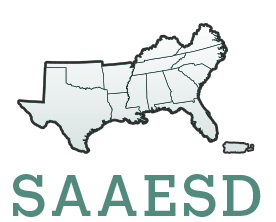
S1066: Development of sustainable crop production practices for integrated management of plant-pathogenic nematodes
(Multistate Research Project)
Status: Inactive/Terminating
S1066: Development of sustainable crop production practices for integrated management of plant-pathogenic nematodes
Duration: 10/01/2015 to 09/30/2020
Administrative Advisor(s):
NIFA Reps:
Non-Technical Summary
Statement of Issues and Justification
Plant-parasitic nematodes significantly reduce the yield and quality of a wide range of crops, world-wide. Although recently quantified descriptions of these losses are difficult to obtain, average yield losses to nematode parasitism in the world’s major crops has been estimated to average 10-14% annually (Sasser and Freckman 1987). Annual crop losses in the US have been estimated to range from minimal for some commodities in some regions to an approximate maximum of 15% in others (Koenning et al, 1999). The research in this proposal addresses important agricultural crop management issues as identified by the REE Advisory Board. In the Report on Agriculture Research Needs of Commodity Crops, the REE Advisory Board states that sustainable, efficient production of these staples (including cotton, soybean, and peanut) is critical to the US food supply and economy, and that “productive efficiency” must be a top priority in order to meet the demands of a growing population in the US and the world. They further list as top priorities, nematode resistance in cotton and improved varieties and disease resistance in peanut. Many farmers have relied on fumigant and non-fumigant pesticides to control plant-parasitic nematodes, but most of the non-fumigant nematicides are no longer available, and application of most soil fumigants now involves significant additional application restrictions and requirements. If the research in this proposal is not conducted, growers will experience greater production costs and yield losses. Nematode-resistant crops can lower production costs by reducing nematicide applications, while at the same time improve yield. Reduction in nematicide use will also reduce risks of environmental contamination and potential adverse effects on public health. Host resistance to nematodes and other plant pests and pathogens is often cited as the preferred method of pest management (Cook and Starr, 2006), but some parasitic nematodes are able to adapt quickly to resistant cultivars and overcome the resistance (Vuong et al., 2013; Jones et al., 2013), and incorporating nematode resistance into superior genetic backgrounds for yield and quality attributes can be difficult (Starr et al., 2002). Nematode-resistant germplasm has been deployed in various crop plants (Concibido et al., 2004; Brucker et al., 2005; Kim et al., 2011; Starr et al., 2002), but with the narrow genetic basis for this resistance, new sources of host resistance are urgently needed. Nematode resistance is also almost always limited to a single nematode species, and is even sometimes limited to one or more specific “host races”. Crop production in the South frequently involves fields infested with multiple economically important plant-parasitic nematode biotypes that can adapt to nematode management practices, including crop rotations and host resistance (Starr et al., 2002). For these reasons, resistance should be integrated into nematode management systems that include other control tactics in order to successfully manage the entire community of plant parasitic nematodes in commercial fields. These other tactics could include cropping systems (crop rotation and/or use of cover crops) and nematode management agents (NMA), which can include synthetic pesticides as well as biocontrol agents. Crop rotation, cover crops and soil amendments have been increasingly studied for their ability to suppress nematode population densities (Lu, et al., 2005), and considerable research is also being conducted on biofumigation and other methods to produce compounds that reduce preplant nematode populations (Zasada et al, 2010). Compounds applied to control other soilborne pathogens have been found, more recently, to also possess significant and useful nematicidal activity (Faske and Hurd, 2014). There have also been significant advances in the detection, identification, and use of biological agents that suppress nematodes (Timper, 2011). However, there has been very little research on integrating these other practices, particularly cultural and biological practices, with host-plant resistance. Thus, there is a need to evaluate the combined use of host resistance with cover crops, commercially available biological control agents, and other new NMAs, to control plant-parasitic nematodes. Through this proposed regional project, there will be on-going scientist to scientist integration of research and outreach to accelerate progress. There are common crops that are vulnerable across states with programs that are locally specific; by sharing information on a timely basis what is learned at one location can have a significant effect on programs in other locations. The proposed project provides the formal relationship to facilitate multistate cooperative efforts with the annual meeting providing the incentive.
