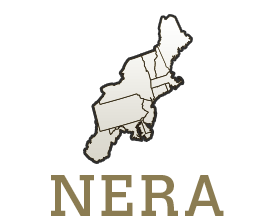
NE1710: Improving Forage and Bioenergy Crops for Better Adaptation, Resilience, and Flexibility
(Multistate Research Project)
Status: Inactive/Terminating
NE1710: Improving Forage and Bioenergy Crops for Better Adaptation, Resilience, and Flexibility
Duration: 10/01/2017 to 09/30/2022
Administrative Advisor(s):
NIFA Reps:
Non-Technical Summary
Statement of Issues and Justification
The economics of producing food, fiber, and energy products is a major issue in providing food security in the region and in all of North America. Forage crops are the foundation of livestock and dairy enterprises in the USA and Canada. Breeding of perennial forage crops has resulted in improved cultivars that make livestock, dairy, and energy production more economical by reducing inputs and increasing outputs. Compared to other types of crop species, perennial forage species enable more sustainable agricultural systems.
Leguminous forages reduce or eliminate N fertilization due to fixation of atmospheric N, thus reducing inputs and the risk of environmental contamination from fertilizer usage. Forage grass species have fibrous roots that reduce soil erosion and capture environmental contaminants. All of these perennial species reduce land disturbance that could lead to soil erosion. In addition, they sequester carbon and reduce greenhouse gas emissions.
Improved forage cultivars translate to benefits to agricultural producers of animal and energy products. The seed industry benefits from production and marketing of improved cultivars. All Americans benefit by reducing costs of food and energy and by protecting the environment by reducing use of pesticides, herbicides, and fertilizers.
Continuing the trend across the last few decades, the number of forage breeders in North America is decreasing. The number of forage researchers in the USA has decreased by 60% between 1984 and 2009. The number of extension workers declined by 30% (Rouquette et al., 2009). In some state experiment stations, as a forage scientist has retired or left their position for another, often they are not replaced. For example, in the last 15 years, forage breeding positions have been lost at Iowa State University, Oklahoma State University, and Kansas State University. The number of forage scientists at USDA-ARS also has declined. When the forage breeder at USDA-ARS in Mandan, ND, retired, his position was not replaced. At state agricultural experiment stations, alfalfa breeding research is being done in only three states and agronomic research in six states (Undersander, 2014). In FY 2012 USDA expenditures for alfalfa research were $3.7M among 9.9 scientist years, compared to more than $42M and more than 95 scientist years each for corn, cotton, and wheat (Samac, 2014).
The number of private breeding companies is few and have been reduced through consolidations. Currently, three main companies constitute the bulk of alfalfa breeding in the USA (Forage Genetics, Intl., S&W Seed Company, and Alforex). These companies work on few perennial forage species, mostly alfalfa with a smattering of grasses and clovers. Several major international forage seed companies focus on cool-season grasses and clovers, but most of that breeding is done outside the US. Many forage species of importance in North America are receiving no attention by private breeders.
As budgets and the number of scientists have been reduced, the need for cooperative research is more essential than ever. Most forage breeders work on more than one forage species, thus diluting efforts on individual species. Because these forage species are perennial, establishing fields is less frequent than with annual crops. Therefore, seed is sold less frequently per unit land area compared to that of annual crops. Unless forage cultivars are broadly adapted for use across a large range of environments, the seed industry is not interested in new cultivars because of the economic limitation. All of these factors point to the need for cooperative research to accomplish significant advances in developing improved forage cultivars adapted to a wide range of environments. Although forage scientists are few in number and individual efforts on some forage species are small, the cumulative efforts among forage scientists through cooperative research is significant. The current NE-1010 project fosters the interactions necessary to achieve goals with diminishing resources without unnecessary duplication.
This multistate cooperative research project addresses most of the NIFA priority focus areas, as explained below.
- Global food security and hunger: Breeding crops with higher forage yield, improved forage quality for livestock production, longevity, and resistance or tolerance to biotic and abiotic stress conditions will provide more economical food production.
- Climate change: Because of global warming, forage crops need to be developed that will be productive under abiotic stresses, including drought, flooding, cold and warm temperatures, and soil salinity.
- Sustainable bioenergy: Several of the researchers in the current NE-1010 multistate research project have been working on various grass and legumes species (for example, switchgrass, big bluestem, and alfalfa) for bioenergy uses. The bioenergy industry is not likely to use these perennial forage species until they become more economical to produce. Cooperative research is needed for developing cultivars with improved biomass and quality, while protecting these crops from biotic and abiotic stress conditions. Research needs to be done on stand establishment (improved seed germination and seedling vigor), biomass production, disease and insect resistance, etc., across multiple environments, especially on marginal soils where these species are likely to be used without competing with food crops.
- Food safety: Improving the yield, nutritional quality, and storability of forage crops will ensure an ample supply of good quality feed to animals and an essential step in securing the food chain to the consumer.
Without cooperative research through the multistate project, these priorities would not be accomplished for most of the perennial forage species. The impact on providing feed for the livestock industries, especially for beef and dairy production, would be huge. Research outputs would be minimal, and cultivars would be narrowly adapted. In 2013 more than 35 M acres of hay and haylage were harvested, valued at $22.8 trillion (USDA National Agricultural Statistics Service). These figures are conservative estimates of forage production since significant acreage is devoted to pastures and rangelands. Farmers have relied on forage breeders to improve productivity of these crops, especially when new diseases, insects, and other problems have arisen.
Impacts of the proposed research will vary. Germplasm with new traits will be available to private and other public breeders to use in their programs for developing improved cultivars. Improved forage cultivars directly released from NE-1010 scientists will make seed and forage production more economical for farmers and seed companies. Development of breeding methods, both traditional and molecular methods, will enhance efficiencies and effectiveness of improving forages for traits of low heritability or from unadapted genetic backgrounds. Data from forage yield trials across multiple locations and years will be available for breeders to use for selecting experimental populations that will be released as cultivars and available for licensing, for the seed industry in advertising seed of the cultivars, and for extension educators and farmers when selecting cultivars for their locations. Development of forage species as feedstocks for the biofuel industry ultimately will contribute toward more secure and sustainable energy production. The overall impact will be more economical food and energy production while reducing negative environmental impacts in the agricultural systems.
The scientists cooperating in this project have the ability to accomplish the proposed research. The current NE-1010 project consists of most of the forage breeders from North America, who have cooperated in research for several years. In addition to scientists at state agricultural experiment stations, NE-1010 has evolved over the years to include more scientists from USDA-ARS, Agriculture and Agri-Food Canada, and the Noble Foundation. These forage breeders and scientists of other disciplines have extensive experience in research on forages. Many accomplishments already have been realized in the form of release of improved germplasm and cultivars, information on breeding methods for improving forage yield, and data on forage yield of multiple species for use by breeders, the seed industry, farmers, and extension educators. Extension presentations and information on the web have informed various stakeholders of the new information and cultivars developed by this project. Other scientists have been informed of the research results through professional publications and presentations at professional conferences. Regarding the continued or new project, the scientists have the major equipment along with field, greenhouse, and laboratory facilities to accomplish the proposed work.
Because of the long-term nature of research on perennial forage species, some of the research that was begun in the last few years will continue into the next project period. Most of the research, however, will be new as a result of the collaborative efforts and discussions during our technical committee meetings. The current project has evolved from focusing almost exclusively on traditional breeding to initiating research on new molecular genetic technologies. The cooperative multistate research project being proposed will increase the emphasis on integrating molecular technologies with traditional breeding efforts to improve forage species. Another emphasis will be on plant adaptation and resilience to changing environments due to extreme and variable weather conditions and other factors. In addition, emphasis will increase in cooperative research on plant species for biofuel use as well as use for the livestock industry.
Funding for these collaborative efforts would be only partially covered by the multistate-Hatch funding. Much of the funding would be from other sources such as the seed industry, royalties from seed sales of cultivars, private sources, and various public funding sources at the state and federal levels (primarily competitive grants). In the past, the existence of the NE-1010 project has been a key factor in helping to secure other grant funds for accomplishing the research goals, such as USDA-NIFA grants for alfalfa association analysis and genomic selection under the direction of PI Brummer.
Related, Current and Previous Work
This proposal continues a long-term regional research project that has provided multi-location selection and testing environments to a number of breeding projects. The current project, NE1010, which ends in 2017, has grown to include forage grass and legume breeders throughout the US and Canada. This breadth of geographical dispersion enables us to design experiments that cannot be accomplished by each breeder individually but that have relevance across North America. The participants of this proposal individually have research projects narrowly focused on their regions, species adapted to those regions, and the needs of producers at their locations. Our goal with this multi-location research project is to identify several major objectives that complement each location’s individual research projects but that, through the collaborative arrangements provided by this project, provide a larger geographical context in order to ensure new cultivars will have adaptability, resilience, and flexibility in different geographies, under different cropping systems or uses, and with changing weather patterns.
In this project, we have developed projects related to the three major perennial herbaceous crop groups, viz., legumes (alfalfa, birdsfoot trefoil), cool-season grasses (tall fescue, orchardgrass, meadow bromegrass), and warm-season grasses (switchgrass). While numerous other forage crops are of importance, particularly in certain regions or specific niches, these crops represent major species of interest across broad regions of the continent. Therefore, we focus on these projects which require multi-location collaboration.
As funding methods have changed over time, most of the collaborators, even those at state AES, do not receive funding for this regional research. Consequently, these projects are ones that complement existing research and can reasonably be tied with ongoing goals each collaborator has at his/her location. This also means that expensive objectives (e.g., those involving large scale genotyping or DNA sequencing) cannot be proposed within these research projects. However, by combining our programs, we can develop a framework to ask interesting questions about topics of broad interest, e.g., genotype × environment interaction, that we can then use to attract external funding. By having this regional project in place, the opportunities to attract external funding increase, and thus, this project is a key leverage point to get further funding to forage- and/or bioenergy- based projects.
Previous research results from this project (NE1010)
The previous NE1010 project included a diverse set of smaller projects that had two or several collaborators. The projects included breeding and evaluation of many forage crops, including both legumes and grasses. However, because most participants in the recent past were focused more on legumes than grasses, the outputs of the project are skewed toward legumes.
Legumes:
- Selection methods for increased yield in alfalfa are currently under multiple site evaluation.
- Genomic selection model for biomass yield shown to be effective in alfalfa.
- Alfalfa cultivars with multiple disease resistance and with improved forage yield and quality have been developed and are currently in use by dairy and other livestock producers to make those operations more economical. The most recent cultivar that was released is N-R-Gee, the first alfalfa cultivar bred for higher pectin concentration for increasing milk production in cows.
- Comparisons of breeding methods on alfalfa have been completed or are underway.
- Alfalfa association mapping with replicated clones has been completed and published.
- A potato leafhopper resistant alfalfa cultivar has been developed for the Northeast USA and will be commercialized.
- An acid tolerant alfalfa cultivar (AAC Meadowview) was developed and released for commercial production.
- A salt tolerant alfalfa cultivar (AAC Bridgeview) was released.
- A new sanfoin cultivar (AAC Mountainview) was released. This cultivar was bred for ability to survive when grown in alfalfa stands.
- An alfalfa snout beetle tolerant alfalfa cultivar (Seedway 9558 SBR) was released and is now in commercial production. Continued selection has improved resistance further.
- New birdsfoot trefoil germplasm bred for forage yield and persistence has been evaluated for potential release.
- Birdsfoot trefoil populations with higher rhizome production and vigor are being developed to enhance productivity and longevity of the crop
- Birdsfoot trefoil bred with grass companion crops has better performance in mixtures with grass than germplasm bred without companion crops.
- Comparison of selection methods for biomass yield is almost complete in multiple location trials.
- Populations were selected using a genomic prediction model. These will be increased in 2016 and tested in field trials in 2017.
- Seed of red clover populations with improved persistence, general adaptation, and biofuel use are being increased for evaluation. Marker-assisted selection strategy for red clover improvement is now in use.
Grasses:
- Selection of meadow and hybrid bromegrasses and evaluation at multiple sites.
- A model to identify desirable reed canarygrass cultivars for biofuel use was developed.
- Orchardgrass germplasm was developed with minimal flowering production, thus improving forage quality.
Multiple species:
- Mixture trials documented the value of including legumes with grasses.
- Multiple species of cool and warm season perennial forages were evaluated annually for forage yield, persistence, and other agronomic traits at multiple locations in North America to provide data on new cultivars and experimental populations to plant breeders, seed companies, extension educators, and growers. Legume/grass mixture trials are being evaluated for use as feedstocks for biofuels and livestock feed.
Relevant background for research planned in this project
Although participants in this project individually conduct breeding, genetics, and agronomic research on various forage and biofuel crops, the specific research projects we are proposing here represent aspects of these improvement programs that can be enhanced through regional collaboration. These projects are not meant to be comprehensive programs covering all, or even a few, forage and biofuel crops.
Alfalfa Improvement.
Alfalfa breeders have been highly successful at improving alfalfa disease and insect resistances over the past 75 years, so that most new cultivars today have high levels of resistance to multiple pathogens or pests (see the alfalfa variety leaflet at www.alfalfa.org). However, variable and extreme weather may create opportunities for additional disease or insect pressures, and undoubtedly the need for new resistances in the future will increase. Further, the improvement of alfalfa yield, other than that resulting from improved resistances, has been slow or non-existent over the past 25 years (Brummer and Casler, 2014). For these reasons, a broad goal for alfalfa improvement is to ensure breeding germplasm is available to assist breeders dealing with these challenges in the future. One way to address this concern is to begin prebreeding germplasm now.
The U.S. National Plant Germplasm System (NPGS) currently maintains over 3000 alfalfa (Medicago sativa L.) germplasm accessions. This collection has been a rich resource for North American alfalfa breeders, particularly as sources of disease or insect resistances. However, virtually no work has been expended in prebreeding the germplasm in this collection to develop new sources of variation for quantitative traits, such as yield and adaptation. Prebreeding can be defined as the process of converting a large number of germplasm accessions into a few adapted breeding pools that could be incorporated into commercial breeding programs. That intermediate step of making initial selections to synthesize new germplasm breeding populations is rarely done, leaving much of the collection unexplored for commercial potential.
Given the possibility for a rapid change in climate across the globe, prebreeding offers the potential of providing breeders with new, semi-improved populations to incorporate into their programs in order to counter these expected changes. At its 2010 meeting, the North American Alfalfa Improvement Conference (NAAIC, an international organization of public and private alfalfa researchers) made the systematic germplasm improvement program one of the highest priority research projects. This project is direct response to that priority setting exercise by NAAIC. Past examples using non-North American alfalfa germplasm to enhance elite alfalfa germplasm pools include the development of leafhopper resistant alfalfa (Elden and McCaslin, 1997), lodging tolerant alfalfa (Lamb et al., 1997), disease resistance (Elgin et. al, 1988). Numerous studies have also show that genetically distinct alfalfa germplasm could be used as the basis for obtaining yield heterosis in hybrid or semi-hybrid alfalfa breeding schemes (Dudley and Davis, 1966; Busbice and Rawlings, 1974; Riday and Brummer, 2002; Bhandari et al., 2007). Over the past decade, a program to pre-breed pure yellow flowered alfalfa germplasm (subsp. falcata) has been underway (Riday and Wagner, 2012), including several breeding and evaluation projects within the NE1010 regional project).
Historically, alfalfa germplasm was classified into pools based on region of origin, most notably, in the synthesis by Barnes et al. (1977). However, defining pools of extant breeding germplasm in 2016 is more difficult, given the extensive mixing that has occurred over the past century; if anything, cultivars appear to be structured according to fall dormancy classification (Li et al., 2013), possibly reflecting past introgression of falcata germplasm. A number of molecular marker analyses have been conducted in various alfalfa germplasms (reviewed in Li and Brummer, 2011), and several more recent analyses have focused on putatively wild diploid (Şakiroğlu et al., 2010) and tetraploid accessions (Ilhan et al., 2016). Across all these experiments, the differentiation of yellow and purple flowered taxa is well established. Among tetraploids, however, a clear gradation is obvious with the hybrid subsp. xvaria falling intermediate between the two ends, effectively creating a continuum between true sativa and true falcata types. Alfalfa germplasm most likely follows a diversity ‘cline’ subject largely to isolation by distance based on continental geography. More fully understanding overall genetic variation, would be useful in helping to structure future breeding programs and to preserve and incorporate germplasm resources.
For all these reasons this project is desirable and necessary to more fully exploit the NPGS germplasm system to pre-breed alfalfa to be utilized by North American alfalfa breeders in a systematic way. Work is already fully underway to develop a yellow-flowered ‘falcata’ pool. The project described here would seek to emulate and expand this work by developing multiple geographic based germplasm pools in the subsp. sativa ranges.
Switchgrass Improvement.
Switchgrass (Panicum virgatum L.) is a native warm-season C4 perennial range grass that is a target feedstock for U.S. production of sustainable cellulosic biofuels, electricity, and synthetic gas (Boateng et al, 2007; Schmer et al., 2008). The principal, on-going objective of switchgrass breeding programs is to select for and develop cultivars with high biomass production (Parrish et al., 2012). However, high yield depends on the cultivar’s ability to tolerate environmental and biotic stresses. Currently, large monoculture biofuel plantings have not been sown, but when they will be, disease pressure will increase, negatively impacting yield (Crouch et al., 2009; Uppalapati et al., 2013; Zhu et al., 2013; Stewart and Cromey, 2011). Therefore, understanding the epidemiology of and the genetic variation for resistance to the most serious disease pathogens needs to be undertaken now, so successful cultivars will be available in the future.
Phytopathogenic fungi with explosive disease potential will likely become more prevalent and economically damaging as switchgrass plantings expand (Casler et al., 2011; Aguirre et al., 2012; Casler and Vogel, 2014; Kenaley et al. 2016). More than 150 fungal species have been found to infect switchgrass, including anthracnose (Colletotrichum navitas), leaf spot (Bipolaris oryzae), smut (Tilletia maclaganii), and rust (Puccinia emaculata and Uromyces graminicola) fungi (Farr and Rossman, 2016). Substantial yield loss attributable to fungal pathogens has already been demonstrated (Gravert et al., 2000; Thomsen et al., 2008; Fajolu, 2012; Sykes et al., 2016). For example, rust infection of switchgrass has recently been demonstrated to reduce ethanol yield up to 55% (Sykes et al., 2016) and three species of rust fungi have been determined to affect commercially important switchgrass cultivars throughout the north-central and eastern U.S. (Kenaley et al., 2016).
The rust fungi are particularly acute and recurrent threats to switchgrass (Cornelius and Johnston, 1941; Uppalapati et al., 2013; Sykes et al., 2016). Because of their high evolutionary rate and explosive disease potential, rust fungi historically are among the most serious and damaging pathogens of agricultural crops worldwide (Strange and Scott, 2005). Four species of rust fungi reportedly cause disease in switchgrass: P. emaculata, P. graminis, P. huberi, and U. graminicola (Burrill, 1884; Arthur, 1934; Ramachar and Cummins, 1963, 1965; Anonymous, 1970; Cummins, 1971). Puccinia emaculata has received most of the attention as it is reportedly distributed across the eastern two-thirds of North America (Ramachar and Cummins, 1965; Lenne, 1990; Farr and Rossman, 2016) and has caused recurrent outbreaks on cultivated switchgrass in the central and eastern U.S. (Gravert and Munkvold, 2002; Gustafson et al., 2003; Zale et al., 2008; Hirsch et al., 2010; Gilley and Tomaso, 2011; Frazier et al., 2013; Uppalapati et al., 2013; Kenaley et al., 2016). However, the geographic distribution and presence of P. graminis, P. huberi, and U. graminicola, either alone or in combination with P. emaculata, on switchgrass remains unknown. Moreover, P. emaculata may be a compound species consisting of two or more morphologically convergent taxa (Arthur, 1934; Kenaley et al., 2016).
Switchgrass harbors substantial natural genetic variation for many traits, and that variation appears to extend to disease resistance. Much genetic variation within switchgrass is partitioned between upland and lowland ecotypes, driven by adaptation to habitats differing in soil water availability and other factors (Porter et al., 1966; Lowry et al., 2014). In addition, many genetically-based abiotic stress responses and phenological traits vary by latitude (McMillan, 1964; Aspinwall et al., 2013; Lowry et al., 2014). Genetic variation in switchgrass disease resistance likewise differs both between ecotypes and with latitude (Gustafson et al., 2003; Uppalapati et al., 2013; Serba et al., 2015). Recent studies have shown that the southern lowland ecotype is generally more resistant to fungal diseases, including anthracnose, Bipolaris leaf spot, rust, and head smut, than the northern upland ecotype. One of the primary reasons that southern lowland cultivars have greater disease resistance is because they are exposed annually and persistently to a greater diversity of pathogens. Pathogen diversity often increases at lower latitudes where pathogen populations are less likely to experience local extinction and recolonization events.
At present, breeders have a limited understanding of the genetics of resistance to the multiple microbial pathogens that infect switchgrass (Gustafson et al., 2003; Schrotenboer et al., 2011; Uppalapati et al., 2013; Serba et al., 2015). However, no previous study has evaluated pathogen diversity across geographically distinct switchgrass populations at the national scale. Thus, our research presents an unparalleled opportunity to determine pathogen diversity and host-pathogen combinations as well as to evaluate germplasm for resistance to these disease agents. Moreover, given that pathogen diversity differs by region, the success of the proposed work will necessitate multistate collaboration.
Cool-season Grass Improvement.
Cool-season (C3) grasses comprise a major forage source in both the United States and Canada. Cool-season grasses are grown both in monoculture plantings and mixed with legumes and stands are managed for grazing, hay, and/or silage production. Meadow bromegrass (Bromus riparius Rehm.), tall fescue (Lolium arundinaceum (Schreb.) Darbys.) and orchardgrass (Dactylis glomerata L.) are three important cool-season grasses used in temperate regions of North America. While tall fescue and orchardgrass are widely grown and are well known to farmers, meadow bromegrass is now recommended across Canada (Knowles et al., 1993) and interest in the U.S. is increasing with new cultivars being developed in Montana and Utah. The increased consumer demand for grass-fed and organic animal products and producer needs for increased sustainability necessitate further advances in the improvement of cool-season grasses for North American production. Maintaining or expanding the seeded acreages of cool-season grasses will only occur if new cultivars with broader adaptation and/or novel traits are developed.
The previous NE1010 project developed a meadow bromegrass breeding population by crossing superior plants selected at four locations across North America. However, this germplasm has not been tested widely. Tall fescue is recommended across North America; however, improving its palatability and nutritive value would expand its use. New soft-leaf tall fescue populations developed in Utah may be useful if they prove to be adapted across the tall fescue growing regions of North America and the soft-leaf trait, which improves palatability, proves to be expressed in all environments. Orchardgrass is cultivated in the northern regions of United States and in all Canadian provinces. In Europe, increased concentrations of water soluble carbohydrates (WSC) in grasses provided increased animal production and decreased environmental impacts (Miller et al., 2001; Lee et al., 2001). Increased WSC may also buffer plants against various abiotic stresses, including drought and freezing (Volaire and Thomas, 1995; Sanada et al., 2007). No cultivars have been developed in North America for higher WSC, despite the value of the trait, and consequently, no research has been done to determine if higher WSC have value and are reliably expressed under the management systems and, importantly, the climates found in North America. The development of cultivars with increased levels of WSC is a new breeding objective of forage grass breeding programs in recent years.
Birdsfoot trefoil improvement.
Birdsfoot trefoil, as a non-bloating legume, is a desirable component of grazing systems. Breeding programs have focused on improving persistence and seedling germination. One of the characteristics that make trefoil a useful forage legume is their condensed tannin (CT) content. The quantity of CT present in legumes has been shown to correlate with animal productivity as well as impact gastrointestinal parasites in forage-fed ruminants (Waghorn and McNabb, 2003). Condensed tannin is a term that includes a group of diverse secondary compounds. The specific compounds associated with the above improved animal performance were not identified.
Research into the impact of CT on the parasitic gastrointestinal nematodes that are parasitic to livestock has suggested the possibility that higher CT content of forage legumes will reduce the levels of infection, (Hoste et al., 2006; Marley et al., 2006; Waghorn and McNabb, 2003). Assessing the CT profiles and content among currently available birdsfoot trefoil cultivars and elite lines will aid in estimating available genetic variability for this trait.
Relation to other CRIS/REEIS research
Several of these projects are also part of other CRIS projects. Many CRIS/REEIS reports were identified for alfalfa germplasm development, but they involved developing specific traits in elite germplasm in plant breeding programs. The new NE multistate project would enhance adaptation of alfalfa germplasm developed mostly from plant introductions that have not previously been used in plant breeding programs. This enhanced germplasm would to provide new germplasm for future cultivar development by alfalfa breeders in North America. A few CRIS/REEIS reports are about research on switchgrass diseases, but none of these reports described surveys of switchgrass diseases across North America.
CRIS/REEIS reports indicate that research has been done on gastrointestinal parasites that affect health and performance of small ruminants. Much of the research has focused on animals and hence is not relevant to our project. Of the research focused on birdsfoot trefoil, most of it has been done at the University of Rhode Island by K. Petersson and others. One of our multistate project researchers (Rebecca Brown) is now located there. We plan to expand on this research by sampling birdsfoot trefoil cultivars in experimental field trials across multiple locations in the USA and Canada.
Objectives
-
Developing broadly adapted, climate resilient forages for sustainable cropping systems.
Comments: This objective has four sub-objectives. 1.1. Developing regionally adapted, resilient alfalfa germplasm pools. Cooperating locations: AES: Cornell Univ., South Dakota State Univ., and Univ. California, Davis [co-lead]; USDA-ARS: Logan, UT and Madison, WI [co-lead]; AAFC: Québec, QC, Saskatoon, SK, and Truro, NS; Noble Foundation, Ardmore OK. 1.2. Developing switchgrass germplasm with improved fungal pathogen resistance. Cooperating locations: AES: Cornell Univ. [lead], Mississippi State Univ., Rutgers Univ., South Dakota State Univ.; USDA-ARS: Madison, WI. 1.3. Developing resilient cool-season grasses adapted to variable climatic conditions. Cooperating locations: AES: Cornell Univ.; South Dakota State Univ.; Univ. California, Davis; Univ. Kentucky, and Univ. Minnesota; USDA-ARS: Logan, UT [co-lead] and Madison, WI; AAFC: Québec, QC and Saskatoon, SK [co-lead]. 1.4. Determining the extent of genetic variability of CT among currently available birdsfoot trefoil cultivars and elite lines. Cooperating locations: AES: Cornell Univ., Univ. Rhode Island; USDA-ARS: Logan, UT and Madison, WI; AAFC: Truro, NS [lead]. -
Understanding genotype by environment interactions across multiple forage species
Comments: Cooperating locations: AES: Auburn Univ., Cornell Univ., Mississippi State Univ., Rutgers Univ., South Dakota State Univ., Univ. California, Davis, Univ. Florida, Univ. Georgia, Univ. Kentucky, Univ. Minnesota, Univ. Rhode Island, Univ. Tennessee; USDA-ARS: Logan, UT and Madison, WI; AAFC, Lethbridge, AB, Québec, QC, Saskatoon, SK, and Truro, NS; Noble Foundation, Ardmore OK.
Methods
- Developing broadly adapted, climate resilient forages for sustainable cropping systems.
The four subobjectives described here are natural outgrowths of the existing breeding programs of the participants. These four project complement and extend individual programs and could not be conducted without regional (or continent-wide) participation.
1.1 Developing regionally adapted, resilient alfalfa germplasm pools
The overarching goal of this project is to use the NPGS alfalfa germplasm collection to enhance genetic diversity in elite North American alfalfa breeding pools. Selection from the NPGS pools will be organized into region of origin germplasm pools that can be useful for long-term genetic improvement of alfalfa and potentially valuable for the creation of heterotic groups and hybrid cultivars. The project consists of a series of related experiments that by nature are collaborative across multiple North American locations. This objective is an outgrowth of a long-term discussion at the North American Alfalfa Improvement Conference (NAAIC) on using the germplasm collection for long-term improvement.
Germplasm pool development. Based largely on accessions that are documented as landraces or cultivars from regions outside North America, we will develop pools based on Northern (fall dormancy levels from 1-5) and Southern adaptation (fall dormancy 5- 12). Populations will be developed that derive from discrete ecogeographic regions. We propose to have four Northern and four Southern pools. Northern pools: include Siberia/Mongolia, Central Asia, Balkans-Turkey-Black Sea Region, and North Eastern Europe to the Ural Mountains; Southern pools from South America, North Africa, Southern Asia (India, Iran), and the Arabian Peninsula. In conjunction with those regional pools, we plan to develop two broadly based populations by pooling the four regional pools.
Evaluation/selection within new germplasm pools for broad adaptation. We expect that multiple cycles of selection will be necessary for these pools to be both broadly adaptable to North American climates and useful in commercial breeding programs. Therefore, we will undertake multi-location breeding nurseries to select plants within populations to develop improved versions of these germplasms. In addition to scientists involved at the outset of this project, we will also invite other breeders to participate either by becoming members of the regional project itself of by conducting evaluation trials.
Diversity evaluation of germplasm pool. In order to validate our germplasm pool approach we will conduct DNA marker based diversity studies of developing pools using falcata as outgroup to determine how distinctive the eco-geographic regional germplasm pools are in relationship to the North American elite alfalfa germplasm pool. This analysis could help us also define less utilized regional germplasm sources and guide further enhancement efforts.
Seed increases and yield/performance trials of new germplasm pools. Seed of the germplasm pools will be increased to provide sufficient seed quantities for yield evaluation at multiple locations. New germplasms are expected as part of this project that will be freely available to commercial alfalfa breeding interests through the NPGS system.
Characterization of pools for various biotic and abiotic stresses in conjunction with on-going research projects of participants. Including reference cultivars and using standard test procedures where available (NAAIC standard tests), we will characterize the new pools for various traits, including (but not limited to) fall dormancy, insect/disease resistance, salinity, winter hardiness, and waterlogging tolerance. Where appropriate, we will select from these populations to develop new germplasm that is enhanced for these traits. We will work with other US alfalfa scientists in the public and private sectors to evaluate key insect and disease resistances of most importance nationally and within specific regions.
Release of germplasm to the public. All populations developed through this project will be made publicly available through the National Plant Germplasm System, accessable through the Germplasm Resources Information Network, and also through individual scientists involved in the project.
1.2 Developing switchgrass germplasm with improved fungal pathogen resistance.
As biofuel production expands, planting of large monocultures of switchgrass will likely result in increased disease, especially those caused by fungal pathogens. In order to develop switchgrass germplasm and cultivars with resistance to fungal pathogens, breeders first need to understand which pathogens are present in likely biofuel growing regions, how the pathogens are distributed throughout the US and Canada, and whether resistance to these pathogens is present. Because no previous study has evaluated pathogen diversity at the national scale, this project presents an unparalleled opportunity to evaluate germplasm for resistance to these disease agents. Given that pathogen diversity differs by region, the success of the proposed work requires multistate collaboration.
Quantify important switchgrass pathogens across the US. We will characterize the geographic distribution and severity of economically important/yield-reducing diseases caused by phytopathogenic fungi in the north-central, eastern, and south-eastern United States through surveys of common cultivars and breeding lines. Fungi can cause a range of mild to severe disease in plant hosts. However, disease symptoms or signs of phytopathogenic fungi may not immediately reveal the specific identity of a pathogen. Therefore, quantification of diseases in switchgrass requires both field assessments of disease severity and analytical laboratory procedures to identify the specific pathogens. Thus, we will first quantify the severity of fungal diseases in switchgrass with an annual survey (2017-2020) of all commercially important and promising new lines of switchgrass in yield trials and nurseries in Mississippi (Mississippi State University), New Jersey (Rutgers University), New York State (Cornell University), South Dakota (South Dakota State University), and Wisconsin (USDA). Disease surveys will focus on determining the severity of four diseases: 1) rust (Puccinia emaculata and Uromyces graminicola); 2) anthracnose (Colletotrichum navitas); 3) Bipolaris leaf spot; and, 4) head smut (Tilletia species). For each survey, collaborators will score only promising new lines and commercially important cultivars for infection using established, standardized visual disease severity rating systems (e.g., for rust, 0-9 Puccinia emaculata system). Because different pathogens are detectable at different times, surveys for smut will be executed in summer (July or early August) during or shortly after flowering whereas surveys for rust and the other foliar disease will be conducted during peak infection late in the growing season (mid-September). From these surveys, we will establish how disease severity varies by geographic location, differs between northern upland and southern lowland populations, and differs among germplasm within ecotypes.
Identify and quantify specific host-pathogen relationships. We will identify patterns of phenotypic variation for switchgrass resistance and, hence, susceptibility to fungal pathogens across common cultivars, breeding lines, and field sites. We have noted previously that varying fertilization regimes (esp. nitrogen application rate) and summer rainfall can significantly influence the incidence and severity of switchgrass disease, particularly anthracnose and head smut (S.C. Kenaley, Cornell Univ. unpub. data).
Thus, we predict that the extent and severity of pathogen infection will differ by site and be influenced significantly by local environmental factors. To identify environmental factors associated with the severity of disease(s), we will compile a set of environmental covariates for each field site including: (i) 2017- 2020 weather data, obtained from PRISM; (ii) soil fertility (established by collaborators); (iii) proximity of alternate hosts for foliar pathogens of switchgrass; and (iv) extent of vegetation types within 1 km, as determined from field observation and the USDA National Agricultural Statistics Service Cropland Data Layer. To determine how landscape features predict genotype-specific disease severity, we will conduct stepwise general linear model fitting. Here, the response variable will be disease severity and the landscape environmental factors will be predictor variables. Model comparison using Akaike information criterion (AIC) will be executed to identify best-fit models.
Pathogen resistance in switchgrass. We will quantify pathogens across common cultivars and breeding lines to identify switchgrass germplasm possessing tolerance and/or resistance to regional phytopathogenic fungi. We will compare results among field sites to identify regionally adapted switchgrass plants for possible cultivar release and further downstream genetic analyses. We will collect fungi with switchgrass leaves annually in mid-summer to early fall 2017-2020 at all sites. Culturable fungi will be identified by morphological analysis, whereas for rust and smut fungi, will be identified based on teliospore measurements and DNA marker results. We will conduct phylogenetic and phylogeographic analyses among sampled taxa using single-locus or multilocus sequence data and three separate phylogenetic approaches (maximum-likelihood, maximum parsimony, and Bayesian inference of phylogeny). We will conduct phylogeographic analyses to understand how species diversity is distributed across our field sites. Drs. Shawn Kenaley and Gary Bergstrom (Cornell Univ.) have conducted the only morphological and phylogenetic analyses of switchgrass rust fungi to date and, hence, have the expertise and procedures to successfully complete the aforementioned molecular and morphometric analyses. Pathogen identification will be critical for understanding patterns in host-pathogen combination(s) and why some cultivars/breeding lies express disease resistance at some sites but not others.
New resistant switchgrass germplasm. Through the evalations of fungi populations described above, we will be able to identify switchgrass populations and/or genotypes that are resistant or tolerant to one or more fungal pathogens. Resistant germplasm will be evaluated for biofuel characteristics and considered for release, based on the release protocols of the institutions involved in the project. Further selection, intercrossing, and population development will be conducted by the partners to generate highly productive, disease resistant cultivars.
1.3 Developing resilient cool-season grasses adapted to variable climatic conditions.
This project will develop cultivars and breeding lines of important cool-season grasses (meadow bromegrass, tall fescue, and orchardgrass) across multiple North American locations.
- Evaluate and select meadow bromegrass breeding lines to understand the G x E interaction, and to develop adapted cultivars
- Evaluate soft-leaf tall fescue populations to select adapted populations.
- Quantify water-soluble carbohydrate expression and cell wall digestibility of tall fescue and orchardgrass at different growth environments to understand the underlying genetic basis.
- Select individual genotypes from new orchardgrass populations to develop populations with improved winter hardiness.
Meadow bromegrass cultivars with improved forage value. Five populations of meadow bromegrass will be characterized for biomass production, nutritive value, survival, and seed production at Logan, UT; Saskatoon, SK; Québec, QC; and Madison, WI for three production years. Biomass and nutritive value will be collected during the first two production years, and seed production will be collected during the third production year. Survival data will be collected each year. The experimental design at each location will be a randomized complete block design consisting of four complete blocks. Each population will be represented in each block by a plot consisting of forty genotypes. Based on the data collected from each location, the best genotypes will be identified and selected for the development of four new meadow bromegrass germplasms.
Adapted tall fescue and orchardgrass cultivars with improved nutritive value. Five populations of tall fescue and fifty half-sib families of orchardgrass will be evaluated for winter injury, survival, water-soluble carbohydrate content, and cell wall digestibility at Logan, UT; Panguitch, UT; Saskatoon, SK; St. Paul, MN; Quebec City, QC; and Normandin, QC field sites. Data will be collected for three production years at each location to determine the relationship between water-soluble carbohydrate expression with winter injury, digestibility, and survival under varying precipitation levels. Based on the data collected from each location, the best families will be selected for development of an improved orchardgrass germplasm based on multi-location selection.
1.4 Determining the extent of genetic variability of CT among currently available birdsfoot trefoil cultivars and elite lines
Assess the CT profile and content of birdsfoot trefoil germplasm across diverse climatic conditions in the northern latitudes. This investigation will be initiated by establishing small, replicated plots of birdsfoot trefoil cultivars and elite lines in regions where the investigators are located. In 2019 and 2020, prior to harvesting, the plots will be assessed for development of stage. Plots will be sampled for CT profile and content and harvested for determine forage yield.
2.0. Understanding genotype by environment interactions across multiple forage species
To provide multilocation evaluation data for broadly adapted as well as location specific germplasm developed by members of the NE1010 committee either jointly or independently.
Cooperating locations where testing will be conducted will be identified for each species and an individual from one of the locations will act as the coordinator. Seed of each entry will submitted by each cooperating location to the coordinator of the trial, entries will typically not exceed four to six entries. For each species a core set of check cultivars will be included for testing. Seed from each cooperating location and the core set of cultivars will be distributed by the coordinator to each cooperating location. These will then be established following local practices in small plot trials and will be maintained for two to three years following the establishment year.
Each location will collect a core set of data. Seedling vigor and plant stand in the establishment year, forage yield from a minimum of one harvest in the two to three years following establishment, forage quality data where the local cooperator has that capability and persistence following the last harvest in the second year following establishment. Each location will collect data unique to the biotic and abiotic stresses for their specific environment. Individual locations may choose to include additional check cultivars when a test is specific to their location. At the conclusion of the final harvest year, raw/rep data will be sent from each cooperating location to the coordinator of that crop. This should occur no later than mid November. The coordinator for that crop will analyze and summarize the data, reporting G × E interactions for establishment, forage yield, and persistent. All data, including that unique to specific locations, will be summarized in mean tables, which will then be distributed to all cooperators.
Measurement of Progress and Results
Outputs
- Activities Comments: • Conducting and analyzing data from experiments on alfalfa, switchgrass, cool-season grasses and birdsfoot trefoil • Mentoring undergraduate, graduate, and postdoctoral scientists in plant breeding, agronomy, and pathology • Training students and staff in proper experimental design and research techniques
- Events Comments: • Presentations of research results at national and international conferences • Demonstrations of new germplasm at cooperating locations • Field days for producers and seed companies to showcase new germplasm and cultivars
- Services Comments: • Consultation and discussion with seed companies about licensing new cultivars
- Products Comments: Germplasm or cultivars • Ten new broad-based alfalfa germplasm populations. • Disease resistant switchgrass germplasm. • Four improved meadow bromegrass germplasms. • Orchardgrass and tall fescue germplasms with improved water soluble carbohydrate concentration, nutritive value, and broad geographic adaptation. • Birdsfoot trefoil germplasm with improved concentration of bioactive compounds.
- Scientific data Comments: • Agronomic data on the new alfalfa populations across North American testing locations • Incorporation of alfalfa data generated from this project into the Alfalfa Breeders’ Toolbox developed at the Noble Foundation and integration with other alfalfa databases, including the alfalfa genome sequence and gene expression atlas. • Deposition of DNA sequence data for rust and smut fungi in public genetic databases (e.g., GenBank). • Adaptation data useful for breeders in making cultivar release decisions.
- Scientific publications Comments: • Analysis of alfalfa genetic diversity using genetic markers. • Agronomic value of broad-based alfalfa germplasm pools.Documentation of the severity of fungal diseases among switchgrass ecotypes across time and space. • Identification of environmental factors that are most predictive of switchgrass disease severity by region. • In-depth analyses of switchgrass pathogen diversity.Assessment of geographic distribution of fungal pathogens affecting upland and lowland ecotypes in temperate region of U.S. • Determination of unique host-pathogen relationships across the U.S. • Understanding of the relationship between water-soluble carbohydrate expression and abiotic stress and the underlying quantitative genetics of these traits in orchardgrass and tall fescue. • Identification of the magnitudes of genotype × environment interaction of bioactive compounds in trefoil. • Understanding of genotype by environment interactions across North America for major forage species.
- Extension activities and information Comments: • Data for seed dealers and extension specialists in making cultivar recommendations, and forage producers in choosing cultivars that meet their needs. • Extension information especially on websites to disseminate results to the to producers.
Outcomes or Projected Impacts
- