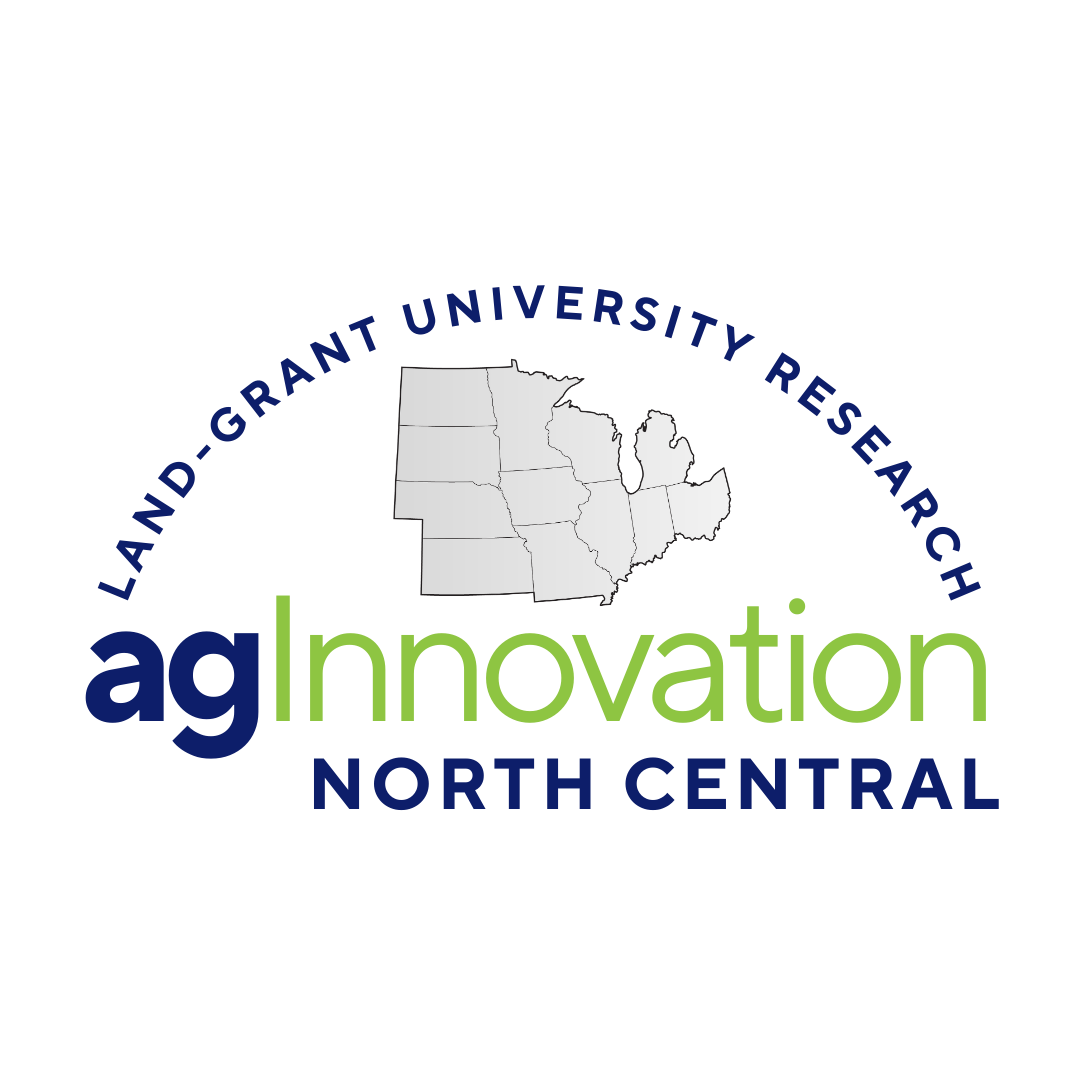
NC1204: Advancement of Brassica carinata
(Multistate Research Project)
Status: Inactive/Terminating
NC1204: Advancement of Brassica carinata
Duration: 10/01/2016 to 09/30/2021
Administrative Advisor(s):
NIFA Reps:
Non-Technical Summary
Statement of Issues and Justification
The need, as indicated by stakeholders. Since the late 1970’s, the United States and many other countries have strived to develop technologies to convert renewable resources into fuels and chemicals that can sustainably and economically replace petroleum based products. The most important outcome of these efforts has been creation of the fuel ethanol industry, produced primarily from corn (US) and sugarcane (Brazil). The US currently produces 13-15 billion gallons of ethanol annually, which represents ~10% of gasoline consumption. A second outcome has been development of biodiesel production from vegetable oils (primarily soybean) and animal fats. The 1.3 billion gallons of biodiesel represents ~2% of annual diesel use in the US. The ethanol industry is currently beginning commercialization of second generation biofuels (ethanol produced from lignocellulose). The challenge with this approach is the “blend wall” that EPA has established by allowing only 10-15% ethanol mixtures in gasoline for non-flex fuel vehicles. To overcome this issue, efforts are also underway to convert biomass and algal oil into drop-in biofuels, which are molecules identical to their petroleum equivalents. These drop-in biofuels could be seamlessly incorporated into the existing petroleum fuel infrastructure. The challenges to this point have been: 1) sourcing biomass and/or algal oil at a sufficient scale and price point, and 2) developing efficient and economical conversion processes. An alternative to lignocellulosic biomass and algal oil as renewable feedstocks for biofuel production are plant-based, non-food oilseeds (NFOS). Several plants produce long-chain oils that are not suitable for food or feed use, but are ideal for conversion into drop-in biofuels. These include many plants in the mustard family such as carinata, as well as camelina. Equally significant is that companies such as ARA and UOP have already demonstrated economical processes to convert NFOS into jet fuel and diesel fuel equivalents. These relatively simple hydro-treatment processes are estimated to account for only 10% of the total cost of the final fuel. Moreover, many of these fuels have already successfully completed certification processes required by military and commercial airlines. The primary hurdle standing in the way of commercial deployment of NFOS-based drop-in fuels is the lack of sufficient supplies of the crops themselves. The cost of the feedstock can account for as much as 90% of the total production costs of the fuel. Thus there is a need for integrated research and development to overcome supply chain barriers to establish an economical and sustainable supply of NFOS. This will require research to achieve the following outcomes: improved varieties, knowledge of varieties that perform best in different locations, demonstrated agronomic practices (including fertility, weed and pest control), rotational effects of these alternative crops, understanding of economic potential and life cycle impacts, defined crop insurance programs, knowledge of how to maximize value and use of meal co-products, and scale-up and commercialization plans. The primary initial stakeholders seeking additional sources of bio-based, drop-in fuels are the Departments of Agriculture and Energy, along with the US Navy, who established the Farm to Fleet Initiative in 2010. This initiative has a goal of obtaining one-half of its fuel from non-food bio-feedstocks by the year 2020, which will require 700+ million gallons per year. To this end, biofuels are to be purchased as part of all DOD domestic solicitations. The US Navy promoted this goal in 2012 during the Great Green Fleet demonstration of biofuels at “Rim of the Pacific” maritime exercise. The carrier strike force was fueled by 50% biofuels, and performance was slightly better than conventional fuels. In the spring of 2013 the Navy initiated the biofuel pilot program under Defense Production Act. In the spring of 2014 the US Navy announced that biofuels will be incorporated into all solicitations for jet engine and marine diesel fuels, and last fall announced the announce purchase of 100 MGY of military grade biofuels at an average price of $3.45/gal beginning in 2016/17. Plans for 2016 include fully deploying the Great Green Fleet full deployment using 50% renewable fuels. Additional stakeholders from the fuel supply perspective include national and international commercial airlines, who are seeking renewable sources of jet fuel. Stakeholders in the farm-to-fuel part of the value chain include farmers, rural communities, and the Federal government. To increase the availability of renewable fuels for consumers, the USDA established the Rural Energy for America (REAP) Program in the early 2000s to grow rural economies by developing a domestic biofuel industry. Initial production trials with NFOS has been conducted with producers in both the North Central and Southeastern parts of the US over the past several years. Results have been encouraging, but the research described above is needed to expand crop production capabilities to the levels needed. The importance of the work, and what the consequences are if it is not done. The over-arching objective of this project is to perform needed research and demonstration to enable commercial deployment of NFOS as rotational crops, while providing in-edible plant oil for advanced biofuel production and another source of high protein feed for animal and fish production. NFOS represent a significant new opportunity for the US economy, ranging from production agriculture (new crops in wheat rotations and livestock feeding operations) to expanded production of drop-in biofuels to the development of an aquaculture industry. Two specific NFOS crops, Ethiopian mustard (Brassica carinata, aka carinata) and camelina [Camelina sativa (L.) Crantz., Brassicaceae], are the focus of this effort. This proposal brings together a broad range of expertise from across the nation to address key research, development, and scale-up issues that must be overcome to allow these new opportunities to be profitably and sustainably capitalized upon. The mission of this NCRA is to develop a future industry in NFOS crops suitable for conversion to military and civilian liquid fuels and co-products suitable for animal production. Our vision is to conduct this work in cooperation with organizations including other universities, USDA, United States Navy, the Sun Grant Program, and commercial partners. Strategic goals include: 1) identifying improved NFOS varieties that are adapted for production in specific ecoregions, 2) determining appropriate agronomic practices for NFOS crop performance in rotational farming systems, 3) demonstrating suitability of NFOS crops for biofuel and high protein feed production, 4) defining the economic potential and life cycle impacts of NFOS crops. The unfortunate consequences of not performing this work will be the loss of the opportunity to provide new rotational crops for production of biobased fuels and high protein feeds, as well as the rural economic development this could provide. The technical feasibility of the research. For most biofuel projects, development of an efficient and economical conversion technology is the biggest hurdle. Supplies of lignocellulosic feedstocks are already available, and so only cost, competing uses, and logistics are factors. For this project the hydrotreating conversion technology is already available and demonstrated, and the resulting fuels are being certified. The critical need is availability of NFOS feedstocks that are produced in a sustainable manner that can be integrated into current farming practices. To achieve a sufficient supply of NFOS and ensure that the resulting meal co-product will have valuable market uses, we will address the issues described below. Fortunately, the technical feasibility of achieving these objectives is high, as they have been used for many decades to develop other crops and establish valuable uses for processing co-products. For example, methodologies for plant breeding, variety selection, and agronomy are well known. Similarly, livestock feeding trials have established protocols, as do the methods used for technoeconomic and life cycle analyses. Perhaps the most challenging aspect of this proposed activity will be in processing the co-product meals to reduce the levels of glucosinolates, which are anti-nutritional compounds found in many Brassica species. However in preliminary work investigators have demonstrated that various physical, chemical, and microbial methods can substantially reduce glucosinolate levels. We anticipate that optimization of these methods will both reduce/eliminate glucosinolates and generate feeds with higher protein levels. This will allow for higher feed inclusion rates and higher value assigned to the co-product meals. Anticipated deliverables include key elements in the value chain required to commercialize production, processing, and marketing of NFOS as new rotational crops for the US. These are described below. Variety selection: Protocols have been established and implemented to accurately evaluate varieties for performance across several ecoregions. The key milestone will be identifying top yielding varieties for each geography and climate. The key indicator will be adoption of these varieties in crop rotations. Agronomy practices: Baseline production methods are being adapted for use in various ecoregions. The key milestone will be developing a comprehensive suite of agronomic practices for use by producers. The key indicator will be adoption of these agronomic practices by producers. Meal processing: Oilseed meals are being processed by established physical, chemical, and biological protocols to develop nutritionally enhanced meals, with lower levels of anti-nutritional compounds. The key milestone will be developing a procedure to enhance protein content and digestibility of these meals, while reducing or eliminating anti-nutritional factors. The key indicator will be adoption of this technology by meal processing companies to produce commercial quantities of these microbially enhanced feeds. Livestock feeding: Feeding trials are underway using standard methods on beef and dairy cattle, as well as fish. The key milestone will be identifying optimal feed inclusion rates for the oilseed meals, either in their raw state or after microbial conversion to reduce anti-nutritional factors. The key indicator will be adoption of these feeds by livestock producers. Economic, insurance, and life cycle analysis: Data from the work above is being used to characterize the economics, insurability, and LCA of the non-food oilseed production system. The key milestone will be linking all elements of this value chain together to demonstrate technical, economic, and environmental feasibility and sustainability. The key indicator will be adoption of this system as part of US agriculture. The advantages for doing the work as a multistate effort. To produce sufficient quantities of feedstock will require acreage spread across multiple ecoregions. This distributed production will also help maintain a more stable production base in light of climatic variability, and will expand the economic development benefits to a greater and more diverse population base. Since each ecoregion has unique characteristics, it is critical that this NRCA effort be conducted as a multistate effort, to bring local research and development expertise to the table. Moreover, the commercial partners developing improved oilseed varieties, scaling up production of seed, handling, production contracts, logistics, and commercializing conversion processes are already located in multiple states. Thus, bringing together a diverse team from across the US will be the most effective way to achieve the stated objectives. Beyond the Experiment Station researchers from several states who will be involved in this project, below are listed other collaborators: • USDA Agricultural Research Service • Midwest Aviation Sustainable Biofuels Initiative • U.S. Navy Energy Security Program • Sun Grant Initiative • Agrisoma Biosciences LLC, Saskatoon, CA (Carinata breeding and commercial deployment) • Sustainable Oils (Global Clean Energy Holding), Bozeman, MT: Camelina breeding and commercial deployment • Canterra Seeds, Winnipeg, Canada: Carinata seed production and sales. • Paterson Grain/PGF Biofuels, Winnipeg, Canada: Carinata production contracts, procurement, logistics, oilseed crushing • Stengel Oils, Milbank, SD: Cold press oil recovery • Blue Sun Energy, St. Joseph, MO: Commercial Biodiesel Production • Applied Research Associates (ARA), Panama City, FL: Biofuel Technology Development for ReadiJet ® and ReadiDiesel ® • Prairie AquaTech, Brookings, SD: Meal processing and utilization What the likely impacts will be from successfully completing the work. Rural economic growth in semi-arid regions of Great Plains could be improved by an expansion into production of NFOS that would serve as feedstocks for production of biofuel and high protein livestock feed. Similarly, production of NFOS could serve as important winter crops for the Southeastern US. These crops could be valuable new additions to existing crop rotation programs, thereby increasing land productivity and making use of available moisture and fertility resources. Local crushing and processing facilities for these NFOS crops would keep the economic benefits in rural areas. Advanced biofuel processing plants could be developed in these regions, much the same as corn-ethanol plants have brought high-paying local jobs and economic development to the Midwest. For private consumers and the military, the benefits would be increased supply of sustainably produced biofuels at competitive prices. For livestock and aquaculture producers the benefit would be an additional source of highly digestible protein feeds for animal and fish production.
Related, Current and Previous Work
Interest in producing 3rd generation biofuels from non-food oilseed crops has intensified over the past 5-6 years, driven initially by the Department of Defense (DOD; especially the Navy), who desire a more sustainable and diversified source of liquid fuels (eg, JP5, JP8, and Jet A) (Lane, 2012; Biofuels Digest, 2012). The Departments of Energy and Agriculture have also joined in support of this effort, providing funding for research as well as scale-up and commercialization (U.S. Department of Energy, 2012; U.S. Department of Agriculture, 2012). The commercial aviation industry is also very interested in biofuels that are competitively priced, environmentally acceptable, and meet global performance and safety standards (Stein, 2012). In fact, the U.S. Department of Agriculture (USDA), Airlines for America, Inc. (A4A), and the Boeing Company (Boeing) signed a resolution formalizing their commitment to work together on the “Farm to Fly” initiative (USDA, 2012). Production of biofuels from non-food oilseed crops will help meet the renewable fuel production requirement established in the RFSII legislation, while diversifying agricultural production systems throughout the county and spreading the economic development benefits across the same areas (Nelson and Kephart, 2011). Fortunately, processes to convert plant oils into biofuels are relatively well established. Many methods are now being considered as commercially viable and typically include some type of catalytic conversion (Wang and Tao, 2016). For example, Honeywell Universal Oil Products (UOP) and Advanced Research Associates (ARA) have demonstrated their catalytic biofuel conversion processes at the pilot scale, and are proceeding through the fuel certification process with DOD as well as commercial airlines (Glover and Heimlich, 2009). Current economic issues mostly involve the feedstock supply chain. Matters such as the method of oil recovery and the size and distribution of the processing facilities impact the feasibly of production. For example, the complete process could occur in large, centralized refineries that collect oilseeds from the surrounding region. It is likely that such a process would use conventional extraction processes (eg. hexane extraction) to recover >95% of the oil from the meal. The other extreme would be a network of smaller processing systems to recover most of the oil in the meal using a less capital intensive process (eg. expeller or cold press), and then shipping the oil to a larger, centralized conversion and refining facility. The meals resulting from such processing could thus have minimal oil (from solvent extraction) or contain a low-moderate levels of oil (from pressing). Over the past decade and more, a broad range of oilseed crops have been evaluated for biofuel production. Some of these have been traditional crops such as sunflowers, flax, and soybeans. However, concerns over the food vs fuel issues have, for all practical purposes, eliminated these crops for consideration in producing advanced biofuels. Therefore, focus has shifted to non-food oilseed crops (e.g., carinata, camelina, crambe, etc). This broad range of potential oilseed crops provides the opportunity to match the best plant and variety for each location (soil type, climate, etc.). Plant selection will also be based on yield, oil characteristics, and the specific type of processing to be used and fuel to be produced. Brassica carinata (aka. carinata) appears to have significant advantages over other non-food oilseed crops for both agronomic and fuel production perspectives. The crop has a broad geographic and climatic range, with yields similar to or higher than other non-food oilseeds. Perhaps the greatest advantage, however, is the high content of C-22 erucic acid, which can be more efficiently converted into 10-11 carbon length hydrocarbons than shorter chain fatty acids. The resulting biofuels have excellent low temperature tolerance and other desired traits (eg. viscosity, acid number, flash point, density) without additives (Wang and Tao, 2016). Carinata meal also has higher protein content than many other alternative oilseeds (Xin and Yu, 2013), thus providing a higher value co-product. Agrisoma Biosciences, Inc has conducted breeding and agronomy research on carinata for over a decade, initially in western Canada, but for the past 4 years in the north central and southeastern regions of the US. Agrisoma has the world’s largest collection of carinata germplasm (>8,000 varieties), and has developed and released two commercial varieties at this point. In collaboration with university researchers in the US and Canada, Agrisoma is evaluating performance of additional varieties that have been bred for high yield in various ecoregions. Breeding research is also focused on enhancing tolerance cold weather, drought, and to dicamba and other herbicides. Agronomy and management practices for carinata that were developed in western Canada are being evaluated and modified as needed for different ecoregions. Weed and pest management options also must be optimized for this new crop. Researchers are also beginning to investigate crop rotation options for carinata, as well as the life cycle impacts of deployment. Even though carinata is not an edible oilseed (due to the high content of erucic acid) and can be grown on marginal land, the use of arable lands for carinata production could still lead to food vs. fuel criticism, because carinata production could divert land from food to fuel production. Fortunately, Australian researchers have noted that glucosinolates released into the soil during production provide some level of nematode control (Potter et al, 1998; Osler et al, 2000), which can increase yields of subsequent crops in the rotation (eg. wheat). Therefore diversifying rotations to include carinata may benefit other crops through biofumigation. Carinata meal is currently being commercially marketed as BioFence® in Europe to control fungi that cause root-rot (Morales-Rodriguez et al, 2015; Mocali et al, 2015). The biofumigant impacts of carinata crop residue in different ecoregions (eg. soils and climates) has not been studied. Carinata inclusion in rotations could help improve overall water quality through nutrient scavenging and water retention through deep root systems (Zegada-Lizarazu and Monti, 2011). The rotational management of carinata is not well developed and the rotational effects of crop combinations are currently not available. Carinata is capable of self-pollination. However, its relationship with pollinators is thought to be similar to canola. According to the Canola Council of Canada: “canola is good for bees, and bees are good for canola.” For canola, pollinators increase yield, seed set and weight, and profitability while canola provides a nutrient-dense pollen source, plentiful blooms with a good sugar profile, and high quality honey for pollinators. Canola has been known to strengthen weak colonies and help them fight disease. Honey produced from brassicas is general light in color, mild flavored, and high in volume but may crystallize easily. The relationship between carinata and pollinators has not been scientifically studied but is believed to be mutually beneficial. An important economic aspect is that carinata meal can be efficiently used as a livestock feed constituent. Carinata meal is low in fibre and high in crude protein. Carinata meal proteins are digestible by a wide array of livestock, including: ruminants, poultry, swine, and fish (Jain and Gupta, 2013; Xin and Yu, 2014; Sackey et al, 2015; Rajapakse, 2015). However, animal feed inclusion levels are still being studied and are limited due to glucosinolate content (Tripathi and Mishra, 2007). Therefore research has been initiated to improve the feed value of carinata oilseed meal by: 1) reducing levels of residual oils and glucosinolates, 2) increasing protein and amino acid levels, 3) reducing anti-nutritional factor levels, and 4) enhancing digestibility. Proof-of-concept for this approach has been demonstrated on soybean meal, by increasing the protein content from 50% to over 65% by using a fungal incubation process. If this technology can be successfully applied to carinata meal, inclusion levels can be increased in dairy, beef, and swine rations. Moreover, it is possible that a highly digestible carinata meal could find applications in more lucrative opportunities such as a replacement for fish meal in aquaculture and soybean meal in swine and poultry diets. Fish meal prices range from $1,600-2,600/ton (2014-2015), compared to $300-400/ton for soybean meal (SBM), and substantially less for carinata meal (due to the presence of glucosinolates and slightly less protein). Market demand for fishmeal is rising with aquaculture production (7-9%/yr) and competing livestock feed markets. Wild harvest of fishmeal is declining due to decades of overharvest and continued market competition for available supplies (FAO, 2013). Thus, there is a tremendous market opportunity for converting non-food oilseed meals into a replacement for fishmeal and food-grade oilseed meals. Unconverted carinata meal has been used in livestock feeding trials in both the US and Canada. Glucosinolates currently limit feed inclusion rates, however energy and protein digestibility of carinata meal compares favorably with soybean meal in beef and dairy cattle trials. The relative palatability of carinata meal is unknown. Research with monogastrics such as fish and swine will commence when treated carinata meal is available. Unpublished trials with fungal converted soybean meal in fish and swine have shown improved performance compared to unconverted soybean meal. Cursory trials of ensiling carinata meal have shown promising results with decreased glucosinolate levels and increased digestibility in ruminants. We anticipate that treating carinata meal will enable higher feed inclusion levels and better performance, leading to higher economic value of this meal. Preliminary farm-to-fuel life-cycle analyses show that greatest variability and most prominent controls associated with the environmental impacts of carinata production and products are within the on-the-farm production phases, similar to other biofuels reported within the literature. For example, carinata agricultural production in unpublished GREET results accounts for approximately 47% of sulfur dioxide emissions but could be as low as 26% or as high as 68% depending on agricultural management. Other environmental impacts show similarly high levels of variability. Therefore, optimization and sound agricultural management for carinata production is paramount to achieving sustainability. References Biofuels Digest, “Military signals advanced biofuel demand: 336 million gallons per year by 2020.” 2010. Available at http://www.biofuelsdigest.com/bdigest/2010/12/16/military-advanced-biofuelsdemand-336-million-gallons-by-2020-voices-from-the-bio-pacific-rim-summit/. [Accessed June 23, 2012] FAO, “Fish to 2030: Prospects for fisheries and aquaculture.” World Bank Report Number 83177-GLB, p 102, 2013. Available at: http://www.fao.org /docrep/019/i3640e/i3640e.pdf Glover, B. and J. Heimlich, “Farm to Fly: Towards a Comprehensive Sustainable Aviation Biofuels Rural Development Plan.” 2009. Available at http://www.airlines.org/Energy/Alternativefuels/Documents/farmtoFly Presentation 071410.pdf. [Accessed June 20, 2011] Jain, S.M. and S.D. Gupta (ed.), “Biotechnology of neglected and underutilized crops.” Springer Science and Business Media, p 459, 2013. Lane, Jim, “Aviation and Military biofuels: new thinking on finance, fuels.” 2012, Available at http://www.biofuelsdigest.com/bdigest/2012/02/08/aviation-andmilitary-biofuels-new-thinking-on-finance-fuels/ [Accessed June 24, 2012] Mocali, S., S. Landi, G. Curto, E. Dallavalle, A. Infantino, C. Colzi, G. D’Errico, P.F. Roversi, L. D’Avino, and L. Lazzeri, “Resilience of soil microbial and nematode communities after biofumigant treatment with defatted seed meals.” Industrial Crops and Products, 75 (A) 79-90, 2015. Available at: http://www.sciencedirect.com/science/article/pii/S092666901530039X Morales-Rodiguez, Carmen, A.M. Vettraino, and Andrea Vannini, “Efficacy of biofumigation with Brassica carinata commercial pellets (BioFence®) to control vegetative and reproductive structures of Phytophthora cinnamomi.” Plant Disease, 2015. Available at: http://apsjournals.apsnet.org/doi/abs/10.1094/PDIS-03-15-0245-RE Nelson, Robert J., and Kevin D. Kephart, “Feedstock Development in North Central Region for Green Diesel and Jet Fuels,” May 2011. Available at http://ncsungrant.sdstate.org/upload/North-Central-Region-Oilseed-White-Paper060811-2.pdf. [Accessed June 18, 2012] Osler, Graham H.R., Petra C.J. van Vliet, Craig S. Gauci, and Lynette K. Abbott, “Changes in free living soil nematode and micro-anthropod communities under a canola-wheat-lupin rotation in Western Australia.” Journal of Soil Research, 38 (1) p 47-60, 2000. Available at: http://www.publish.csiro.au/paper/SR99050 Potter, Mark J., Kerrie Davies, and Anthony J. Rathjen, “Suppressive impact of glucosinolates in Brassica vegetative tissues on root lesion nematode pratylenchus neglectus.” Journal of Chemistry Ecology, 24 (1) p 67-80, 1998. Available at: http://link.springer.com/article/10.1023%2FA%3A1022336812240 Rajapaske, Banuka Y., “Nutritive evaluation of mechanically-pressed camelina (Camelina sativa), carinata (Brassica carinata) and soybean (Glycine max) meals for broiler chickens.” M.S. Thesis, Dalhousie University, Halifax, Nova Scotia, p 246, April 2015. Available at: http://dalspace.library.dal.ca/xmlui/bitstream/handle/10222/56764/ Rajapakse-Banuka-MSc-AGRI-April-2015.pdf?sequence=3&isAllowed=y Sackey, A., E.E. Grings, D.W. Brake, and K. Muthukumarappan, “Effect of processing conditions on nutrient disappearance of cold-pressed and hexane-extracted camelina and carinata meals in vitro.” iGrow Beef Reports, p 5, 2015. Available at: https://www.sdstate.edu/ars/species/beef/beef-reports/upload/05-Adolf-oilseed-processing-04_21_2015-pm.pdf Stein, Luke, “Economic Analysis of Potential Camelina Oil Crop Supplies in the Northwest US.” Selected paper presented at Western Agricultural Economics Association, 2012 Annual Meetings, Park City, Utah, June 20-22, 2012. Tripathi, M.K. and A.S. Mishra, “Glucosinolates in animal nutrition: A review.” Animal Feed Science and Technology, 132 (1-2) p 1-27, 2007. Available at: http://www.sciencedirect.com/science/article/pii/S0377840106001362 U.S. Department of Agriculture, (USDA), “Agriculture and Aviation: Partners in Prosperity.” January 2012. Available at http://www.usda.gov/documents/usdafarm-to-fly-report-jan-2012.pdf. [Accessed June 24, 2012] U.S. Department of Energy. “State & Federal Incentives & Laws: All Incentives and Laws Sorted by Type,” Washington DC, 2012. Available at http://www.afdc.energy.gov/afdc/laws/matrix/incentive . [Accessed June 18, 2012] Wang, Wei-Cheng and Ling Tao, “Bio-jet fuel conversion technologies.” Renewable and Sustainable Energy Reviews, 53, p 801-822, 2016. Available at: http://www.sciencedirect.com/science/article/pii/S1364032115009867 Xin, Hangshu and Peiqiang Yu, “Rumen degradation, intestinal and total digestion characteristics and metabolizable protein supply of carinata meal (a non-conventional feed resource) in comparison with canola meal.” Animal Feed Science and Technology, 191 p 106-110, 2014. Available at: http://www.animalfeedscience.com/article/S0377-8401%2814%2900041-8/pdf Xin, H. and P. Yu, “Chemical profile, energy values, and protein molecular structure characteristics of biofuel/bio-oil co-products (carinata meal) in comparison with canola meal.” Journal of Agricultural Food Chemistry, 61 (16) p 3926-33, 2013. Available at: http://pubs.acs.org/doi/abs/10.1021/jf400028n Zegada-Lizarazu, Walter and Andrea Monti, “Energy crops in rotation. A review.” Biomass and Bioenergy 35 p 12-25, 2011. Available at: http://www.sciencedirect.com/science/article/pii/S0961953410002588
Objectives
-
Identify optimal carinata varieties for specific geographies and climates
-
Determine appropriate agronomic practices such as planting dates, planting densities, fertilization levels
-
Develop appropriate weed and pest control strategies
-
Assess the effects of carinata on pollinator health
-
Ascertain rotational effects with other crops
-
Develop methods to enhance the nutritional content of carinata meal while reducing levels of anti-nutritional compounds
-
Evaluate carinata meal in livestock and aquaculture diets to determine optimal feed inclusion rates and animal/fish performance
-
Quantify costs and returns of carinata production to establish economic feasibility
-
Assess live cycle impacts of carinata production and utilization for the full value chain
Methods
Specific objectives and the methodologies to accomplish them are listed below. Identify optimal carinata varieties for specific geographies and climates Plant Scientists from the participating universities will conduct adaptability and performance evaluation trials of Brassica carinata (Ethiopian mustard, carinata) varieties developed by Agrisoma Biosciences, Inc. according to Material Transfer/Seed Evaluation Agreements signed by the parties. Breeders from Agrisoma will determine varieties to be tested each year for the respective sites, and will provide sufficient seed in advance of the desired planting dates. The seed provided will be pre-treated with insecticide/fungicide by Agrisoma prior to shipment. Variety plots will be laid out in a randomized complete block with sufficient replications to obtain sufficient statistical power. Plot dimensions will be at the discretion of each university, however a typical layout could include plots that are 4.1 ft wide (seven 7-in rows) by 25 ft long (1.2x7.6 m) or 5 ft wide (six 10-in rows) by 25 ft long (1.5x7.6 m). Planting dates would be in late March to early April in the northern region, and October to November in the southern region. Seeding rate will be six lbs/acre or as recommended by Agrisoma. Seeding depth will be 0.5 to 1.5 in (1.3-3.8 cm), depending on moisture conditions. Weed control will be at the discretion of each university, if needed. Standard seasonal plant health, growth, and productivity measurements will be made. Plots will typically be straight-combined with a small-plot combine as they reach maturity (assume multiple harvest dates will be necessary) and all seed bagged. Seed will be cleaned before weighing. Determine appropriate agronomic practices such as planting dates, planting densities, fertilization levels Plant Scientists from the participating universities will conduct trials to establish appropriate agronomic practices for carinata production. Current Agrisoma production manuals will serve as the basis for evaluating specific practices. Findings from this research will be used to develop agronomy manuals for each eco-region in the US. Locations for agronomy trials will be selected to represent the typical soil types, landscape positions, and prior crop histories for likely commercial production areas. A single carinata variety will be used for all trials at a particular location in a given year. Insecticide/fungicide pre-treated seed will be provided by Agrisoma. Plot size will depend on the specific variable to be tested, but will contain sufficient replications for statistical validity in a randomized complete block design. Seeding rate studies will evaluate same-day planting rates of 6-12 lb/ac (7-14 kg/ha). Seeding date studies will evaluate several dates spread over several weeks, each planted at the same seeding rate. Fertilizer trials will evaluate rates up to 150 lbs (68 kg) of N applied in the form of (NH4)(NO3), with all replicates using the same seeding rate and date with appropriate rates of P2O5 application to ensure that P is not limiting. Weed control will be at the discretion of each university but a typical program might include: Roundup (glyphosate) burndown, Prowl (pendimethalin) pre-plant, Poast (sethoxydim) post-emerge, if needed. In-season measurements include: stand density; days from planting to 50% flower and maturity; lodging (1-9 scale); % seed shatter; plant height; weed pressure; disease and insect incidence (% infestation); seed yield. For nitrogen fertility trials, ten to twelve 0-6 in (15 cm) soil samples will be collected and composited before planting to determine initial fertility levels. Plant response notes and plant samples for nutrient concentration will be collected at the bolting stage. About 15-20 first mature leaves from the growth point will be collected and analyzed for N. Plots will be harvested as they reach maturity, and all seed will be bagged and cleaned before weighing. Develop appropriate weed and pest control strategies University agronomists will conduct a series of herbicide evaluations for crop safety on several varieties of carinata. Seeding rates, planting depth, fertility and other factors will be held constant. Several broadleaf crop herbicides will be applied in replicated plot trials in soils in which weed pressure is present. Treatments will include pre-emergence and post-emergence herbicides. Injury ratings will be taken through the growing season, and plots will be harvested at the end to measure seed yields. Scientists from the participating universities will conduct trials to assess the efficacy of different fungicide and insecticide seed and foliar treatments in research plots located at the respective university farms. For the trials, a susceptible variety of carinata will be seeded at a rate that is standard for each location. Plots will be arranged in a randomized complete block design with eight replications. Foliar treatments will be sprayed at the recommended label rates and will include an untreated check. The products used for seed treatments will be determined by Agrisoma based on availability. Disease and insect abundance will be assessed and plots will be harvested at the end of the season to measure yields. Assess the effects of carinata on pollinator health It is believed that a carinata will be a valuable bloom source for pollinators, similar to canola, however there is no data to currently support this assumption. Researchers will conduct a series of pollinator studies to determine the potential benefits of carinata on domestic and wild bees/other pollinators. Carinata may fit well into the bloom sequence for both wild and domestic pollinators. The timing of the blooms with respect other factors such as distance to field edges, field size, uncultivated land, water, and hives, as well as, influence of regional crop rotations will be studied. Pollinators increase seed set and yield for canola. To determine if this influence is present in carinata, as well as to determine optimal hive spacing, placement, and introduction/removal – carinata yield and oilseed samples will be taken as a function of distance from domestic hives. Hives will be placed in a wreath experiment configuration and populations will be monitored. Bees and hives will be inspected for health issues (e.g. mites, pesticide exposure, colony disorders) and the pollen they have collected will be analyzed to determine the types of flowers frequented. The interaction between the larval and adult bees and the development and size of hypopharyngeal glands of nurse bees (indicative of pollen nutritive value) will be monitored. Honey will be graded and tested for water content, bacterial, and fungi to determine the quality produced. Wild pollinators will be sampled using monthly sweep net transects and light trap sampling with increased sampling frequency at the beginning, middle, and end of bloom both in the carinata field and selected surrounding locations to compare pollinator utilization and influence on overall health. Ascertain rotational effects with other crops It is important to evaluate various crop rotation strategies to optimally incorporate carinata into existing cropped landscapes to both enhance production of existing crops and provide a new oilseed crop for biofuel and high protein feed production. Crop rotation strategies will be influenced by the climate and soils in a particular region, as well as the equipment and management practices that producers typically use. With small seeded crops like carinata, residue management of the crop prior to carinata is critical to obtain uniform seed placement and emergence. Herbicide residues from the prior crop can also be an important factor in designing rotation strategies. Equally important are the cropping options that could be used following carinata production. Glucosinolates produced by carinata can reduce levels of nematodes and other soil-borne pests, thereby potentially increasing the yield of the following crops, or at least reducing the need for some crop protection products. Another important consideration for crop rotation systems is to minimize displacement of existing food or feed crops. In some regions this may be feasible by use of double-cropping systems, replacement of fallow systems, or even use of carinata for phytoremediation on marginal lands. Long-term crop rotation studies will be conducted in multiple regions to identify appropriate rotational systems that incorporate carinata into existing agricultural systems. Agronomic, economic, and environmental factors will be monitored to develop profitable and sustainable crop rotation strategies. Develop methods to enhance the nutritional content of carinata meal while reducing levels of anti-nutritional compounds Carinata meal is an important co-product, and must be effectively used in feed applications to help ensure economic feasibility and to counter the food vs. fuel argument used to oppose biofuels. While carinata meal is relatively high in protein (40-45%), it also contains high levels of glucosinolates, which are toxic to fish and animals. This will restrict the inclusion level of carinata meal in feeds to 10% or less, and thus limit the economic value of carinata meal. To overcome this limitation, researchers will develop processes to enhance the nutritional value of carinata meal by increasing protein levels and degrading or eliminating glucosinolates. Solvent extracted and cold pressed carinata meal will first be subjected to thermal and/or chemical pretreatments such as extrusion, hot water cook, dilute acid cook, dilute alkali cook, or AFEX treatment. Pretreatment effectiveness will be assessed by a standard saccharification procedure conducted in shake flasks. Analyticals will include levels of fiber, carbohydrates, and glucosinolates. Various commercial enzymes (cellulases and pectinases) will be evaluated to identify the best enzyme cocktail for saccharification. Again, this will be performed in shake flasks, with the same analyticals as listed above. Then carinata meal will be treated by the best pretreatment and enzyme hydrolysis, and subjected to incubation with several filamentous fungi that are known to metabolize a broad range of carbohydrates and generate highly digestible microbial protein. Trials will initially be performed in shake flasks to identify the best fungal strain based on levels of fiber, carbohydrates, glucosinolates, and protein. Trials will then be conducted in bioreactors under optimal levels of aeration and agitation to produce sufficient quantities of high-protein carinata meal for use in fish and livestock feeding trials. Evaluate carinata meal in livestock and aquaculture diets to determine optimal feed inclusion rates and animal/fish performance Raw carinata meal and high-protein carinata meal will be evaluated in livestock (dairy, beef, swine) and fish (trout or tilapia) feeding trials to quantify their values in relation to established feeds such as soybean meal and corn distillers’ grains. An additional treatment of ensiled carinata meal may also be tested for livestock use. The value of carinata meals will be a critical component of the business plans to commercialize this feedstuff industry. Meals will be subjected to palatability trials with livestock and fish using carinata meal basal diets, along with controls not containing carinata. In livestock trials, each animal will be provided with multiple feeds on an ad-libitum basis. After the favorite feed is identified, it will be withdrawn to determine the second most palatable feed, and so forth. In fish trials, individual tanks of fish will be fed separate feeds and pellet counts (provided vs. consumed) will be monitored for 10-14 days. Carinata meal types will be subjected to digestibility trials. Inert tracer compounds will be added to the feed and levels in manure will be monitored. In ruminants, we may also conduct in-vivo digestibility trials by placing nylon pouches containing feed into cannulated rumens for an incubation period. Feeding trials will be used to determine animal or fish performance. Raw carinata meal and high-protein carinata meal will be used to replace protein sources typically used in livestock and fish diets. Feeding trials will be conducted in accordance with each university’s Animal Care Committee guidelines. Feed performance results will be compared to that from more traditional protein sources to establish the market value of carinata meal. This information will be critical to the economic analysis described below. These trials will be used to determine apparent digestibility coefficients (ADC) for protein, energy, and amino acids of untreated, treated, and converted meals. Nutrient flows and apparent feeding value of co-products without anti-nutritional factors will be directly measured. The effect of carinata oil on ruminal fermentation and N recycling, growth performance, feed efficiency, nutrient use and total track digestion, and metabolism will be determined. Quantify costs and returns of carinata production to establish economic feasibility If carinata can be profitably grown in a region, it will diversify and stabilize production agriculture and spur economic development. Production could lead to establishment of a regional supply network of processors, refineries, and meal by-product processing into valuable livestock or aquaculture feeds which would create new businesses and jobs. Research is needed to both establish the profitability of carinata production and to assess potential impacts of establishing a supply chain. Specific economic feasibility objectives include: 1) assessing current oilseed production trends, 2) delineating suitable production areas, 3) identifying current crop rotations, 4) pinpointing factors that influence producers’ planting decisions, 5) developing and updating carinata crop budgets, 6) assessing production impact on crops currently grown in the region, and 7) estimating the potential carinata supply. USDA’s crop reporting district level data will be analyzed to identify trends in acreage, yields, production, prices, and potential comparative advantages of growing carinata. USDA and regional soil and climate data will be used determine crop suitability. Producer surveys will be used to identify prior crop rotations and factors important in deciding to grow (or not to grow) oilseeds. Carinata production budgets will be developed and updated in collaboration with local agronomy, oilseed, and extension specialists. The potential supply of carinata and producer profitability will be based on the number of suitable acres, yields, production costs, profitability of land in alternate use, and risk aversion. Appropriate regional budgets will be developed or revised for selected rotations. Sensitivity analyses will be conducted to identify profitability based on insured risk and insurance availability. The potential supply of carinata will be estimated under alternative profitability, risk, and adoption scenarios. Assess live cycle impacts of carinata production and utilization for the full value chain As carinata feedstock varieties become more productive and as uses expand, the life cycle impacts change. A spatially-specific ISO 14040/14044 compliant field-to-tank life cycle analyses (LCA) will be conducted using both Argonne National Laboratory’s GREET and Pré Consultant’s SimaPro LCA models. The LCA will encompass and contrast the ‘niche’ varieties and locations, variable experimental methods, and chemical use impacts. Existing South Dakota LCA models will be adapted to new test locations and production methods. Recent peer-reviewed and government literature will be monitored and used to update models with results from projects on-going in other locations. New data and results will continue to be added as the project progresses to refine results and build a library of feedstock information. Data will be constructed into inventory datasets and used in locational (niche), regional, and national analyses. Agricultural data and formatted life cycle inventory data acquired on carinata will be submitted to the USDA Life Cycle Assessment Commons (http://www.lcacommons.gov/) following Agricultural Research Service (ARS) and National Agricultural Library (NAL) standards for public and governmental use. Up-to-date LCA model results and summaries will be available to other researchers and used in proposals for additional external funding. LCA model results will be used to develop strategies and recommendations to improve the sustainability and reduce the environmental impact of carinata production. Information from the economic evaluation will be combined with the midpoint environmental and endpoint societal impacts from the LCA to conduct a ‘triple bottom line’ assessment. Based on these results, the production methods and options will be ranked and used to optimize production while reducing overall negative impacts. One or more summaries of LCA results developed will be published in peer-reviewed literature.Measurement of Progress and Results
Outputs
- Top yielding carinata varieties for each ecoregion
- Best management practices for carinata production for each ecoregion
- Yield, hardiness, soil, nutrient efficiency, and GHG data for carinata production
- Herbicide and disease/pest management recommendations for carinata
- Herbicide resistant lines of carinata
- Effects of carinata on pollinator health
- Strategies for rotating carinata with other crops
- Allelopathic and fungicidal benefits of carinata residues for subsequent crops
- Optimal process conditions to enhance carinata meal nutritional content
- Palatability of raw and modified carinata meal for various species
- Feed rates for raw vs modified carinata meal with various species
- Financial risk and profitability of carinata production in different regions
- Potential supply of carinata for various ecoregions
- Local, regional, and national LCA impacts for carinata production
- LCA on-line story maps for potential impacts of carinata
Outcomes or Projected Impacts
- Increased production of specific carinata varieties in each ecoregion
- Greater crop rotational flexibility for producers
- Increased farm profitability
- Improved pollinator health
- Increased availability of highly digestible carinata meal for livestock and aquaculture
- Increased availability of carinata oil for conversion into drop-in biofuels
- Expanded economic development in rural America
- Improved sustainability of biofuel and high protein feed production
Milestones
(2017): Nitrogen fertility recommendations(2017): Optimal bench scale conditions for processing carinata meal
(2017): Beef and dairy feeding results with raw carinata meal
(2017): Initial life cycle analysis completed
(2018): Aquaculture feeding trials with modified carinata meal
(2018): Economic sensitivity analyses and producer surveys.
(0):: Seeding rate and timing recommendations.
(0):: Herbicide and insecticide recommendations.
(0):: Optimal carinata varieties for each ecoregion.
(0):): Effects on pollinator health.
(0):: Optimal pilot scale conditions for processing carinata meal.
(0):: Beef and dairy feeding results with raw carinata meal.
(0):: Rotational role and effects of carinata.
(0):: Updated economic and life cycle analyses
(0):: Complete final manuscripts for publication
Projected Participation
View Appendix E: ParticipationOutreach Plan
The proposed research will generate new information, outreach resources, and peer-reviewed publications. In addition to best management guides and agricultural articles, an ESRI on-line story map will feature an interactive and visual interface to carinata test and analysis results. Users will be able ‘play’ with the data and learn as they interact with the maps and graphics. The resources developed will be integrated into current university extension activities [e.g. iGrow (http://igrow.org/), EDIS (https://edis.ifas.ufl.edu/)]. A ‘road show’ presentation summarizing results will be developed and presented to local and state legislative representatives. Results and resources will also be presented at local conventions, meetings, and through highlights on radio and television (such as regional show “Today’s AG”). Field days will be hosted at test plots and at research farms throughout the research project, providing producers the opportunity to see and learn more about carinata. The proposed research will be integrated into collaborative learning opportunities for K-12 students through organizations (e.g. 4-H, Future Farmers of America) and university relationships and programs [e.g. summer camps; Girls, Engineering, Mathematics, and Science (GEMS); Gear-up]. The learning modules will focus on sustainable agriculture. Presentations will also be made by researchers to individual K-12 classrooms and assistance with developing rubrics and lessons to integrate non-food feedstocks, pollinator health, animal foods, agricultural economics, and environmental impacts into curriculum will be provided to interested teachers. It is the intent for these modules to foster the children’s interest in science and engineering so that they may eventually emerge as tomorrow’s scientific leaders.
Organization/Governance
The recommended Standard Governance for multistate research activities include the election of a Chair, a Chair-elect, and a Secretary. All officers are to be elected for at least two-year terms to provide continuity. Administrative guidance will be provided by an assigned Administrative Advisor and a CSREES Representative.
Literature Cited
1. U.S. Department of Energy. “State & Federal Incentives & Laws: All Incentives and Laws Sorted by Type,” Washington DC, 2012. Available at http://www.afdc.energy.gov/afdc/laws/matrix/incentive . [Accessed June 18, 2012] 2. Glover, B. and J. Heimlich, “Farm to Fly: Towards a Comprehensive Sustainable Aviation Biofuels Rural Development Plan.” 2009. Available at http://www.airlines.org/Energy/Alternativefuels/Documents/farmtoFly Presentation 071410.pdf. [Accessed June 20, 2011] 3. Lane, Jim, “Aviation and Military biofuels: new thinking on finance, fuels.” 2012, Available at http://www.biofuelsdigest.com/bdigest/2012/02/08/aviation-andmilitary-biofuels-new-thinking-on-finance-fuels/ [Accessed June 24, 2012] 4. U.S. Department of Agriculture, (USDA), “Agriculture and Aviation: Partners in Prosperity.” January 2012. Available at http://www.usda.gov/documents/usdafarm-to-fly-report-jan-2012.pdf. [Accessed June 24, 2012] 5. Nelson, Robert J., and Kevin D. Kephart, “Feedstock Development in North Central Region for Green Diesel and Jet Fuels,” May 2011. Available at http://ncsungrant.sdstate.org/upload/North-Central-Region-Oilseed-White-Paper060811-2.pdf. [Accessed June 18, 2012] 6. Biofuels Digest, “Military signals advanced biofuel demand: 336 million gallons per year by 2020.” 2010. Available at http://www.biofuelsdigest.com/bdigest/2010/12/16/military-advanced-biofuelsdemand-336-million-gallons-by-2020-voices-from-the-bio-pacific-rim-summit/. [Accessed June 23, 2012] 7. Stein, Luke, “Economic Analysis of Potential Camelina Oil Crop Supplies in the Northwest US.” Selected paper presented at Western Agricultural Economics Association, 2012 Annual Meetings, Park City, Utah, June 20-22, 2012. 8. Wang, Wei-Cheng and Ling Tao, “Bio-jet fuel conversion technologies.” Renewable and Sustainable Energy Reviews, 53, p 801-822, 2016. Available at: http://www.sciencedirect.com/science/article/pii/S1364032115009867 9. Xin, H. and P. Yu, “Chemical profile, energy values, and protein molecular structure characteristics of biofuel/bio-oil co-products (carinata meal) in comparison with canola meal.” Journal of Agricultural Food Chemistry, 61 (16) p 3926-33, 2013. Available at: http://pubs.acs.org/doi/abs/10.1021/jf400028n 10. Potter, Mark J., Kerrie Davies, and Anthony J. Rathjen, “Suppressive impact of glucosinolates in Brassica vegetative tissues on root lesion nematode pratylenchus neglectus.” Journal of Chemistry Ecology, 24 (1) p 67-80, 1998. Available at: http://link.springer.com/article/10.1023%2FA%3A1022336812240 11. Osler, Graham H.R., Petra C.J. van Vliet, Craig S. Gauci, and Lynette K. Abbott, “Changes in free living soil nematode and micro-anthropod communities under a canola-wheat-lupin rotation in Western Australia.” Journal of Soil Research, 38 (1) p 47-60, 2000. Available at: http://www.publish.csiro.au/paper/SR99050 12. Jain, S.M. and S.D. Gupta (ed.), “Biotechnology of neglected and underutilized crops.” Springer Science and Business Media, p 459, 2013. 13. Xin, Hangshu and Peiqiang Yu, “Rumen degradation, intestinal and total digestion characteristics and metabolizable protein supply of carinata meal (a non-conventional feed resource) in comparison with canola meal.” Animal Feed Science and Technology, 191 p 106-110, 2014. Available at: http://www.animalfeedscience.com/article/S0377-8401%2814%2900041-8/pdf 14. Sackey, A., E.E. Grings, D.W. Brake, and K. Muthukumarappan, “Effect of processing conditions on nutrient disappearance of cold-pressed and hexane-extracted camelina and carinata meals in vitro.” iGrow Beef Reports, p 5, 2015. Available at: https://www.sdstate.edu/ars/species/beef/beef-reports/upload/05-Adolf-oilseed-processing-04_21_2015-pm.pdf 15. Rajapaske, Banuka Y., “Nutritive evaluation of mechanically-pressed camelina (Camelina sativa), carinata (Brassica carinata) and soybean (Glycine max) meals for broiler chickens.” M.S. Thesis, Dalhousie University, Halifax, Nova Scotia, p 246, April 2015. Available at: http://dalspace.library.dal.ca/xmlui/bitstream/handle/10222/56764/ Rajapakse-Banuka-MSc-AGRI-April-2015.pdf?sequence=3&isAllowed=y 16. Tripathi, M.K. and A.S. Mishra, “Glucosinolates in animal nutrition: A review.” Animal Feed Science and Technology, 132 (1-2) p 1-27, 2007. Available at: http://www.sciencedirect.com/science/article/pii/S0377840106001362 17. FAO, “Fish to 2030: Prospects for fisheries and aquaculture.” World Bank Report Number 83177-GLB, p 102, 2013. Available at: http://www.fao.org /docrep/019/i3640e/i3640e.pdf