Related, Current and Previous Work
There are currently three other multistate research projects that focus on plant parasitic nematodes: NC1197, NE1040, and W3186. These multistate projects differ in the crops of primary interest, the specific nematode species investigated, and the research approaches used and/or specific goals sought. The research proposed here is unique in its primary interest on cotton, peanut, corn, and soybean, and its focus on host resistance in one of more of these crops to M. incognita, Rotylenchulus reniformis, and Heterodera glycines. For instance, R. reniformis is the primary economic pathogen in cotton production in the southeastern United States, reducing the annual value of cotton production in Louisiana, Mississippi and Alabama by an estimated 5% (Blasingame et al, 2010). Because host resistance is so specific to nematode taxa, the proposed research will also work on Hoplolaimus spp. on a variety of crops grown in the South, other species of Meloidogyne that may occur in this region (such as M. arenaria, M. enterolobii, M. javanica, M. naasi, M. partityla) and Pratylenchus zeae. The plant-parasitic nematodes and crops, cultivars and practices in this proposal are of major importance in the southern US versus other regions of the country. NC1197 focuses strongly on nematode management for soybean and corn as major crops in the north-central US, with some research on managing potato nematodes, and with a particularly strong concentration on soybean cyst nematode (SCN – Heterodera glycines) and root lesion nematode (RLN- Pratylenchus penetrans). NE1040 concentrates on identifying improved management tactics (especially cultural practices) for the root-knot nematode species Meloidogyne hapla and M. incognita on vegetable crops, strawberry, and in “rotational grain crops”, and seeks a broad perspective by including concepts of “sustainable soil health” in its methods and goals. Research for W3186 investigates variability and adaptation of plant-parasitic nematode populations, as well as management, for a wide variety of nematode parasites (Globodera ellingtonae, Heterodera avenae, H. cruciferae, H. glycines, H. schachtii, M, chitwoodi, M. hapla, M. incognita, Pratylenchus spp.) on a very diverse set of annual and perennial crops (including cotton and soybean, sugar beet, potato, and small grains, but also blueberry, raspberry, turf, pecans, and even pineapple). Our primary focus on host plant resistance, particularly in objectives 1 and 2, is of less importance in the other multistate research projects, except for the focus on SCN resistance in NC1197. Our Objective 1 (advancing species identification and analysis) focuses on use of multiple research approaches to nematode taxonomy, in contrast to studies in the W-3186 project that focus more tightly on use of molecular techniques on just a few species of cyst and root-knot nematodes. The starting point for any effective management plan for plant-parasitic nematodes is accurate identification of the key pests. The efficacy of biological control and host plant resistance, especially, can be highly species-specific. Species of concern in field crop and vegetable production in the southeast, and the focus of this project, include: Meloidogyne spp., Hoplolaimus spp., Rotylenchulus reniformis, Heterodera glycines, and other cyst species. Identification of species as widespread and intensely studied as M. arenaria, M. incognita, and M. javanica is still challenging (Perry et al., 2009); several populations elude identification even by experts. Numerous populations have been misidentified by trying to make them “fit” as one of the three most common species (i.e. M. enterolobii, M. floridensis, M. naasi, among others). Meloidogyne species that do not reproduce on tomato, the “universal host”, are often overlooked and understudied. The identification problem is compounded by the presence of mixed species and the occurrence of physiological variants (host races). With the advent of phylogenetic approaches to species delimitation, it has become necessary to take a closer look at the status of currently described morphospecies. High genetic variability within species is common in plant-parasitic nematodes and makes it difficult to differentiate between extensive population subdivisions and species. Characterizing intraspecific variability is necessary for the development of consistently reliable or “durable” plant host resistance. This variability should be characterized both genetically and phenotypically (morphology, reproduction, isozyme profiles) and has important implications on the selection of populations used for resistance screening. A key feature of plant parasitism by cyst and root-knot nematodes is the injection of effector proteins into host root cells through their stylets. Stylet secretions manipulate a wide range of cellular processes, and knowledge of these nematode effector repertoires is expanding (Maier et al., 2013). Recent research has revealed some of the fundamental roles of effectors in nematode infection (Hewezi et al., 2013) and global gene expression studies has provided sequence information on various gene functional categories that can be targeted for generating host resistance (reviewed by Escobar et al., 2011). Several genes involved in biosynthesis of multiple secondary metabolites constitute a significant portion of the nematode-regulated genes. Secondary metabolites frequently play an important role in plant immunity by controlling the entry and/or development of the pathogens into plant cells and tissues (Bednarek, 2012). Thus, manipulating the biosynthesis of secondary metabolites may provide novel solutions for controlling plant-parasitic nematodes. Transcriptome analysis alone, however, is insufficient for assigning potential roles to the genes differentially expressed in nematode parasitism. Functional characterization of these genes by overexpression and RNAi is required to elucidate their functional role in parasitism. Individually assaying hundreds to thousands of differentially expressed genes without any selection criteria would be extremely difficult and labor-intensive, but co-expression analyses provide a means to select and prioritize genes of interest for detailed function analysis. Genes showing similar expression patterns under diverse conditions often have similar or associated functions. The biological function of genes can be predicted based on co-expression networks (Usadel et al, 2009). Furthermore, gene co-expression networks will provide a system view of how host plants employ a genome-wide response to nematode infection, and hence main hubs with high connectivity relationships can be selected for in-depth functional analysis. Genome-wide expression profiling studies also provided convincing evidence that gene silencing might be essential for successful parasitism by cyst and root-knot nematodes (reviewed by Hewezi and Baum 2014). Comparing gene expression changes between susceptible and resistant interactions between soybean and Heterodera glycines revealed that gene up-regulation was associated with the resistant interactions, while gene down-regulation was associated with the susceptible interactions (Klink et al., 2007 and 2009; Kandoth et al, 2011). Recent studies point to key roles of small RNA pathways in controlling gene expression of nematode-infected host cells, both at transcriptional and post-transcriptional levels (Hewezi et al., 2008; Hewezi et al., 2012). Understanding how parasitic nematodes exploit small RNA pathways to manipulate host gene expression to mediate susceptibility may provide opportunities to interfere with this regulation and hence render host plants resistant. Crop rotation with a resistant or non-host crop can be an effective management tactic, but resistance is not commercially available in all crops, and a non-host crop may not be a good fit in some production systems. There are numerous examples of cover crops that are antagonistic, resistant, or suppressive to nematode population development (Lu et al., 2005), and several species of Brassica have long been recognized for their suppression of several plant-parasitic nematodes (Matthiessen and Kirkegaard, 2006). A key component is the release of isothiocyanate, generated from the breakdown of glucosinolates when Brassica spp. cover crops are incorporated into the soil (Zasada et al., 2004, 2009, 2010). Although this technique has been effective on many species of plant-parasitic nematodes, there has been some inconsistency in nematode suppression. In some studies this was related to the level of glucosinolates produced by specific species of Brassica, or stages of plant growth when incorporated, whereas in other studies Brassica spp. were a suitable host for RKN (McLeod, 2001; Oka, 2010). Allyl isothiocyanate, which is chemically related to isothiocyanate produced by the breakdown of glucosinolates, recently became commercially available (Dominus® - http://www.businesswire.com/news/home/20130930006408/en/Isagro-USA-Announces-U.S.-EPA-Registration-DOMINUS%C2%AE#.VHorr010zIU). This product may provide more consistent release of isothiocyanate without the risk of cover crop being a suitable nematode host. However, little is known about the compatibility of isothiocyanate or allyl isothiocyanate with biological agents and other cultural practices to manage plant-parasitic nematodes. There are also numerous examples of soil amendments that produce nematicidal compounds during degradation, whereas other amendments may enhance antagonistic microbes (Lu et al., 2005: Oka, 2010), but few studies have investigated the combined effects of crop rotation (resistant and non-host), cover crops, soil amendments and biological agents (Timper, 2014). A contributing factor in these limited studies was likely the lack of a commercially available biological agent, but commercialization of biological agents has improved, providing an opportunity to evaluate some biological agents with cultural tactics in large scale studies for suppression of plant-parasitic nematodes. One desirable characteristic of a nematode management agent (NMA) is to have more than one function in disease or pest management. PCNB was one of the first fungicides recognized to inhibit M. incognita motility in-vitro (Adams et al. 1979), and thiophanate-methyl was one of many benzimidazole fungicides exhibiting some toxicity to plant-parasitic nematodes (Faghihi et al., 2007; Krishna Prasad et al. 1980). In both of these earlier studies fewer nematodes were recovered from field plots treated with either fungicide (highest rate) compared to the non-treated control, but no consistent yield benefit. Recently, fluopyram was reported to be toxic to both M. incognita and R. reniformis, which is unique among SDHI fungicides (Faske and Hurd, 2014). Fluopyram is being evaluated as a seed treatment and for in-furrow application in soybean, corn, and cotton fields for its effects on fungal disease and plant-parasitic nematodes. Given advances in biological control (Timper, 2011) and soil amendments (Oka, 2010), strategies that integrate biological control with other control measures need investigation. Registration of Pasteuria nishizawae Pn1 as a seed treatment (Clariva®) for SCN provides an opportunity for scientists to conduct large scale field trials to evaluate a biological control agent on SCN, where race shifting has been a challenge with continued use of host plant resistance (Koenning and Wrather, 2010; Niblack, et al. 2008). Bacillus spp. also interfere with nematode behavior to reduce infection in vegetable and some field crops (Viaene et al. 2013). Bacillus subtilis GBO3 and B. cereus C4 suppressed Meloidogyne sp. root galling on pepper (Kokalis-Burelle et al 2002). Bacillus firmus 126 suppressed M. incognita on tomato by 54-65% (Terefe et al., 2009), and R. reniformis by 70% in cotton field trials (Castillo et al., 2013). Nematophagous fungi are the most common organisms in nematode suppressive soils, effective against eggs and females of sedentary nematodes (Timper, 2011). Of these fungi, Paecilomyces lilacinus is one of the most widely tested, and P. lilacinus 251 is commercially available in agronomic (NemOut®) and horticultural crops (MeloCon®). The organism was somewhat effective on turf against M. marylandi and Belonolaimus longicaudatus, but did not provide season long control as a stand-alone application (Crow, 2013; Starr et al., 2007). Paecilomyces lilacinus 251 reduced galling of tomato by M. incognita by 66% to 70% (Kiewnick 2009; Lara Martez et al. 1996). In tomato microplot trials, P. lilacinus reduced total mid-season vermiform reniform counts by 59% at mid-season when applied 2-wk before nematode infestation. However, application timing is often critical, and multiple applications are often needed when used as a stand-alone management tactic. Three applications of P. lilacinus (7 d pre, at, and 7 d post planting) in a cotton field trial reduced RN vermiform in soil by 59% and 70% at 60 and 90 days after planting, respectively (Castillo et al., 2013). Alternately, the fungus applied at transplanting and 2 weeks after transplanting did not reduce RN numbers on tomato (Lara Martez et al. 1996). Other fungi (i.e. Pochonia chlamydosporaia, Myrothecium verucaria AARC-0255, Trichoderma spp., etc.) have also been detected in suppressive soils and some are available in commercial products for large scale tests or are relatively easy to culture on grain products (Hallmann et al., 2009). Combining these biological agents with host plant resistance, crop rotation and/or cover crops, and other NMAs could provide satisfactory nematode control in a sustainable system.
Objectives
-
Advance the tools for identification of nematode species and characterization of intraspecific variability
-
Elucidate molecular and physiological mechanisms of plant-nematode interactions to improve host resistance
-
Integrate nematode management agents (NMAs) and cultural tactics with the use of resistant cultivars to develop sustainable crop production systems.
Methods
1. Advance the tools for identification of nematode species and characterization of intraspecific variability. The populations and species to be identified will come from individual field collection efforts, existing cultures in different labs, and collaborations with nematologists in this project and elsewhere. Planned surveys will be conducted in Virginia and South Carolina, in which soil samples will be collected from corn, soybean, cotton, and peanut fields arbitrarily selected but representative of the row crop growing areas in the two states. Host races of M. incognita and M. arenaria will be identified using North Carolina Differential Host Test protocols (Taylor and Sasser, 1978). The correct identification of species will form the basis for many of the activities carried out in Objectives 2 and 3, where the identity of populations used in the experiments described for the other two project objectives will be confirmed by light and scanning electron microscopy, isozyme profiling and PCR-based methods. Detailed morphological and morphometric studies will be undertaken with selected populations to detect population mixtures and species either undescribed or not reported in the southeastern United States. Esterases and malate dehydrogenases will be profiled to supplement morphological observations for Meloidogyne species and to investigate correlations with morphology and other phenotypic traits. Nuclear and mitochondrial genes will also be sequenced for populations characterized morphologically. Phylogenetic approaches and population structure analyses will be used to delimit species and correlations between phylogenetic lineages and morphospecies will be examined. Phenotypic variation will be correlated with measures of genetic diversity which may be shaped by plant host factors. Key aspects of the project will be the use of common protocols, rapid data exchange, and coordinated effort to reduce redundancy while ensuring key discoveries are rigorously tested in multiple locations. 2. Elucidate molecular and physiological mechanisms of plant-nematode interactions to improve host resistance. Whole individual esophageal gland cells will be isolated from both cyst (Heterodera glycines) and root-knot (Meloidogyne spp.) nematodes (Maier et al., 2013) and used for mRNA purification and high-throughput sequencing. Sequence reads and putative effector gene candidates will be identified as described in Rutter et al. (2014). An integrated approach of genetic, molecular, and biochemical methods will be used to functionally characterize effector gene candidates. Because RNA-Seq technology can quantify gene expression changes at genome levels and identify alternative gene spliced transcripts, post-transcriptional modifications and mutations/SNPs among various lines and cultivars (Ozsolak and Milos, 2011), RNA-Seq will be used to quantify gene expression changes associated with nematode infection and with specific genes or lines. The differentially expressed genes of syncytium and giant-cells will be clustered into groups of closely-connected modules based on their co-expression under various sets of experimental conditions to generate co-expression networks. The main networks will be further subdivided into subnetworks or modules. Main nodes or hubs will be considered for further studies by reverse genetic methods to decipher the mechanisms by which these genes control plant response to nematode infection. Because thousands of genes are known to be involved in plant response to nematode infection, rapid screening strategies are critical for elucidating the contributions of individual genes in the interaction of host plants and parasitic nematodes. Transgenic hairy roots and virus-induced gene silencing (VIGS) are becoming increasingly efficient methods to conduct large-scale, high-throughput genomic studies in plants (Kereszt et al., 2007; Purkayastha and Dasgupta, 2009). Agrobacterium rhizogenes-mediated root transformation results in generation of composite plants containing transgenic hairy roots developed on non-transformed shoots (Kereszt et al., 2007). The transgenic hairy root system is a well-adapted tool to investigate interactions between host plants and parasitic nematodes because nematodes can complete their entire life cycle in this system. We will use transgenic hairy roots to manipulate the expression of potential host resistance genes to M. incognita using overexpression, artificial microRNA or RNAi constructs. VIGS technology uses modified viruses to introduce small nucleotide sequences into the plant to silence the expression of a particular host gene. When the plants degrade the RNA of the virus to defend themselves, they also degrade the endogenous RNA of the target gene, “silencing” the gene. Transgenic hairy roots and VIGS are both efficient systems to evaluate the direct involvement of host genes in plant resistance to nematodes, and offer an efficient alternative to high-throughput screening without the requirement to generate stably transgenic plants. Host genes preferentially expressed in H. glycines and Meloidogyne spp. feeding sites will be screened by cloning promoters of the candidate genes, driving expression of a reporter gene such as GUS, GFP or RFP, using the hairy root overexpression system or stable transformation model systems. The inducibility of the promoters will be assayed at various time points post-nematode inoculation. An integrative strategy will also be employed for functional analysis of genes involved in secondary metabolite biosynthesis pathways. Key candidate genes for secondary metabolite biosynthesis will be identified using various Omics tools and bioinformatics. The biochemical function of these genes will be determined using in vitro biochemistry, and their biological function will be determined using the reverse genetic approach described above and bioassays. 3. Integrate nematode management agents and cultural tactics with the use of resistant and/or tolerant cultivars to develop sustainable crop production systems. The influence of plant genotype on nematode parasitism and reproduction will be compared each year among advanced breeding lines and new versus standard cultivars on a range of crops grown in the Southern region of the US. In order to monitor interspecific variation in relation to nematode resistance genes, potential changes in populations of non-target nematode genera will also be evaluated in addition to resistance effects on the population dynamics of the target nematode(s) in each trial. We will also evaluate the impact of current crop rotation sequences, potential new winter cover and/or rotation crops, and alternating resistant versus susceptible cultivars on the annual population dynamics of plant parasitic nematodes in the Southern region. “Cropping systems” tend to change based more on commodity market forces than pest control potential, but the impacts of these changes in terms of management of plant-parasitic nematodes are poorly understood. Corn, soybean, cotton and peanuts are widely grown in sequence in the Southern United States. Nematode population development in these sequences will be monitored in participating states by annually collecting soil samples from each crop from multiple GPS-designated sites within fields. Soil samples will also be collected near harvest for nematode assays from a soybean, corn, wheat, and sorghum rotational study in a field infested with H. glycines. Samples will be collected over a three year period in order to monitor changes in nematode populations. Root-knot nematode population dynamics may also be monitored in a soybean:corn rotation, and possibly also a peanut/cotton rotation. Nematode populations will be monitored at multiple sites in each participating state, and then compared across regions and crops. Nematode population development and crop productivity associated with potential new nematode management agents (NMA) will be compared in multiple field trials across the South. A NMA would be any product marketed for its ability to suppress population development of a plant parasitic nematode, and would include, but not be limited to, both experimental and commercially available biocontrol agents and other products. Each year, field trials with comparable NMAs will be evaluated across states for cotton, soybean and grain crops. For example, members of the committee will continue active participation in the Beltwide Cotton Nematode Research and Education Committee, which annually conducts field research in 10 Southern states to evaluate cotton cultivar and NMA combinations in diverse production systems. Common treatment and data collection protocols are used in order to analyze annual results across locations (as many as 11 in 2014). Each location utilizes a randomized complete block experimental design (RCBD) with four to five replications. Root-knot and/or reniform nematode populations will be estimated at planting, 30-60 days after planting (DAP), and at harvest. Cotton stand counts and vigor ratings are also made 30-60 DAP, and seed cotton yield data are collected. Two soybean NMA trials will also be conducted each year, each typically also arranged in a RCBD with four to five replications. Two to three greenhouse experiments are also planned to more fully characterize the nematicidal activity of fluopyram applied using various methods. An IPM approach with both NMA and cropping system approaches will likely prove to be more effective than either approach alone to manage plant-parasitic nematodes. The same set of specific NMAs will be compared annually for activity against root-knot and/or reniform nematodes on cotton and soybean cultivars resistant and/or tolerant to these plant parasites. For all these trials, crop performance as well as nematode population dynamics will be monitored at each site and appropriate statistical evaluation used to determine the relationship between nematode population density and crop response.Measurement of Progress and Results
Outputs
- New characters for more accurate species delimitation; improved detection of mixed populations; better knowledge of the geographic distribution of economically important plant pathogens of Meloidogyne, Hoplolaimus, Rotylenchulus, and Heterodera.
- Identification of host genes uniquely responsive to nematode infection to expedite development of more durable resistance. Identification and functional characterization of nematode effector genes, to facilitate discovery of the molecular mechanisms of nematode parasitism. Characterization of nematode-regulated gene function and improved knowledge of gene co-expression networks.
- Research results on how crop cultivars, rotation sequences, cover crops, and NMAs may fit best or be adapted to improve profitability and sustainability of cropping systems among southern states.
Outcomes or Projected Impacts
- Nematode management will improve because nematode-resistant cultivars, NMAs, and cultural tactics will be based on more accurate characterization of the nematode species and races present and causing damage.
- New species of plant-parasitic nematodes will be described
- Enhanced plant resistance to parasitic nematodes is expected to ultimately result from o integrated pathways developed based on analysis of gene co-expression networks, and o discovery of the molecular mechanisms by which parasitic nematodes infect host plants and cause disease, facilitated by the identification and functional characterization of nematode effector genes.
- More durable resistance should also ultimately result from: o identification of host genes uniquely responsive to nematode infection, and o introgression of novel sources of nematode resistance identified through functional characterization of nematode-regulated host genes.
- New nematode-resistant cultivars should be available for a range of crops based on: o Annual field comparisons of advanced breeding lines and new cultivars with current standards, and o Private sector seed companies releasing cultivars that incorporate the new sources of genetic resistance identified in this project
- New cropping systems identified through this project should better integrate host plant resistance with cropping sequences and nematode management agents for practical use in crop production systems.
- Decreased crop yield losses and reduced reliance on pesticides should result from increased availability of nematode-resistant crop cultivars and from practical and profitable crop production systems that reduce nematode population densities.
Milestones
(2016): • Obj#1- Describe unnamed Meloidogyne and Hoplolaimus populations obtained from previous survey work or extension nematode assays in South Carolina and Virginia. • Obj#2- Identify key components of nematode virulence and host resistance. • Obj#3- Establish small plot field trials comparing NMAs and new nematode resistant genotypes. Identify fields and begin collecting samples for monitoring nematode population dynamics in cropping systems project.(2017): • Obj#1- Characterize the known distribution of Meloidogyne and Hoplolaimus species in selected states. • Obj#2- Initiate the functional studies of nematode effector genes and host resistance factors (Gene expression analysis, co-expression networks and generate overexpression and silencing constructs). • Obj#3- Repeat and expand small plot trials and continue nematode population monitoring in cropping system project outlined for 2016. Annual results will be extended to stakeholders through multi-media approach.
(2018): • Obj#1- Re-describe root-knot and lance nematode species that were described inadequately according to current standards, and begin describing new species, morphospecies, variants, or races conducted in Virginia and South Carolina. • Obj#2- Continue the functional studies (generate transgenic lines and perform nematode susceptibility assays). • Obj#3- Repeat and expand small plot trials and continue nematode population monitoring in cropping system project outlined for 2016. Annual results will be extended to stakeholders through a multi-media approach
(2019): • Obj#1- Finish describing new species, morphospecies, variants, or races found during surveys conducted in Virginia and South Carolina. Re-describe root-knot and lance nematode species that were described inadequately according to current standards. Begin preparing a monograph on the root-knot nematode species. • Obj#2- Complete the functional studies and begin data analysis • Obj#3- analyze and compare nematode population data over the three-year cropping sequence, across participating states. Annual results will be extended to stakeholders through a multi-media approach. Begin data analysis and organization across participating states.
(2020): • Obj#1- Produce publications that improve species delimitation of lance and root-knot nematode species. Publish a monograph of the root-knot nematode species. • Obj#2- Complete data analysis and disseminate information through peer-reviewed publications. • Obj#3-Complete data analysis and evaluate results for publication in appropriate journals and venues. Dissemination of results to the public as outlined in the Outreach plan
Projected Participation
View Appendix E: ParticipationOutreach Plan
Field experiments will be conducted with the collaboration of county extension personnel and cooperating growers. Results from these applied research trials will be distributed to clientele through a variety of venues, such as county agent meetings, production meetings, extension web sites, blogs, newsletters or newspapers, production guides, and the popular press. Initial reports on efficacy of various NMAs and cropping systems will also be published in more applied, peer reviewed journals such as Plant Disease Management Reports, and Plant Health Progress. Such publications are typically preceded by presentation of preliminary data at meetings of commodity groups (Beltwide Cotton Conferences, Southern Soybean Disease Workers) and meetings of the American Phytopathological Society and Society of Nematologists. Additionally, project personnel will make regular reports on research findings to commodity leadership groups within each state. More basic aspects of this research (i.e., resistance/parasitism gene discovery and characterization) will be published in refereed journals such as the Journal of Nematology, Nematology, Phytopathology, Molecular Plant-Microbe Interactions, Plant Molecular Biology, and PLoS ONE.
Organization/Governance
Activities of the project will be coordinated by elected officers serving as Chair, Vice-Chair, and Secretary. Each officer will serve a two year term, with the Vice-Chair automatically moving up to the position of Chair after two years. Additionally, an Objective Coordinator will be appointed for each of the three principle objectives of the project, they will be responsible for summarizing research results for the objective for which they are responsible. Administrative guidance will be provided by an assigned Administrative Advisor and a NIFA Representative. Project members will meet annually to review research progress and to plan and coordinate future efforts. Additionally, annual meetings will be used to review and revise as needed common protocols for screening plant germplasm and nematode populations for virulence. The use of such common protocols will be essential for eventual publication of these data. Many decisions on exchange of germplasm will also be made at the annual meetings. Lastly a project web site will be established to facilitate communication and interim results among the participants
Literature Cited
Adams, J. R., Rodriguez-Kabana, R., and King, P. S. 1979. The non-target effects of pentachloronitrobenzene on plant parasitic and free-living nematodes. Nematropica 9:110-122. Bednarek, P. 2012. Chemical warfare or modulators of defence responses - the function of secondary metabolites in plant immunity. Current Opinion in Plant Biology 15:407–414. Blasingame, D., Patel, M. V., Lawrence, K., Gazaway, W., Olsen, M., Kirkpatrick, T.. Monfort, S., Davis, M., Marios, J., Kemerait, B., Colyer, P., Scuimbato, G., Lawrence, G., Wrather, A., Goldberg, N., Koenning, S., Pitts, J. T., Muller, J., Newman, M., Woodward, J., Wheeler, T., and Phipps, P. 2010. 2009 Disease loss estimate committee report, Proc. Belt-wide Cotton Conf., Vol. 1:238-240. National Cotton Council of America. Memphis, TN. Brucker E., Niblack T., Kopisch-Obuch, F. J., Diers, B. W. 2005. The effect of rhg1 on reproduction of Heterodera glycines in the field and greenhouse and associated effects on agronomic traits. Crop Science 45:1721–1727. Castillo, J. D., Lawrence, K. S., and Kloepper, J. W. 2013. Biocontrol of the reniform nematode by Bacillus firmus GB-126 and Paecilomyces lilacinus 251 on cotton. Plant Disease 97:967-976. Concibido, V. C., Diers, B. W., and Arelli, P. R. 2004. A decade of QTL mapping for cyst nematode resistance in soybean. Crop Science 44:1121–1131. Cook, R., and Starr, J. L. 2006. Resistant cultivars. Pp. 370-391 in Plant Nematology, R. N. Perry and M. Moens, eds. CABI Publishing, Wallingford, UK. Crow, W. T. 2013. Effects of a commercial formulation of Paecilomyces lilacinus strain 251 on over-seeded bermudagrass infested with Belonolaimus longicaudatus. Journal of Nematology 45:223-227. Escobar, C., Brown, S., Mitchum, M. G. (2011) Transcriptomic and proteomic analysis of plant response to nematode infection. In: Gheysen, G., Fenoll, C., Jones, J. T., eds. Genomics and molecular genetics of plant–nematode interactions. London, UK: Springer Science & Business Media, B.V., 157–175. Faghihi, J., Vierling, R. A., Santini, J. B., and Ferris, V. R. 2007. Effects of selected fungicides on development of soybean cyst nematode. Nematropica 37:25-265. Faske, T. R. and Hurd, K. 2014. Sensitivity of Meloidogyne incognita and Rotylenchulus reniformis to fluopyram. Phytopathology 104:S2.4. Hallmann, J., Davies, K. G., and Sikora, R. 2009. Biological control using microbial pathogens, endophytes, and antagonists. Pp. 380-411 in R. N. Perry, M. Moens, and J. L. Starr eds. Root-knot nematodes. Cambridge, MS: CABI Press. Hewezi, T., Baum, T.J. 2013. Manipulation of plant cells by cyst and root-knot nematode effectors. Mol. Plant Microbe Interact. 26:9-16. Hewezi, T., and Baum, T.J. 2014. Gene silencing in nematode feeding sites. In: Plant nematode interactions. Carolina Escobar & Carmen Fenoll (Eds). Advances in Botanical Research Series, Elsevier. Hewezi, T., Howe, P., Maier, T. R. and Baum, T. J. 2008. Arabidopsis small RNAs and their targets during cyst nematode parasitism. Molecular Plant-Microbe Interactions. 21:1622-1634. Hewezi, T., Maier, T. R., Nettleton, D. and Baum, T. J. 2012. The Arabidopsis microRNA396-GRF1/GRF3 regulatory module acts as a developmental regulator in the reprogramming of root cells during cyst nematode infection. Plant Physiology 159:321-335. Jones, J. T., Haegeman A., Danchin, E. G., Gaur, H. S., Helder, J., Jones, M. G., Kikuchi, T., Manzanilla-Lopez, R., Palomares-Rius, J., Wesemae, W. M. L., Perry, R. N. 2013. Top 10 plant?parasitic nematodes in molecular plant pathology. Molecular Plant Pathology 149:946-961. Kandoth, P. K., Ithal, N., Recknor, J., Maier, T., Nettleton, D., Baum, T. J. and Mitchum, M. G. 2011. The Soybean Rhg1 locus for resistance to the soybean cyst nematode Heterodera glycines regulates the expression of a large number of stress- and defense-related genes in degenerating feeding cells. Plant Physiology 155:1960-1975. Kereszt, A., Li, D., Indrasumunar, A., Nguyen, C. D., Nontachaiyapoom, S., Kinkema, M., Gresshoff, P. M. 2007. Agrobacterium rhizogenes-mediated transformation of soybean to study root biology. Nature Protocols 2:948-952. Kiewnick, S. 2009. Understanding multitrophic interactions to facilitate successful biocontrol of plant parasitic nematodes with Paecilomyces lilacinus strain 251. IOBC/WPRS Bulletin 43:297-299. Kim, M., Hyten, D. L., Niblack, T. L., Diers, B. W. 2011. Stacking resistance alleles from wild and domestic soybean sources improves soybean cyst nematode resistance. Crop Science 51:934-943. Klink, V. P., Hosseini, P., Matsye, P., Alkharouf, N. W. and Matthews, B. F. 2009. A gene expression analysis of syncytia laser micro-dissected from the roots of the Glycine max (soybean) genotype PI 548402 (Peking) undergoing a resistant reaction after infection by Heterodera glycines (soybean cyst nematode). Plant Molecular Biology 71:525-567. Klink, V. P., Overall, C. C., Alkharouf, N. W., MacDonald, M. H. and Matthews, B. F. 2007. Laser capture micro-dissection (LCM) and comparative microarray expression analysis of syncytial cells isolated from incompatible and compatible soybean (Glycine max) roots infected by the soybean cyst nematode (Heterodera glycines). Planta 226:1389-1409. Koenning, S. R., Overstreet, C., Noling, J. W., Donald, P. A., Becker, J. O., and Fortnum, B.A. 1999. Survey of crop losses in response to phytoparasitic nematodes in the United States. Journal of Applied Nematology 31:587-618. Koenning, S. R., and Wrather, J. A. 2010. Suppression of soybean yield potential in the continental United States by plant diseases from 2006 to 2009. Online. Plant Health Progress doi:10.1094/PHP-2010-1122-01-RS. Kokalis-Burelle, N., Vavrina, C. S., Rosskopf, E. N., and Shelby, R. A. 2002. Field evaluation of plant growth-promoting Rhizobacteria amended transplant mixes and soil solarization for tomato and pepper production in Florida. Plant and Soil 238:257-266. Krishna Prasad, K. S. and Rao, Y. S. 1980. Relative toxicity of chemicals to infective larvae of rice root-knot nematode, Meloidogyne graminicola. Indian Journal of Nematology 10:216-224. Lara Martez, J., Acosta, N., Betancourt, C., Vicente, N., and Rodriguez, R. 1996. Biological control of Meloidogyne incognita in tomato in Puerto Rico. Nematropica 26:143–152. Lu, M., Sikora, R. A., and Bridge, J. 2005. Plant parasitic nematodes in subtropical and tropical agriculture. Cambridge, MS: CABI Press. Matthiessen, J. N., and J. A. Kierkegaard. 2006. Biofumigation and enhanced biodegradation: Opportunity and challenge in soilborne pest and disease management. Critical Reviews in Plant Science 25:235-265. Maier, T. R., Hewezi, T., Peng, J., and Baum, T. J. 2013. Isolation of whole esophageal gland cells from plant-parasitic nematodes for transcriptome analyses and effector identification. Mol. Plant-Microbe Interact. 13:31-35. McLeod, R. W., Kirkegaard, J. A., and Steele, C. C. 2001. Invasion, development, growth and egg-laying by Meloidogyne javanica in Brassicaceae crops. Nematology 3:463-472 Niblack, T. L., Colgrove, A. L., Colgrove, K., and Bond, J. P. 2008. Shift in virulence of soybean cyst nematode is associated with use of resistance from PI 88788. Online. Plant Health Progress doi:10.1094/PHP-2008-0118-01-RS. Oka, Y. 2010. Mechanisms of nematode suppression by organic soil amendments – A review. Applied Soil Ecology 44:101-115. Ozsolak, F., Milos, P. M. 2011. RNA sequencing: advances, challenges and opportunities. Nature Review Genetics 12:87-98. Perry, R. N., M. Moens, and Starr, J. L. 2009. Root-knot Nematodes. CABI. Wallingford, UK. Purkayastha, A. and Dasgupta, I. 2009. Virus-induced gene silencing: a versatile tool for discovery of gene functions in plants. Plant Physiology and Biochemistry 47:967-976. Rutter, W. B., Hewezi, T., Abubucker, S., Maier, T., Huang, G., Mitreva, M., Hussey, R., and Baum, T. 2014. Mining novel effector proteins from the esophageal gland cells of Meloidogyne incognita. Molecular Plant-Microbe Interactions 27:965-974. Sasser, J. N., and Freckman, D. W. 1987. A world perspective on nematology: the role of the society. Pp. 7-14 in J. A. Veech and D. W. Dickson, eds. Vistas on Nematology. Society of Nematologists. Simpson, C. E., Starr, J. L., Church, G. T., Burow, M. D., and Paterson, A. H. 2003. Registration of NemaTAM peanut. Crop Science 43:1561. Starr, J. L., Bridge, J., and Cook, R. 2002. Resistance to plant-parasitic nematodes: History, current use and future potential. Pp. 1-22 in J. L. Starr, R. Cook and J. Bridge, eds. Plant Resistance to Parasitic Nematodes, CABI Publishing, New York, New York. Starr, J. L., Ong, K. L., Huddleston, M., and Handoo, Z. A. 2007. Control of Meloidogyne marylandi on bermudagrass. Nematropica 37: 43-49. Starr, J. L., Koenning, S. R., Kirkpatrick, T. L., Robinson, A. R., Roberts, P. A., and Nichols, R. L. 2007. The future of nematode management in cotton. Journal of Nematology 39:283-294. Terefe, M., Tefera, T., and Sakhuja, P. K. 2009. Effect of a formulation of Bacillus firmus on root-knot nematode Meloidogyne incognita infestation and the growth of tomato plants in the greenhouse and nursery. Journal of Invertebrate Pathology 100:94-99. Timper, P. 2011. Utilization of biological control for managing plant-parasitic nematodes. Pp. 259-289 in K. Davies, and Y. Spiegel, eds. Biological control of plant-parasitic nematodes: Building coherence between microbial ecology and molecular mechanisms. London: Springer. Timper, P., Holbrook, C.C., and Anderson, W.F. 2003. Reproduction of Meloidogyne spp. on resistant peanut genotypes from three breeding programs. Journal of Nematology 35:417-421. Timper, P. 2014. Conserving and enhancing biological control of nematodes. Journal of Nematology 46:75-89. Usadel, B., Obayashi, T., Mutwil, M., Giorgi, F. M., Bassel, G. W., Tanimoto, M., Chow, A., Steinhauser, D., Persson, S., and Provart, N. J. 2009. Co-expression tools for plant biology: opportunities for hypothesis generation and caveats. Plant Cell & Environment 32:1633-1651. Viaene, D., Coyne, D. L., and Davies, K. G. 2013. Biological and Cultural Management. Pp. 383-410 in R. N. Perry and M. Moens eds. Plant Nematology. 2nd ed. Boston: CABI Press. Vuong, T. D., Jiao, Y., Shannon, J. G., Nguyen, H. T. 2013. Nematode Resistance in Soybean. Pp. 95-124 in R. Varshney and R. Tuberosa, eds. Translational Genomics for Crop Breeding: Biotic Stress 1. Wiley-Blackwell. Zasada, I. A. and Ferris, H. 2004. Nematode suppression with Brassicaceous amendments: application based on glucosinolates profiles. Soil Biology & Biochemistry 36:1017-20124. Zasada, I. A., Meyer, S. L. F., and Morra, M. J. 2009. Brassicaceous seed meals as soil amendments to suppress the plant-parasitic nematodes Praytlenchus penetrans and Meloidogyne incognita. Journal of Nematology 41:221-227. Zasada, I. A., Halbrendt, J. M., Kokalis-Burelle, N., LaMondia, J. A., McKenry, M. V., and Noling, J. W. 2010. Managing nematodes without methyl bromide. Annual Review of Phytopathology 48:311-328