-based X-ray fluorescence (XRF) and X-ray absorption fine structure (XAFS) spectroscopy are providing microscopic and molecular level information on soil systems not previously available using other techniques. While there exists a variety of micro-analytical techniques that have long been used in these disciplines, synchrotron-based spatially resolved XRF, XANES, scanning tunneling X-ray microscopy (STXM), EXAFS spectroscopy, and X-ray diffraction (XRD) are emerging as important methods that complement characterization by traditional techniques.
Advanced analytical techniques are also at available at national labs, such as the Environmental Molecular Science laboratory (EMSL), a national scientific user facility at the Pacific Northwest National Laboratory that has a number of instruments useful for the studying soils and sediments. Specialized instrument facilities, funded by the National Science Foundation and located at universities around the country, also have instruments that can assist in meeting the basic research needs of NC1187.
Long-term solutions to natural resource utilization and management requires basic research focusing on understanding fundamentals of processes governing innovations in food, energy and water science. To address the modern challenges of food production, and decrease use of water and energy resources, NC1187 will pursue the following objectives:
Objective 1: Help multistate members and scientific community members access and use advanced molecular and microscopic tools.
Objective 2: Educate about and help prepare sample preparation methods used in pure systems for advanced molecular and microscopic analysis of soil-plant-water and air systems so that fundamental information on these complex systems can be discovered.
Objective 3: Characterize the physical, chemical, biological and morphological properties of particulate matter and their agricultural, environmental, human health and economic impacts over a wide range of spatial and temporal scales, including their potential effects on ecological sustainability, food and energy production, climate change, air and water quality, soil health, and human health.
The demand for increased food production, water, and securing the health of the environment have created a research challenge for the current and next generation of scientists. To meet these demands NC1187 members will use advanced instruments to do molecular and microscopic level analysis and collaborate to train members and make these tools available for such analysis.
There are several advantages in doing this project as a multistate effort. First, the central focus of this project (integrated modern instrumentation, including synchrotron microspectroscopy) demands extensive cooperation among members: sharing experience with specific facilities and analytical techniques, and sharing disciplinary expertise (soil, water, and air chemistry, microbiology, physics, etc.). Second, this project will promote the use of synchrotron sources to push the envelope in terms of micro-spectroscopic characterization of particles and will integrate synchrotron techniques with other state-of-art-tools provided by national labs and universities (Coward et al., 2019; Pitumpe Arachchige et al, 2018; Sowers et al, 2018). Third, project participants will share samples and data for multiple analyses, and will work to produce an integrated investigation sample set or experimental site.
In the past, synchrotron research by soil scientists primarily focused on industrial contaminants, i.e., metal(loids). There are many opportunities now with micro-focused techniques to study elements like carbon and organic matter dynamics, and nitrogen dynamics which are critical in addressing global climate change, and phosphate and other macro and micronutrients transformations and fate in soils. This approach will link more basic soil science with applied soil science, particularly in the area of nutrient management, climate change, and soil health. The members have already established an excellent record of multistate collaboration and technological information sharing and the members anticipate to continue their collaborations.
This project will enhance our ability to assess the impact of micro- and submicron-sized particles on processes taking place in agricultural and natural ecosystems by elucidating links between particulate (physical, biological and chemical) properties and their role in the sustainability, productivity and health of those systems. Research activities coordinated under this project will result in a catalog of physical and chemical properties of particulates related to agriculture production and of evaluations of the rate and transfer mechanisms of particulates through the environment causing environmental degradation. This project will also improve our ability to monitor exposures to airborne particulates including biological agents in a variety of environments. Improved understanding of particulate and bioaerosol sampler performance and guidelines reg. their integration with molecular analysis tools will enable more accurate exposure estimates. Better understanding of fate and transfer of contaminants and exposure estimates of airborne particulates will lead to informed decisions and better protective and control measures thus contributing to the protection of resources and populations at risk.
A greater number of scientists from state agricultural experiment stations will be utilizing the advanced analytical facilities funded by DOE and NSF to address important questions related to agricultural production, and soil, human and environmental health protection. This will lead to the development of a better understanding of the behavior of pollutant and nutrient elements and compounds associated with fine particles in soil, water and air.
Related, Current and Previous Work
A CRIS search was conducted. Though other multistate projects examining soil, agriculture, and human health do exist, there is no overlap with this project. None of the current projects do focus on investigating fundamental of particulate matter cycling and reactivity using advanced analytical techniques, Projects with some aspects broadly similar to the proposed NC1187 activities include:
W4188: Soil, Water, and Environmental Physics to Sustain Agriculture and Natural Resources
W3170: Beneficial Reuse of Residuals and Reclaimed Water: Impact on Soil Ecosystem and Human Health
Members of this project are applying a wide range of analytical tools to elucidate mechanisms of physical and chemical protection of carbon in soils, redox cycling of iron, cycling and reaction pathways of nitrogen, phosphorus and other nutrients in soils, micro-scale hot-spots of greenhouse gas production within soil pore structure, colloid transport through soil, removal and in situ stabilization of soil contaminants, effect of climate change on soil structure, storage and transport of soil water and contaminant mobility, detection of dense non-aqueous phase liquids (DNAPLs) in geomedia, testing a few different wastewater treatment processes that will supply unrestricted reuse water, will recycle nutrients, and will sequester carbon in soils to help mitigate greenhouse gas increases in the atmosphere, time-resolved simultaneous measurement of atmospheric aerosols, and fate and effects of airborne particulates.
Several members of our group (Anderson, Gimenez, Kravchenko) are working on various aspects of imaging and quantification of pore systems in soils. Computed microtomography (mCT) can be viewed as a technique in soil studies that enables examination of local variation (μm-scale), whereas conventional computed tomography (CT) enables examination at a mm-scale (Anderson and Hopmans, 2013). Udawatta et al. (2013) have described the relative advantages of CT and mCT procedures on soil images. Advantages of mCT procedures include repeated examination of interior structural features at μm-scale resolution within three dimensions, measurement of connectivity and tortuosity, nondestructive evaluation of sample interiors retaining connectivity and spatial variation in pores, as well as enabling examination of dynamic soil processes and quantification of pore geometry (Udawatta et al., 2008a). mCT has been used in examination of pores in sealing materials for containing nuclear waste as well as in rock and soil media for evaluation of fluid and chemical transport (Udawatta et al., 2008a). Additionally, pore geometry has been evaluated to quantify management effects on root nutrient uptake, bacteria, rhizosphere, and fluid flow model simulations. Quantitative information of soil microstructure is required to improve our understanding of infiltration, contaminant movement through porous media, root nutrient uptake, land productivity, and quantification of model parameters associated with fluid and gas movement (Wildenschild et al., 2002; Assouline, 2004). We believe that experiments and simulation or building of models must go hand in hand to better understand pore scale soil processes and their relationships to environmental and production benefits.
Transport and retention colloids (nano- and micro-sized particles) in porous media through column breakthrough experiments have been conducted by members of this group. The analytical tools used in their studies include in-situ pore-scale together with microscopy (e.g., bright field microscope, TEM, SEM, atomic force microscope) and spectroscopy (e.g., dynamic light scattering). Engineered nanoparticles (ENPs) are increasingly incorporated into many industrial and consumer products (Kah et al., 2013; NRC, 2012). Previous work by our group members investigated the aggregation and transport of ENPs (Bouchard et al., 2013; Bouchard et al., 2012; Wang et al., 2014a; Wang et al., 2014b; Zhang et al., 2012; Zhang et al., 2013) as well as clay (Tian et al., 2015), polystyrene (Morales et al., 2013; Sang et al., 2013; Sang et al., 2014; Zevi et al., 2012) and biochar particles (Wang et al., 2013a; Wang et al., 2013b; Wang et al., 2019b) in soil and water environments, as influenced by solution and surface chemistries, and soil structures. We examined fundamental mechanisms governing aggregation and transport of ENPs and colloids in soil and water systems through laboratory aggregation/attachment experiments and column breakthrough experiments aided with in-situ microscopic visualization (Morales et al., 2013; Tian et al., 2015; Zevi et al., 2012; Zhang et al., 2012; Zhang et al., 2013). Experimental observations were explained using surface interaction energy theories (Derjaguin-Landau-Verwey-Overbeek [DLVO] theory or extended DLVO theory), force and torque analyses, and flow hydrodynamics. To date these investigations have shown that the air-water-solid contact line is an important retention site in unsaturated porous media (Sang et al., 2013; Zevi et al., 2012), in addition to the solid-water interface, the air-water interface, and the grain-grain contacts. The transport of ENPs and colloids was facilitated by the presence of humic acid in solution (Wang et al., 2013b), but hindered by root exudates in solution or iron oxide coatings on soil surface (Tian et al., 2015; Wang et al., 2014a; Wang et al., 2013b). These findings have important implications in understanding environmental factors controlling the fate and transport of ENPs and colloids in agroecosystems.
Some members in our group (Hettiarachchi, Kravchenko, Xia) have studied the role of physical and biochemical interactions at micro-scale in governing soil’s contribution to global cycling of carbon and nitrogen. The strength of these research lies in our ability to obtain direct spectromicroscopic evidence in situ, in free soil microaggregates in their native state directly, while preserving the aggregate microstructure (Pitumpe Arachchige et al, 2018). They found preserved less recalcitrant organic carbon species within microaggregates, some stabilized with their original morphology. Strong organo-mineral associations and no-till promoted-stabilization of less recalcitrant organic carbon were also evident. Kravchenko team identified water absorption by decomposing residues to be one of the primary conditions defining occurrence and strength of micro-scale hot-spots of N2O production (Kravchenko et al. 2017; 2018a; 2018b; Kravchenko and Guber, 2017). While the chemical composition of the plant residues and soil moisture and texture have been long known to influence N2O production and emission, Kravchenko’s team also identified additional never before considered factors that affect the magnitude of N2O production and emission from such hot-spots. These factors are soil pore structure and physical characteristics, e.g., porosity, of the plant residue (Kravchenko et al., 2018a; Kutlu et al., 2018). The findings will facilitate improving accuracy in modeling and predicting N2O emissions from agricultural soils and will suggest new management strategies for reducing N2O emissions.
Phosphorus fertilizer use efficiency is poor (10-30% in the first growing season) in many acid and calcareous soils as a result of fixation reactions between the orthophosphate anion and various forms of Ca, Fe or Al that limit the nutrient’s availability to plants. Similar issues do exist for micronutrient fertilizers as inclusion of micronutrients in commercial macronutrient fertilizers is a common practice throughout the world due to practical reasons. The cost of conventional phosphorus and micronutrient fertilizers, yield loss as well as environmental issues due to their inefficient utilization is considerable and therefore it is essential to find new application methods or novel fertilizer technologies that can increase the efficiency of P (Weeks and Hettiarachchi, 2019) and micronutrient acquisition. Our members will continue to investigate the novel fertilizer technologies for both N and P that will enable nutrients to release slowly and/or diffuse further to furnish more plant available nutrients while preventing the environmental losses.
Although P is a limiting nutrient in many environmental systems, anthropogenic inputs have accelerated terrestrial P flows approximately three times their indigenous rates through the application of synthetic and animal based-fertilizer (Carpenter et al., 1998; Howarth et al., 2002; Liu et al., 2008; Smil, 2000). Long-term use of fertilizer amendments has resulted in the accumulation of P in intensively managed agricultural soils in the U.S. (Foley et al., 2005; Gronberg and Arnold, 2017; Sims et al., 1998). To reduce the occurrence of a variety of environmental issues, including the growth of populations of toxic cyanobacteria and Pfiesteria, eutrophication, and the development of hypoxic zones (Burkholder and Glasgow, 1997; Diaz and Rosenberg, 2008; Dodds, 2006), various management practices have been focusing on control technologies (e.g., buffer strips and cover crops) in key watersheds in each state. However, there is great uncertainty about the temporal distribution of particulate P at the soil-water/atmosphere interface under extreme weather conditions. The contiguous United States just experienced one of the wettest winters and springs in 2019 (NCEI, 2019). Phosphorus is generally transported with sediments and soil colloids through surface runoff and subsurface transport in agricultural soils (e.g., Logan et al., 1980; Sims et al., 1998). Several members of our group (Arai, Strawn) are focusing on P transport mechanisms. One of the biggest challenges for improving agricultural productivity and environmental sustainability is accurate prediction of the amount of P that will be transported to surface waters in a particular system. The P loading problems to surface waters occur because nutrient management plans do not correctly account for P loading potential of soils and availability of P for offsite transport. The core of the problem is that the soil tests were designed to monitor P availability for plant nutrient management. Research has shown that on some soils, P availability tests values are correlated with the amount of dissolved reactive P (DRP) transported off-site, which is the phase that directly contributes to eutrophication. However, it is commonly observed that test P is not correlated to total offsite P loading to surface waters. Total P loading includes DRP, particulate and organic P. Although the latter two phases are not directly available for uptake by algae, the P associated with these phases can mineralize, desorb or dissolve, thereby releasing P to the solution phase and contributing to environmentally-available P. The extent to which this occurs is not known, and requires knowledge of P speciation of organic and suspended particles. In the new project our members will investigate speciation of soil P, transport of soil solution and particulate P, and recovery of P for use as fertilizers for waste waters. Results will provide new knowledge that will lead to improved water quality and better nutrient use efficiency in agriculture.
Several members in our group (Hettiarachchi, Schwab, Sparks) are using advanced spectroscopic techniques to study redox processes in soil using advanced analytical techniques, including various synchrotron based spectromicroscopy techniques. Redox cycling of iron (Fe) in soils between Fe(II) and Fe(III) is linked to carbon and energy flow as well as the movement and bioavailability of most contaminants and nutrients. When soils flood with water and oxygen gets depleted, biogeochemical processes cause reductive dissolution of Fe(III) oxide solids into dissolved Fe(II) and neutralize pH. The fate of released Fe(II) is both complex and important to many paired processes and needs to be better understood. Model systems sorbing Fe(II) with synthetic, pure aluminum (Al) hydroxides show that above neutral pH, Al will partially dissolve and coprecipitate with Fe(II) to form Fe(II)-Al layered double hydroxides (LDH). This still remains to be seen in natural clay minerals, soil or the environment but would represent a missing sorbent and redox reactant in critically-important environments such as wetlands, riparian zones and reducing soils. Studies have also shown clear role of Fe oxides on sequestering native soil arsenic (As), as well as other soil contaminants, mobilized under reduced conditions (Galkaduwa et al. 2018; Wu et al., 2015). These results are a promising step in proving the existence of various Fe oxyhydroxides in reducing soil and their impact on contaminant and nutrient cycling in the environment.
Sea level rise (SLR) poses a great threat to coastal As contamination throughout the globe as its impeding effects will alter current soil biogeochemical conditions. Arsenic has a high affinity for iron (Fe) oxides, which will experience reductive dissolution when they are exposed to flooding conditions, which may lead to As release. However, it is not fully understood how As cycling will behave during flooding with seawater. To better understand As cycling in the face of SLR, laboratory-based experiments in model systems were implemented to simulate flooding conditions on As-sorbed Fe oxides, reflective of the contamination found along the Mid-Atlantic coast of the United States. Work has been shown that when As-contaminated soil was collected from coastal Delaware and reduced in either seawater or fresh river water, more arsenic was mobilized in the river water system. The reason for this difference in not entirely known. Possible formation of ternary complexes between arsenate, divalent cations in seawater, and the mineral surface may contribute to greater sequestration in the seawater system (LeMonte et al., 2018). Spark’s group results show that these ternary complexes may be forming in a seawater system when arsenate [As(V)] is sorbed to goethite, a common iron oxide mineral, at a pH above 7. Future experiments are planned to show direct spectroscopic evidence of ternary complexes in a seawater system, which seem to be lacking in the literature.
Members of this group (Anderson, Gimenez, Xia, Zhang) have also been involved in studies on the fate and transport of antibiotics resulting from land application of animal waste, considering that they constitute a significant concern in agroecosystems and associated water resources. Past work has evaluated the effects of vegetative buffers on adsorption sites for antibiotics and the influence of these adsorption/desorption processes on transport (Chu et al., 2010; Chu et al., 2013). Proposed work will focus on these transport mechanisms for similar pollutants. They have investigated the transport and retention of plant pathogen (e.g., Phytophthora) in porous media, and the filtration of Phytophthora and Pythium from recycled irrigation water for controlling crop disease outbreaks in greenhouse (Jeon et al., 2019; Jeon et al., 2016). The results suggested that biflagellate Phytophthora zoospores were more transported than encysted zoospores through saturated sand column in Na-dominant water, and retention of encysted zoospores was greater in iron-rich porous media and at lower solution pH (Jeon et al., 2016). Also, the filtration of Pythium from recycled greenhouse irrigation water was effective in controlling disease development in floriculture crops (e.g., Poinsettias) (Jeon et al., 2019).
In an effort to connect soil and water contamination with food production, several members of our group (Feng, Hettiarachchi, Zhang) are working on the uptake of pharmaceuticals by vegetables, in-plant metabolisms of pharmaceuticals, the changes in ARGs and bacterial community in lettuce and soils due to pharmaceutical exposure, the deposition and removal of ENPs on fresh produce surfaces (Bhalsod et al., 2018; Chuang et al., 2018; Chuang et al., 2015; Li et al., 2018) as well as uptake, deposition and removal of common trace elements in urban soils (Attanayake et al., 2015; 2017). Our members have also investigated on the effect of biochar, compost and exceptional quality biosolids amendment on contaminant behaviors in soil, water and plant systems, including contaminant sorption and plant uptake of contaminants (Attanayake et al., 2015; 2017; Liu et al., 2019; Wang et al., 2019a). Exceptional quality biosolids and biochar amendment in soils has many agronomic and environmental benefits such as increasing soil organic carbon (SOC) levels, improving soil health and crop productivity, mitigating greenhouse gas emissions, and reducing contaminant bioavailability (Ahmed et al., 2014; Alvarez-Campos et al., 2018; Alvarez-Campos and Evanylo, 2019; Attanayake et al., 2015; Geesley et al., 2011; Jeffery et al., 2011; Kookana, 2010; Laird, 2008; Laird, 2008; Lehmann et al., 2006; Zhu et al., 2017). Therefore, it is important to study how these amendments in soils influence soil physicochemical and biological characteristics to better achieve intended ecosystem services.
Investigation of the presence of airborne microorganisms (bioaerosols) in the ambient air is of interest due to their environmental and human health effects (Burge, 1990; Douwes et al., 2003). Numerous studies have shown that increased exposure to bioaerosols is positively correlated with the incidence of negative respiratory health effects, including lung irritation, asthma, rhinitis, allergy, and cough (Burge, 1990; Douwes et al., 2003; Fung and Hughson, 2003; Herr et al., 2003). Microorganisms in the airborne state may experience a variety of stressors, including unfavorable temperature and humidity, lack of nutrients, UV radiation, chemical pollutants, and other variables that affect their physiological status (Hatch and Dimmick, 1966; Zentner, 1966; Handley and Webster, 1995; Tong and Lighthart, 1997; Tang, 2009). Depending on that status, airborne cells could be classified as culturable, viable but not culturable, nonviable but maintaining membrane integrity, and cell fragments (Caron et al., 1998; Oliver, 2005). When bioaerosols are collected for environmental or health investigations or other purposes, it is highly desirable that the sampling method maintains their physiological status to minimize bias when quantifying and identifying microorganisms in the sample.
Numerous sampling devices have been developed and used to collect bioaerosols using filtration, impaction, impingement, electrostatic precipitation, and other methods. However, during each sampling process, the microorganisms are inevitably exposed to additional stress, which affects their viability and culturability. It has been observed that dehydration during sampling by filters (Willeke, 1999; Wang, 2001) and portable microbial impactors (Mainelis and Tabayoyong, 2010) may cause cell injury and loss of culturability, especially in sensitive species. While one of the liquid-based bioaerosol samplers, the BioSampler (SKC Inc., Eighty Four, PA), is considered to be a relatively low-stress sampling device for collecting bioaerosols (Nevalainen et al., 1993), another study has demonstrated that certain collection fluids, including glycerol and surfactant, greatly decreased the viability of Legionella pneumophila, presumably due to the elevated osmotic pressure (Chang and Chou, 2011). Stewart et al. observed that 49% of Pseudomonas fluorescens bacteria lost their culturability after impacting an agar surface at a speed of 40 m/s, most likely due to mechanical stress (Stewart et al., 1995). Another study showed that the intactness of the genomic DNA was impaired due to the stress of impaction onto collection surface (King and McFarland, 2011). Zhao et al. (2011a; 2011b) found that sampling stress from a variety of bioaerosol samplers decreased the bacterial culturability (Zhao et al., 2011a; Zhao et al., 2011b). Thomas et al. indicated that cell membrane was the major site of damage caused by mechanical forces like impaction and shear force (Thomas et al., 2011).
Our members have published multiple contributions regarding the development and analysis of new and existing bioaerosol samplers, including the application of electrostatic methods for improved bioaerosol collection (Yao et al., 2005; Yao and Mainelis, 2006). They demonstrated the feasibility of an electrostatic collector where the use of a superhydrophobic substance (“Lotus leaf” type) and specially-shaped electrodes allows efficient bioaerosol capture into liquid droplets as small as 5 µL (Han and Mainelis, 2008; Han et al., 2010; Han et al., 2011). This device was further developed and applied in the field (Han et al., 2015). They also successfully explored the application of electrostatic phenomena for passive bioaerosol collection (Therkorn et al., 2017a; Therkorn et al., 2017b). In related work, they also developed a concept of a personal bioaerosol collector based on electrostatic principles (Han et al., 2017; Han et al., 2018). Among other advances, a research protocol for the rapid characterization of bioaerosol sampling devices using adenosine triphosphate (ATP)-based bioluminescence were also developed (Seshadri et al., 2009) and multiple studies comparing bioaerosol samplers were also conducted.
Atmospheric aerosols are known to impact our climate, both through direct interaction with sunlight and through influence on the formation, lifetime, global extent and brightness of clouds. Secondary organic aerosol (SOA), formed through the atmospheric oxidation of volatile organic compounds (VOCs), comprise a substantial fraction of this atmospheric aerosol. Globally, the burden of secondary organic constituents is much larger than that of directly emitted primary organic particulate matter. This is observed in both rural and urban areas. Yet their formation pathways are only just now beginning to be understood. Time-resolved data at the molecular level for organic matter and its volatile, intermediate volatility, and semi-volatile vapor phase counterparts is critical for understanding the sources and chemistry of atmospheric aerosols. Time-resolved simultaneous measurement of these compounds at the molecular level enabled by the new instrument under development in this on-going project by at the University of California, Berkeley (Goldstein’s Group), will provide valuable insights into the role of atmospheric aerosols in global climate and regional air pollution. Such data will aid in evaluating the role of biogenic emissions, in assessing importance of a wide variety of anthropogenic emissions in the urban environment, on understanding the global background, and in elucidating the processes transforming organic vapor emissions into particulate organic matter. When linked to fundamental studies of hygroscopicity and nucleation activation, data at the compound level will be useful in predicting the effects of organic aerosols on clouds, and ultimately on the radiation balance. Such understanding of organic aerosol sources, atmospheric processes, and effects, is necessary for the development of effective pollution reduction strategies and for elucidating the role of aerosols in radiative forcing of earth's climate, thus providing the scientific basis necessary to support models of anthropogenic impacts on climate and sound environmental policy decisions.
The goal of this multistate project is to utilize and integrate modern analytical instruments and techniques to provide information on physical properties, chemical processes, and biological processes occurring in soil systems. This includes a) development of knowledge to improve application of synchrotron tools to assess complex matrices; b) development of sample collection and sample preparation methods; c) continued development of faster analytical methods that will allow us to do more comparative investigations of complex environments and automated in-situ instrumentation for field-based organic chemical composition measurements of particulate matter and semi-volatile gases; d) continued development of analytical and data analysis methods for C, N, P, Al, Ca, Fe, trace elements, and organic chemicals; e) education of participants and the wider community of agricultural researchers in the use and availability of these techniques and instruments; and f) interaction with national laboratory facilities at Argonne, Brookhaven, Stanford, Berkeley and Hanford to promote their use in the agricultural sciences and assist in the development and acquisition of equipment and expertise relevant to the agricultural science community. To achieve these goals, three objectives will be pursued.
Objectives
-
Help multi-state members and scientific community members access and use advanced molecular and microscopic tools.
-
Educate about and help prepare sample preparation methods used in pure systems for advanced molecular and microscopic analysis of soil-plant-water and air systems so that fundamental information on these complex systems can be discovered.
-
Characterize the physical, chemical, biological and morphological properties of particulate matter and their agricultural, environmental, human health and economic impacts over a wide range of spatial and temporal scales, including their potential effects on ecological sustainability, food and energy production, climate change, air and water quality, soil health, and human health.
Methods
Objective 1: Help multistate members and scientific community members access and use advanced molecular and microscopic tools.
While there is growth in the number of micro- and nano-probe instruments at synchrotron facilities worldwide, the scientific user base for these tools in earth and environmental science is still underdeveloped. Demand for access to these techniques by environmental biogeochemists continues to increase. It is becoming increasingly difficult to obtain beamtime to conduct research using the newer and/or less common techniques and beamlines, such as STXM-NEXAFS (Hettiarachchi et al., 2017). Due to the scarcity of instrument time and challenges specific to environmental samples, fully developed research programs where statistically responsible sampling and/or data collection can be employed are limited. An additional and more problematic issue for new synchrotron users is gaining the knowledge required to conduct high-quality measurements and analyze, interpret, and publish meaningful data. We will continue to facilitate training of new instrument users to synchrotron-radiation expertise, and play a key role in helping these techniques fulfill their promise for high-quality, high-impact environmental research over the next decade. The role of this project on these activities is facilitated by current active involvement by members of this multistate project on research at synchrotron facilities at the Canadian Light Source, Brookhaven, Argonne and Lawrence Berkeley National Laboratories and the Stanford Synchrotron Radiation Light Source. Donald Sparks serve on the users' executive committee at the National Synchrotron Light Source at Brookhaven National Laboratory, which place the members of this project in an ideal position to promote and assist users. Yuji Arai is serving on the proposal review panel at the Advanced Photon Source while Ganga Hettiarachchi and Daniel Strawn serving as proposal reviewers for Stanford Synchrotron Radiation Light Source and Canadian Light Sources.
Objective 2: Educate about and help prepare sample preparation methods used in pure systems for advanced molecular and microscopic analysis of soil-plant-water and air systems so that fundamental information on these complex systems can be discovered.
The past decade has witnessed significant advances in technologies related to spatially resolved X-ray spectroscopic techniques, both as a result of advances in X-ray optics, focusing devices, and detectors, and because of greater availability of high brilliance synchrotron facilities worldwide. This includes the Advanced Photon Source (APS) at the Argonne National Laboratory, the Advanced Light Source (ALS) in Berkeley CA, the updated Stanford Synchrotron Radiation Lightsource (SSRL), and the National Synchrotron Light Source II (NSLS-II) at the Brookhaven National Laboratory. The result is that spatially resolved synchrotron-based X-ray fluorescence (XRF), X-ray absorption near edge structure spectroscopy (XANES) and extended X-ray absorption fine structure (EXAFS) spectroscopy have become mainstream techniques in a number of scientific disciplines and are providing molecular level information not previously available using other techniques. While there exists a variety of micro-analytical techniques that have long been used in these disciplines, synchrotron-based spatially resolved X-ray fluorescence spectroscopy (XRF), XANES, EXAFS spectroscopy, X-ray diffraction (XRD) as well as scanning transmission X-ray microscopy (STXM) are important methods that complement characterization of particulates by traditional techniques, as well as by other new methods, such as spatially resolved luminescence, FTIR, and Raman spectroscopy, CT scanning aided with 2D soil zymography. The reasons for this are the elemental specificity, low detection limits, non-destructive nature of the measurement, the ability, in many instances, to examine samples in situ, and the ability to extract information on valence states and on specific bonding environments or molecular forms of target elements in complex matrices.
One of the greatest challenges for using spatially resolved microspectroscopy is sample preparation. A focus of this multistate group will be to work collaboratively on developing a knowledge base on the sample preparation methods to avoid artifacts. For instance, Donald Sparks, Daniel Strawn and other team members are planning a collaboration to determine ideal sample preparation of natural material such as soils for analysis at synchrotron facilities.
It is now widely accepted that specific information on the composition, surface characteristics, and morphology of particulate matter is prerequisite to the development of a comprehensive understanding of toxic element and nutrient behavior in soils and sediments. For example, phosphorous interacts with oxide surfaces to form strong chemical complexes that may reduce its availability to plants or transport to ground and surface waters. On the other hand, colloidal transport by wind or water may hasten the mobility of phosphorous while removing it from a cropping system. The nature of not only the P-soil interaction, but also the nature of the particle itself and its susceptibility to movement must be determined. This cannot be done by any one instrument or investigator. We will apply a mixture of spectroscopic, computational, and analytical methods to particles in complex systems such as soils and sediments under environmentally relevant conditions. Members of this project (e.g., Arai, Strawn, Hettiarachchi, Sparks) are planning the use of novel synchrotron-based X-ray absorption and X-ray fluorescence spectroscopy to speciate phosphorus in soils.
Some of our members will contribute towards Objective 2 by integrating modern analytical instruments and other techniques (Kravchenko, Anderson, Mainelis). One key instrumental tool that they use is X-ray computed micro-tomography scanning (CT). Our members work in close collaboration with colleagues from the APS at Argonne National Laboratory in Argonne (beamline 13-BM-D of the GeoSoilEnvironCARS) and with other researchers at their universities for conducting X-ray CT scanning. The overarching goals of their work are to characterize micro-scale spatial patterns in soil physical characteristics. Specifically, they use the scanning tools to describe soil pore structure, i.e., pore size distribution, porosity, pore connectivity and tortuosity. The key research questions they aim at answering is how long-term implementation of different land use and management practices influences soil pore structure; how properties of the pore structure affect processes governing soil carbon storage and protection, and production and emission of greenhouse gases, CO2 and N2O from the soil; and how soil pore structure influences soil microorganisms and their functioning.
Answering these questions depends on new experimental approaches associated with CT scanning and integrating CT scanning with other analytical techniques. Among such approaches that they implement is dual energy scanning with iodine and barium dopants. Dual energy scanning of soil samples with added iodine and/or barium solutions enables identifying the spatial micro-scale patterns in distribution of liquids added to soil and their movement and storage in pores of different sizes - the aspect crucial for assessing the role of pores of different sizes as microbial habitats. Another novel tool they are exploring is dual energy scanning of soil samples saturated with osmium tetroxide vapors. The gas binds with organic materials in soil, thus making it possible to identify the spatial patterns in distribution of soil organic matter. The samples are scanned at two energies, 74 and 73.8 keV, which provide respective images above and below osmium K-edge. By taking the difference between the above and below K-edge images, a map of the stained soil organic materials can be generated. This method offers a great promise in deciphering the mechanisms behind soil carbon protection. Yet, another technique our members are integrating with CT scanning is 2D soil zymography. Zymography enables obtaining micro-scale maps of spatial distribution patterns for several extracellular enzymes on the intact soil surfaces. During zymography a membrane saturated with an enzyme-specific fluorogenic substrate is placed on the surface of a soil sample. Upon a contact of the substrate with soil enzymes, a fluorescent product (e.g. MUF: 4-methylumbelliferone, or AMC: 7-amido-4-methylcoumarin) is released and its presence on the membrane is then detected under UV light. The fluorescing pattern on the membrane reflects spatial distribution of active enzymes on the soil surface. Zymographic measurements on the soil samples subjected to previous CT scanning enable finding associations between presence of soil pores, particulate organic matter and roots with relative enzyme activities, which then can be used to gain better understanding of the microbial activities and distributions in soil.
Some members of our group will continue further development of electrostatics-based samplers for bioaerosol collection, more specifically further development and conversion of these samplers to portable, battery-operated devices (Mainelis). The operation by battery and portability will enable their wide transfer and application in the field, where electricity is not available or access is not convenient. During further development of the samplers, our members will aim to make them smaller, lighter and battery-operated while maintaining their key performance characteristics, such as high collection efficiency and preservation of microorganism properties needed for their analysis. Since the electrostatic collection method is a low-power and low pressure drop process, devices based on this principle are especially amenable to be battery operated. The collection efficiency of the samplers will be determined by comparing their-determined microorganism concentrations against those determined by the reference instruments. Here the microorganism concentrations will be determined culture-based technique, microscopy, ATP-based luminescence, qPCR, and flow cytometry. The latter technique is especially useful because it allows us to determine the fraction of microorganisms that are viable, injured and dead, thus providing information about the biological performance of the devices. The experimental setup and procedural details of various sampler testing have been published in the literature, including by our group (Han et al., 2010; Han et al., 2011). In the initial phase of the work, the experiments will be performed in laboratory with specifically aerosolized microorganisms. Once the laboratory trials are successful, the testing will move to the field. Here, we will take advance of unique resources offered by the multistate grant mechanisms, namely access to the participants’ sites. Specifically, the battery-operated electrostatic samplers, will be tested in various agricultural environments, access to which will be secured through collaborative efforts with multistate group members.
Objective 3: Characterize the physical, chemical, biological and morphological properties of particulate matter and their agricultural, environmental, human health and economic impacts over a wide range of spatial and temporal scales, including their potential effects on ecological sustainability, food and energy production, climate change, air and water quality, soil health, and human health.
Several members of our group will work on measuring and characterizing particulate P in fertilized soils, tile waters, surface runoff, etc. at farms with various cropping systems and agricultural management (Arai, Hettiarachchi, Sparks, Strawn). This will be done immediately following fertilization, during growing season or during high flow events (e.g., typically March through May in the Midwest), where they can obtain liters of water for this in-depth chemical analysis using novel techniques (e.g., P-31 NMR, P K-edge XANES), in addition to performing other wet chemical analyses (e.g., total P, dissolved reactive P, P fractionation) as well as traditional particle characterization (e.g., elemental association, morphology) using Transmission Electron Microscopy (TEM) and or SEM techniques at their home institutions.
Many of our group members will also study impacts of extreme events effects on biogeochemical processes in soils, including contaminant (metals, metalloids, and nutrients) mobility and cycling both agricultural and non-agricultural areas. Soil and sediment samples will be collected from contaminated sites (e.g., urban sites, coastal sites as well as along a salinity gradient that has been impacted by high inputs of fertilizers). The samples will be characterized for physicochemical and mineralogical properties and salinity using standard methods. Additional characterization, including sequential extractions will be conducted on the samples to gain insights on metal(loid) and nutrient associations with different soil and sediment components and to complement synchrotron-based microfocused X-ray fluorescence (XRF) and X-ray absorption (XAS) spectroscopic analysis. The samples will be exposed to various conditions and stresses in batch and microcosm reactor experiments for various periods of time to simulate different scenarios to perform further studies (Kalnejais et al. 2010; LeMonte et al., 2017).
Some of our members will perform an array of experiments to understand interactions of ENPs, antibiotic resistance genes (ARGs), antibiotic resistant bacteria (ARB), and bacteria with plants, and develop potential mitigation strategies, including growth experiments in greenhouses using model vegetable crops (e.g., lettuce, spinach, radish, and carrots) or in field (Xia, Zhang). They will use high throughput qPCR and 16S rRNA amplicon sequencing to study ARG abundance and diversity and bacterial community, respectively, in addition to using various imaging techniques.
Another group of our members will use µ-CT alone or together with other techniques to get quantitative information of soil microstructure is required to improve our understanding of various soil processes (Anderson, Kravchenko). Soil cores for these studies will be collected from selected soil management treatments including cover crop management systems and agroforestry buffer systems. Soil cores will be collected in 5 cm diam by 10 cm long plexiglass rings and samples will be stored at 4oC before scanning. Image Acquisition, Tomographic Reconstruction, and Image Analysis will be performed as described by Udawatta et al. (2013) and the 3-Dimentional Medial Axis (3-DMA) computer software will be used to examine differences in geometrical pore characteristics among the treatments using a 1.7 GHz Linux computer (Lindquist et al. 2005; http://www.ams.sunysb.edu/~lindquis/3dma/3dma_rock/3dma_rock.html).
Few members in our group will also focus on recovering and recycling of energy, nutrients, including carbon and waters from waste and wastewaters (Hettiarachchi, Strawn, Xia). They will be working on testing the hypothesis that innovative wastewater treatment technologies can produce the right water from different sources, while recovering nutrients and producing soil amendments for crop production and protecting the environment. They will conduct greenhouse and field research on how soil factors affect nutrient and potential contaminant uptake by selected crops grown in the Inland Pacific Northwest and Midwest regions. They will research on how waste, recovered products/coproducts and treated waters affects nutrients and contaminant mobility and bioavailability, and planning to use various analytical tools to (e.g., XAS, XRF, XRD, FTIR and UV/Vis spectroscopy) to elucidate speciation nutrients and contaminants in soils and plants.
Measurement of Progress and Results
Outputs
- Presentations, organizing special symposiums and topical sessions by group members at national/international meetings: These include those of the American Chemical Society, American Geophysical Union, European Geophysical Union, Goldschmidt Conference, American Society of Agronomy/Crop Science Society of America/Soil Science Society of America, Clay Minerals Society, American Association of Aerosol Research and other relevant meetings.
- Publications in peer-reviewed and high-impact journals in the field of particulate science such as Environmental Science and Technology, Langmuir, Soil Science Society of America Journal (SSSAJ), Journal of Environmental Quality (JEQ), Geochimica et Cosmochimica Acta, Journal of Geophysical Research, Atmospheric Chemistry and Physics, Proceedings of the National Academy of Sciences, Science and other relevant journals.
- Proposals submitted to private, federal, and multinational funding sources: AFRI, DOE, DOD, EPA, NIEHS, and NSF. Project members could submit joint proposals including collaborative projects and multi-investigator, interdisciplinary projects involving non-project colleagues at multiple universities and institutions. There is also the opportunity to collaborate with scientists at national laboratories, e.g., Argonne and Brookhaven, since project members have ongoing research with these investigators on synchrotron-based studies.
- Annual meetings of NC2187 group that coordinate with tours of national user facilities and/or concurrently at national meetings.
Outcomes or Projected Impacts
- Generate new fundamental knowledge of the properties of micro-and nano-particulates in air, soil, and water. We will examine particles and their spatial organization in agricultural and nonagricultural systems that directly impact the availability of nutrients and water to human and other living organisms. Microscopic and spectroscopic methods will be used to characterize the locations, bonding mechanisms, and concentrations of C, N, P, K, Fe, micronutrients, meta(loid)s and contaminant species associated with organic and inorganic particles and their aggregates.
- Microscopic and molecular characterization of particles will be combined with macroscopic studies to link particle properties and those resulting from their spatial organization with environmental behavior. We will develop mechanistic models for the partitioning of material at interfaces to link micro- and nano-meter scale processes to mass transfer at larger scales. These models will be applied to environmental and agricultural systems, including interactions between minerals and plant roots, atmosphere and air-borne particles, sediment and water, and nanoparticles and microbial cells.
- Increase the utilization of national laboratory supported advanced analytical techniques by agricultural scientists. Members will use their contacts and influence on user committees of national laboratories and other service centers to gain access to state-of-the-art instrumentation. In addition, they will inform other members of the availability and capabilities of instrumentation through seminars, email, Zoom meetings, and workshops at the Soil Science Society of America Meetings. Each member will work to educate and encourage colleagues and collaborators at their institutions in the use and means of accessing modern instrumentation, including synchrotron sources and national user facilities.
Milestones
(2020):1. Data generation. 2. Submit Final Report of NC 1187. 2. Organize and participate in SSSA International Annual Meetings session entitled “Particulate Matter in a Changing Environment: Implications for Agriculture and Human Health” to showcase NC1187 multistate group accomplishments. Recruit new members.(2021):1. Data generation 2. Increase the utilization of national laboratories and collaborations with researchers at the National laboratories 3. Publish a Special Section in a high impact journal such as SSSAJ or JEQ with selected articles from the session held the previous year. 2. Develop and Evaluate New and Existing Relations with User Facilities. Using the special relationship between NC1187 and national synchrotron facilities such as APS as a model, form similar bonds with national labs, including EMSL, the Advanced Light Source (Berkeley), the National Synchrotron Light Source (NSLS), Stanford Synchrotron Radiation Laboratory, and user facilities at universities.
(2022):1. Data generation 2. Increase the utilization of national laboratories and collaborations with researchers at the National laboratories 3. Submission of joint proposals including collaborative projects and multi-investigator, interdisciplinary projects involving non-project colleagues at multiple universities and institutions.
(2023):1. Data generation. 2. Compilation of information on the properties of micro-and nano-particulate in air, soil, and water 3. Compilation of information on impact of particles and their spatial organization in agricultural and non-agricultural systems on the availability of nutrients and water 4. Compilations of information on the progress and utilization of mechanistic models for the partitioning of material at interfaces to link micro- and nano-meter scale processes to mass transfer at larger scales.
Projected Participation
View Appendix E: ParticipationOutreach Plan
- Develop complementary research methods to solve difficult problems in particulate matter characterization and behavior requiring multi-instrumental expertise and availability. To stimulate this outreach, we will first organize a special symposium at the 2020 ASA/CSSA/SSSA Annual Meeting. This will serve to showcase member skills, interests, and needs. Based on this symposium, we will then publish a special section in JEQ.
- Continuation and expansion of relations with national user facilities including membership on user committees, submission of white papers on user facility needs for agriculture, and interaction with specific facility scientists to develop instruments and methods germane to agricultural research.
- Hold workshops, conferences, and short courses that educate agricultural scientists as to the availability, application, and use of modern instruments for particulate matter research. Members will present their research at professional society meetings to educate other scientists of the value and capabilities of advanced molecular scale facilities. Because most group members have State Agricultural Experiment Station (AES) appointments, applied aspects of research that utilizes advanced analytical facilities will be reported to constituents (e.g., Ag day, field day), and AES members and administrators.
- Together with non-member colleagues, our members will develop or contribute to various educational materials including extension publications beneficial to educators, regulators, local government decision makers, the concerned public, other scientists, and stakeholders on cycling and reactivity of nutrients and contaminants in air, water, and soil. We will continue to convey the science on the implications of particulate matter reactivity and cycling on agriculture and human health through written publications, field demonstrations, workshops, webinars, and direct interaction with stakeholders and clientele.
Organization/Governance
Governance of the multistate project will lie with three elected positions: chair, vice-chair and secretary. Each position is assigned specific tasks and is held for two years with officers rotating through the positions as follows: the chair steps down, the vice-chair becomes chair, the secretary becomes vice-chair and a newly elected individual becomes secretary. Appointed in 2018: Ganga Hettiarachchi (chair), Wei Zhang (vice-chair), Secretary to be elected. The chair is responsible for organizing the technical program for the upcoming "all hands" meeting. Each official group on the multistate project is required to present a research report at each "all hands" meeting. If synchrotron funding is secured, each group allocated synchrotron time during a given year is required to attend the "all hands" meeting and present a research report. The research report will consist of an abstract and either an oral presentation or a poster presentation. If synchrotron funding is secured, the chair will be the official liaison with funding source(s) and synchrotron facilities. The vice-chair is responsible for organizing the logistics for the "all hands" meeting: selecting a venue, reserving rooms suitable for research presentations and the business meeting, arranging housing, etc. The secretary is responsible for all communication with official members of the multistate project and, if synchrotron funding is secured, individuals allocated synchrotron time. The secretary is also responsible for preparing the annual report for the multistate project, which will include the proceedings of the "all hands" meeting: minutes of the business meeting, the technical program, and the abstracts of all research reports. All members are responsible for creating links to user facilities and conveying information on availability and capabilities to the membership. Participants are encouraged to acknowledge the NC1187 project with support from NIFA via the Hatch Act with the match provided by the designated states.
Literature Cited
Ahmad, M., Rajapaksha, A.U., Lim, J.E., Zhang, M., Bolan, N., Mohan, D., Vithanage, M., Lee, S.S., and Ok, Y.S. (2014). Biochar as a sorbent for contaminant management in soil and water: A review. Chemosphere 99, 19-33.
Alvarez-Campos, O., Badzmierowski, M. , Evanylo, G.K. , Bamber, K. , and Yu, H-C. (2018). Development and testing of exceptional quality biosolids-based by-products for urban landscapes. Compost Science & Utilization 26(4): 234-245.
Alvarez-Campos, O., and Evanylo, G.K. (2019). Plant available nitrogen estimation tools for a biosolids-amended, clayey urban soil. Soil Sci. Soc. Am. J. 83: 808-816.
Anderson, S.H. and J.W. Hopmans. (2013). Soil-Water-Root Processes: Advances in TomographyImaging. 304 pp. Soil Science Society of America. Special Publication 61. Madison, WI.
Assouline, S. (2004). Rainfall-induced soil surface sealing: a critical review of observations, conceptual models and solutions, Vadose Zone J. 3: 570-591.
Attanayake, C.P., Hettiarachchi, G.M., Ma, Q. , Pierzynski, G.M. , and Ransom, M.D. (2017). Lead speciation and in vitro bioaccessibility of compost-amended urban garden soils. J. Environ. Qual. 46:1215-1224.
Attanayake, C.P., Hettiarachchi, G.M., Martin, S., and Pierzynski, G.M. (2015). Potential bioavailability of lead, arsenic, and polycyclic aromatic hydrocarbons in compost-amended urban soils. J. Environ. Qual. 44:930-944.
Beesley, L., Moreno-Jiménez, E., Gomez-Eyles, J.L., Harris, E., Robinson, B., and Sizmur, T. (2011). A review of biochars’ potential role in the remediation, revegetation and restoration of contaminated soils. Environmental Pollution 159(12), 3269-3282.
Bhalsod, G.D., Chuang, Y.-H., Jeon, S., Gui, W., Li, H., Ryser, E.T., Guber, A.K., Zhang, W. (2018). Uptake and accumulation of pharmaceuticals in overhead- and surface-irrigated greenhouse lettuce. Journal of Agricultural and Food Chemistry 66(4), 822-830.
Bouchard, D., Zhang, W., and Chang, X. (2013). A rapid screening technique for estimating nanoparticle transport in porous media. Water Research 47(12), 4086-4094.
Bouchard, D., Zhang, W., Powell, T., Rattanaudompol, U. (2012). Aggregation kinetics and transport of single-walled carbon nanotubes at low surfactant concentrations. Environmental Science & Technology 46(8), 4458-4465.
Brussaard, L., and van Faassen, H.G. (1994). Effects of compaction on soil biota and soil biological processes. pp 215-235. In B.D. Soane and V. vanOuwerkerk (ed.) Soil compaction in crop production. Elsevier Science, Amsterdam.
Burge, H. (1990). Bioaerosols: Prevalence and health effects in the indoor environment. J. Allergy. Clin. Immunol., 86(5): 687-701.
Burkholder, J.M., and H.B. Glasgow. (1997). Pfiesteria piscicida and other Pfiesteria-like environmental dinaflagellates: behavior, impacts, and environmental controls. Limnol. Oceanogr. 42, 1052–1075.
Burton, E.D., Bush, R.T., Sullivan, L.A., Johnston, S.G., and R.K., Hocking. (2008).Chemical Geology 253, 64–73. DOI: 10.1016/j.chemgeo.2008.04.006
Cantwell, G., Burgess, R.M., and Kester, D.R. (2002). Environ. Sci. Technol. 36, 5328-5334. DOI: 10.1021/es0115058
Caron, G.N., Stephens, P., and Badley, R.A. (1998). Assessment of bacterial viability status by flow cytometry and single cell sorting. J. Appl. Microbiol., 84: 988-998.
Carpenter, S.R., N. F. Caraco, D. L. Correll, R. W. Howarth, A. N. Sharpley, and V. H. Smith. (1998). Nonpoint pollution of surface waters with phosphorus and nitrogen. Ecol. Appl. 8, 559–568.
Chang, C.-W., and Chou, F.-C. (2011). Assessment of bioaerosol sampling techniques for viable Legionella pneumophila by ethidium monoazide quantitative PCR. Aerosol Sci. Technol., 45(3): 343 - 351.
Chu, B., Goyne, K., Anderson, S., Lin, C-H, and Lerch, R. (2013). Sulfamethazine Sorption to Soil: Vegetative Management, pH, and Dissolved Organic Matter Effects. Journal of environmental quality. 42. 794-805.
Chu, B., Goyne, K., Anderson, S., Lin, C-H, and Udawatta, R. (2010). Veterinary antibiotic sorption to agroforestry buffer, grass buffer and cropland soils. Agroforestry Systems. 79. 67-80.
Chuang, Y.-H., Liu, C.-H., Hammerschmidt, R., Zhang, W., Boyd, S.A., and Li, H. (2018). Metabolic demethylation and oxidation of caffeine during uptake by lettuce. Journal of Agricultural and Food Chemistry 66(30), 7907–7915.
Chuang, Y.-H., Zhang, Y., Zhang, W., Boyd, S.A., and Li, H. (2015). Comparison of accelerated solvent extraction and quick, easy, cheap, effective, rugged and safe method for extraction and determination of pharmaceuticals in vegetables. Journal of Chromatography A 1404(0), 1-9.
Coward, E.K., Ohno, T. and Sparks, D.L. (2019). Direct Evidence for Temporal Molecular Fractionation of Dissolved Organic Matter at the Iron Oxyhydroxide Interface. Environmental Science & Technology. 53: 642-650.
Diaz, R. J., and Rosenberg, R. (2008). Spreading dead zones and consequences for marine ecosystems. Science (New York, N.Y.) 321, 926–929.
Dodds, W. K., (2006). Nutrients and the “dead zone”: the link between nutrient ratios and dissolved oxygen in the northern Gulf of Mexico. Front. Ecol. Environ. 4, 211–217. Douwes, J., Thorne, P., Pearce, N., and Heederik, D. (2003). Bioaerosol health effects and exposure assessment: Progress and prospects. Ann. Occup. Hyg., 47(3): 187-200.
Douwes, J., Thorne, P., Pearce, N., and Heederik, D. (2003). Bioaerosol health effects and exposure assessment: Progress and prospects. Ann. Occup. Hyg., 47(3): 187-200.
Fenter, P.A., M.L. Rivers, N.C. Sturchio, and S.R. Sutton. (eds.) 2002. Applications of Synchrotron Radiation in Low-Temperature Geochemistry and Environmental Science. Mineralogical Society of America.
Foley, J. A., R. DeFries, G. P. Asner, C. Barford, G. Bonan, S. R. Carpenter, F. S. Chapin, M. T. Coe, G. C. Daily, H. K. Gibbs, J. H. Helkowski, T. Holloway, E. A. Howard, C. J. Kucharik, C. Monfreda, J. A. Patz, I. C. Prentice, N. Ramankutty, and P. K. Snyder. (2005). Global consequences of land use. Science. 309(5734):570–574.
Fung, F., and Hughson, W. (2003). Health effects of indoor fungal bioaerosol exposure. Appl. Occup. Environ. Hyg., 18(7): 535-545.
Galkaduwa, M.B., Hettiarachchi, G.M., Kluitenberg, G.J. and Hutchinson, S.L. (2018). Iron oxides minimize arsenic mobility in soil material saturated with saline wastewater. J. Environ. Qual. 47(4):873-883.
Górny, R. L. (2020). Microbial aerosols: Sources, properties, health effects, exposure assessment—A review. KONA Powder Particle J. 2020005
Gronberg, J.M., and Arnold, T.L. (2017). County-level estimates of nitrogen and phosphorus from animal manure (2007 and 2012) and 30-meter-resolution grid of counties (2010) for the conterminous United States: U.S. Geological Survey data release, https://doi.org/10.5066/F7X34VMZ.
Han, T., An, H.R., and Mainelis, G. (2010). Performance of an Electrostatic Precipitator with Superhydrophobic Surface when Collecting Airborne Bacteria. Aerosol Science and Technology, 44(5): 339-348.
Han, T., and Mainelis, G. (2008). Design and Development of an Electrostatic Sampler for Bioaerosols with High Concentration Rate. J. Aerosol Sci., 39: 1066–1078.
Han, T., Nazarenko, Y., Lioy, P.J., and Mainelis, G. (2011). Collection efficiencies of an electrostatic sampler with superhydrophobic surface for fungal bioaerosols. Indoor Air, 21: 110-120.
Han, T., Thomas, N., and Mainelis, G. (2017). Design and development of a self-contained personal electrostatic bioaerosol sampler (PEBS) with a wire-to-wire charger Aerosol Science and Technology, 51: 903-915
Han, T., Thomas, N., and Mainelis, G. (2018). Performance of personal electrostatic bioaerosol sampler (PEBS) when collecting airborne microorganisms, Journal of Aerosol Science, 124: 54-67,
Han, T., Wren, M., DuBois, K., Therkorn, J., and Mainelis, G. (2015). Application of ATP-based bioluminescence for bioaerosol quantification: effect of sampling method. Journal of aerosol science, 90, 114–123.
Handley, B.A., and Webster, A.J.F. (1995). Some factors affecting the airborne survival of bacteria outdoors. J. Appl. Bacteriol., 79: 368-378.
Hatch, M.T., and Dimmick, R.L. (1966). Physiological responses of airborne bacteria to shifts in relative humidity. Bacteriol. Rev., 30: 597-602.
Herr, C.E., Zur Nieden, A., Jankofsky, M., Stilianakis, N.I., Boedeker, R.H., and Eikmann, T.F. (2003). Effects of Bioaerosol Polluted Outdoor Air on Airways of Residents: a Cross Sectional Study. Occup. Environ. Med., 60(5): 336-42.
Hettiarachchi, G.M., Donner, E., and Doelsch, E. (2017). Introduction to Special Section: Application of Synchrotron Radiation-Based Methods for Environmental Biogeochemistry. J. Environ. Qual. 46:1–7
Ioannidis, M.A., and Chatzis, I. (2000). On the geometry and topology of 3D stochastic porous media. J. Colloid and Interface Science 229:323-334.
Jeffery, S., Verheijen, F.G.A., van der Velde, M., and Bastos, A.C. (2011). A quantitative review of the effects of biochar application to soils on crop productivity using meta-analysis. Agriculture, Ecosystems & Environment 144(1), 175-187.
Jeon, S., Krasnow, C.S., Bhalsod, G.D., Harlan, B.R., Hausbeck, M.K., Safferman, S.I., and Zhang, W. (2019). Rapid Sand Filtration of Recycled Irrigation Water Controlled Pythium Root Rot of Poinsettia in Greenhouse. HortTechnology 1(aop), 1-12.
Jeon, S., Krasnow, C.S., Kirby, C.K., Granke, L.L., Hausbeck, M.K., and Zhang, W. (2016). Transport and retention of Phytophthora capsici zoospores in saturated porous media. Environmental Science & Technology 50(17), 9270-9278.
Kah, M., Beulke, S., Tiede, K., and Hofmann, T. (2013). Nanopesticides: State of knowledge, environmental fate, and exposure modelling. Critical Reviews in Environmental Science and Technology 43(16), 1823-1867.
Kalnejais, L.H., Martin, W.R., Signell, R.P., and M.H. Bothner. 2007. Environ. Sci. Technol. 41, 2282-2288.
Kelley, S.D., D. Hesterberg, and B. Ravel. 2008. Analysis of soils and minerals using X-ray absorption spectroscopy, In A. L. Ulrey and L. R. Drees, (eds.) Methods of Soil Analysis: Part 5-Mineralogical Methods. Soil Science Society of America, Madison.
Kim, H.M., Anderson, S.H., Motavalli, P.P., and Gantzer, C.J. (2010). Compaction effects on soil macropore geometry and related parameters for an arable field. Geoderma 160:244-251.
King, M.D., and McFarland, A.R. (2011). Bioaerosol sampling with a wetted wall cyclone: Cell culturability and DNA integrity of Escherichia coli bacteria. Aerosol Sci. Technol., 46: 82-93.
Kookana, R.S. (2010). The role of biochar in modifying the environmental fate, bioavailability, and efficacy of pesticides in soils: a review. Soil Research 48(7), 627-637.
Kravchenko, A.N, E. R. Toosi, A.K. Guber, N. E. Ostrom, J. Yu, K. Azeem, M. L. Rivers, and G. P. Robertson. (2017). Hotspots of soil N2O emission enhanced through water absorption by plant residue. Nature Geoscience doi:10.1038/ngeo2963.
Kravchenko, A. N., and Guber, A. K. (2017). Soil pores and their contributions to soil carbon processes. Geoderma 287, 31–39.
Kravchenko, A.N., J. Fry, and A.K. Guber. 2018a. Water absorption capacity of soil-incorporated plant leaves can affect N2O emissions and soil inorganic N levels. Soil Biology and Biochem. 121:113-119.
Kravchenko, A.N, Guber, A.K., Quigley, M.Y., Koestel, J., Gandhi, H., and Ostrom, N. E. (2018b). X-ray computed tomography to predict soil N2O production via bacterial denitrification and N2O emission from soils in contrasting bioenergy cropping systems. Global Change Biology Bioenergy 00: 1-16 doi.org/10.1111/gcbb.12552.
Kutlu, T., Guber, A. K. , Rivers, M. L., and Kravchenko, A. N. (2018). Moisture absorption by plant residue in soil. Geoderma 316:47-55.
Laird, D.A., 2008. The charcoal vision: A win–win–win scenario for simultaneously producing bioenergy, permanently sequestering carbon, while improving soil and water quality. Agronomy Journal 100(1), 178-181.
LeMonte, J.J., Stuckey, J.W., Sanchez, J.Z., Tappero, R., Rinklebe, J., and D.L. Sparks. (2017). Environ. Sci. Technol. 51, 5913−5922. DOI: 10.1021/acs.est.6b06152
Lehmann, J., Gaunt, J., and Rondon, M. (2006). Bio-char sequestration in terrestrial ecosystems – A review. Mitigation and Adaptation Strategies for Global Change 11(2), 395-419.
Li, Y., Chuang, Y.-H., Sallach, J.B., Zhang, W., Boyd, S.A., and Li, H. (2018.) Potential metabolism of pharmaceuticals in radish: Comparison of in vivo and in vitro exposure. Environmental Pollution 242, 962-969.
Lindquist, W.B. 2002. Quantitative analysis of three dimensional X-ray tomographic images. In U. Bonse (ed.) Development in X-ray tomography. Proceedings of SPIE 4503, SPIE Bellingham, WA.
Lindquist, W.B., Lee, S.M., Oh, W., Venkatarangan, A.B., Shin, H. and Prodanovic, M. (2005). 3DMA-Rock A Software Package for Automated Analysis of Rock Pore Structure in 3-D Computed Microtomography Images. [Online]. Available at http://www.ams.sunysb.edu/~lindquis/3dma/3dma_rock/3dma_rock.html. Department of Applied Mathematics and Statistics, SUNY at Stony Brook, Stony Brook, N.Y.
Liu, C.H., Chuang, Y.-H., Li, H., Boyd, S.A., Teppen, B.J., Gonzalez, J.M., Johnston, C.T., Lehmann, J., and Zhang, W. (2019). Long-term sorption of lincomycin to biochars: The intertwined roles of pore diffusion and dissolved organic carbon. Water Research 161, 108-118.
Liu, Y., G. Villalba, R. U. Ayres, and H. Schroder. 2008. Global phosphorus flows and environmental impacts from a consumption perspective. Journal of Industrial Ecology 12, 229–247.
Logan, T. J., G. W. Randall, and D. R. Timmons. 1980. Nutrient content of tile drainage from cropland in the north central region. North Central Regional Research Publication 268. Ohio Agricultural Research and Development Center, Wooster, OH.
Lombi, E., and J. Susini. 2009. Synchrotron-based techniques for plant and soil science: opportunities, challenges and future perspectives. Plant and Soil 320:1-35.
Mainelis, G., and Tabayoyong, M. (2010). The effect of sampling time and the overall performance of portable microbial impactors. Aerosol Sci. Technol., 44: 75-82.
Morales, V.L., Parlange, J.-Y., Wu, M., Pérez-Reche, F.J., Zhang, W., Sang, W., and Steenhuis, T.S. (2013). Surfactant-mediated control of colloid pattern assembly and attachment strength in evaporating droplets. Langmuir 29(6), 1831-1840.
National Centers for Environmental Information (NCEI) (2019). https://www.ncei.noaa.gov/news/national-climate-201905
Nevalainen, A., Willeke, K., Liebhaber, F., Pastuszka, J., Burge, H., and Henningson, E. (1993). Bioaerosol sampling: Aerosol measurement principles, techniques, and applications. New York, Van Nostrand Reinhold.
NRC, (2012). A research strategy for environmental, health, and safety aspects of engineered nanomaterials, Committee to Develop a Research Strategy for Environmental, Health, Safety Aspects of Engineered Nanomaterials, National Research Council (NRC), The National Academies Press.
Oliver, J.D. (2005). The viable but nonculturable state in bacteria. J. Microbiol., 43: 93-100.
Parker, M., McDonald, M.R., and Boland, G. (2014). Evaluation of air sampling and detection mentod to quantify airborne ascospores of Scledrotinia sclerotiorum. Plant Diesease. 98: 32-42.
Pitumpe Arachchige, P.S., Hettiarachchi, G.M., Rice, C.W., Dynes, J.J., Maurmann, L. , Wang, J., Karunakaran, C., Kilcoyne, A.L.D. A.L.D., Attanayake, C.P. C.P., Amado, T.J. C., and Fiorin, J.E. (2018). Sub-micron level investigation reveals the inaccessibility of stabilized carbon in soil microaggregates. Scientific Reports. 8: 16810.
Reponen, T., Willeke, K., Grinshpun, S., and Nevalainen, A. (2011). Biological Particle Sampling, in Aerosol Measurement: Principles, Techniques and Applications, P. Kulkarni, P. Baron, and K. Willeke, eds., John Wiley & Sons, Inc., Hoboken, NJ, pp. 549–570.
Roose T., Keyes, S.D. , Daly, K.R. , Carminati, A. , Otten, W., Vetterlein, D., and Peth, S. S. (2016). Challenges in imaging and predictive modeling of rhizosphere processes. Plant Soil 407:9-38.
Sang, W., Morales, V.L., Zhang, W., Stoof, C.R., Gao, B., Schatz, A.L., Zhang, Y., and Steenhuis, T.S. (2013). Quantification of colloid retention and release by straining and energy minima in variably saturated porous media. Environmental Science & Technology 47(15), 8256-8264.
Sang, W., Stoof, C.R., Zhang, W., Morales, V.L., Gao, B., Kay, R.W., Liu, L., Zhang, Y., and Steenhuis, T.S. (2014). Effect of hydrofracking fluid on colloid transport in the unsaturated zone. Environmental Science & Technology 48(14), 8266-8274.
Schrader, S., H. Rogasik, I. Onasch, and Jégou, D. (2007). Assessment of soil structural differentiation around earthworm burrows by means of X-ray computed tomography and scanning electron microscopy. Geoderma 137: 378-387.
Seshadri, S., Han, T., Krumins, V, Fennell, D.E., and Mainelis, G. 2009. Application of ATP bioluminescence method to characterize performance of bioaerosol sampling devices Journal of Aerosol Science, 40: 113-121.
Sims, J. T., Simard, R. R., and Joern, B. C. (1998). Phosphorus Loss in Agricultural Drainage: Historical Perspective and Current Research. J. Environ. Qual., 27(2): 277–293.
Singh, B., and M. Grafe. (eds.) 2010. Synchrotron-based Techniques in Soils and Sediments. Elsevier.
Smil, V., 2000. Phosphorus in the environment: natural flows and human interferences. Annu. Rev. Energy Environ., 25, 53–88.
Stewart, S.L., Grinshpun, S.A., Willeke, K., Terzieva, S., Ulevicius, V., and Donnelly, J. (1995). Effect of impact stress on microbial recovery on an agar surface. Appl. Environ. Microbiol., 61(4): 1232-1239.
Tang, J.W. (2009). The effect of environmental parameters on the survival of airborne infectious agents. J. R. Sco. Interface, 6: S697-S702.
Therkorn, J., Thomas, N., Calderon, L., Scheinbeim, J., and Mainelis, G. (2017a). Design and Development of a Passive Bioaerosol Sampler using Polarized Ferroelectric Polymer Film. J. Aerosol. Sci., 105:128–144.
Therkorn, J., Thomas, N., Scheinbeim, J., and Mainelis, G. (2017b) Field performance of a novel passive bioaerosol sampler using polarized ferroelectric polymer films, Aerosol Science and Technology, 51:7, 787-800.
Thomas, R.J., Webber, D., Hopkins, R., Frost, A., Laws, T., Jayasekera, P.N., and Atkins, T. (2011). The Cell Membrane as a Major Site of Damage during Aerosolization of Escherichia coli. Appl. Environ. Microbiol., 77(3): 920-925.
Tian, Y., Liu, C.-H., Smucker, A.J.M., Li, H., Zhang, W. (2015). Plant root exudates decrease mobility of smectite colloids in porous media in contrast to humic acid. Soil Science Society of America Journal 79(2), 467-475.
Udawatta, R.P., C.J. Gantzer, S.H. Anderson, and Assouline, S. (2016). Synchrotron microtomographic quantification of geometrical soil pore characteristics affected by compaction. SOIL 2:211-220. DOI:10.5194/soil-2-211-2016
Udawatta, R.P., S.H. Anderson, C.J. Gantzer, and S. Assouline, S. (2013). Computed tomographic evaluation of earth materials with varying resolution. Pp 97-112. S.H. Anderson and W. Hopmans (Eds.) Soil-Water-Root Processes: Advances in Tomography Imaging. SSSA Special Publication 61. Soil. Sci. Soc. Am. Inc. Madison, WI.
Udawatta, R.P., C.J. Gantzer, S.H. Anderson, A.M. Rossi, R.C. Graham, and Ketcham, R.A. (2012). Three dimensional CT-measured geometrical pore parameters in a moraine weathering chronosequence. Soil Science 177:506-516. DOI: 10.1097/SS.0b013e31825f20c6
Udawatta, R.P., C.J. Gantzer, S.H. Anderson, and H.E. Garrett. (2008a). Agroforestry and grass buffer effects on high resolution X-ray CT-measured pore characteristics. Soil Science Society of America Journal 72:295-304. DOI:10.2136/sssaj2007.0057
Udawatta, R.P., S.H. Anderson, C.J. Gantzer, and H.E. Garrett. (2008b). Influence of prairie restoration on CT-measured soil pore characteristics. Journal of Environmental Quality 37:219-228. DOI:10.2134/jeq2007.0227
Walser, S.M., Gerstner, D.G., Brenner, B., Bünger, J., Eikmann, T., Janssen, B., Kolb, S., Kolk, A., Nowak, D., and Raulf, M. (2015). Evaluation of exposure–response relationships for health effects of microbial bioaerosols–a systematic review. International journal of hygiene and environmental health, 218(7): 577-589.
Wang, D., Ge, L., He, J., Zhang, W., Jaisi, D.P., and Zhou, D. (2014a). Hyperexponential and nonmonotonic retention of polyvinylpyrrolidone-coated silver nanoparticles in an Ultisol. Journal of Contaminant Hydrology 164(0), 35-48.
Wang, D., Su, C., Zhang, W., Hao, X., Cang, L., Wang, Y., and Zhou, D. (2014b). Laboratory assessment of the mobility of water-dispersed engineered nanoparticles in a red soil (Ultisol). Journal of Hydrology 519, Part B, 1677-1687.
Wang, D., Zhang, W., Hao, X., and Zhou, D. (2013a). Transport of biochar particles in saturated granular media: Effects of pyrolysis temperature and particle size. Environmental Science & Technology 47(2), 821-828.
Wang, D., Zhang, W., and Zhou, D.M. (2013b). Antagonistic effects of humic acid and iron oxyhydroxide grain-coating on biochar nanoparticle transport in saturated sand. Environmental Science & Technology 47(10), 5154-5161.
Wang, J., Odinga, E.S., Zhang, W., Zhou, X., Yang, B., Waigi, M.G., and Gao, Y., (2019a). Polyaromatic hydrocarbons in biochars and human health risks of food crops grown in biochar-amended soils: A synthesis study. Environment International 130, 104899.
Wang, Y., Zhang, W., Shang, J., Shen, C., and Joseph, S.D. (2019b). Chemical aging changed aggregation kinetics and transport of biochar colloids. Environmental Science & Technology 53(14), 8136-8146.
Wang, Z., Reponen, T., Grinshpun, S. A., Gorny, R. L., and Willeke, K. (2001). Effect of Sampling Time and Air Humidity on the Bioefficiency of Filter Samplers for Bioaerosol Collection. J. Aerosol Sci., 32: 661-674.
Weeks, J.J., Jr. and Hettiarachchi, G.M. (2019). A Review of the Latest in Phosphorus Fertilizer Technology: Possibilities and Pragmatism. J. Environ. Qual. doi:10.2134/jeq2019.02.0067.
West, J., and Kimber, R. (2015). Innovations in air sampling to detect plant pathogens, The Annals of Applied Biology. 166: 4-17.
Willeke, K., and Macher, J. M. (1999). Air Sampling. In: Bioaerosols: Assessment and Control. (eds. J. Macher), Cincinnati, OH, American Conference of Governmental Industrial Hygienists. p 11-1--11-25.
Wildenschild, D., J.W. Hopmans, C.M.P. Vaz, M.L. Rivers, D. Rikard, and Christensen, B.S.B. (2002). Using X-ray computed tomography in hydrology: systems, resolutions, and limitations. J. Hydrol. 267:285-297.
Wu,Y., Li, W., and Sparks, D.L. (2015). The effects of iron(II) on the kinetics of arsenic oxidation and sorption on manganese oxides. J. Colloid Interface Sci. 457:319-28.
Yao, M. and Mainelis, G. (2006). Investigation of Cut-off Sizes and Collection Efficiencies of Portable Microbial Samplers. Aerosol Sci. Technol., 40: 595–606.
Yao, M., Mainelis, G., and An, H. R. (2005). Inactivation of Microorganisms Using Electrostatic Fields. Environ. Sci. Technol., 39: 3338–3344.
Young, I.M., and Crawford, J.W. (2004). Interactions and self-organization in the soil-microbe complex. Science 304:1634-1637.
Zhang, X., L.K. Leeks, A.G. Bengough, J.W. Crawford, and Young, I.M. (2005). Determination of soil hydraulic conductivity with the lattice Boltzmann method and soil thin-section technique. J. Hydrol. 306:59-70.
Zentner, R.J. (1966). Physical and chemical stresses of aerosolisation. Bacteriol. Rev., 30: 551-557.
Zevi, Y., Gao, B., Zhang, W., Morales, V.L., Cakmak, M.E., Medrano, E.A., Sang, W., and Steenhuis, T.S. (2012). Colloid retention at the meniscus-wall contact line in an open microchannel. Water Research 46(2), 295-306.
Zhang, W., Isaacson, C.W., Rattanaudompol, U., Powell, T.B., and Bouchard, D. (2012). Fullerene nanoparticles exhibit greater retention in freshwater sediment than in model porous media. Water Research 46(9), 2992-3004.
Zhang, X., Leeks, L.K., Bengough, A.G., Crawford, J.W. , and Young, I.M. (2005). Determination of soil hydraulic conductivity with the lattice Boltzmann method and soil thin-section technique. J. Hydrol. 306:59-70.
Zhang, W., Rattanaudompol, U., Li, H., and Bouchard, D. (2013). Effect of humic and fulvic acids on aggregation of aqu/nC60 nanoparticles. Water Research 47(5), 1793-1802.
Zhao, Y., Aarnink, A.J.A., Doornenbal, P., Huynh, T.T.T., Groot Koerkamp, P.W.G., de Jong, M.C.M., and Landman, W.J.M. (2011a). Investigation of the efficiencies of bioaerosol samplers for collecting aerosolized bacteria using a fluorescent tracer. I: effects of non-sampling processes on bacterial culturability. Aerosol Sci. Technol., 45(3): 423-431.
Zhao, Y., Aarnink, A.J.A., Doornenbal, P., Huynh, T.T.T., Groot Koerkamp, P.W.G., Landman, W.J.M., and de Jong, M.C.M. (2011b). Investigation of the efficiencies of bioaerosol samplers for collecting aerosolized bacteria using a fluorescent tracer. II: sampling efficiency and half-life time., Aerosol Sci. Technol., 45(3): 432-442.
Zhu, X., Chen, B., Zhu, L., and Xing, B. (2017). Effects and mechanisms of biochar-microbe interactions in soil improvement and pollution remediation: A review. Environmental Pollution 227, 98-115.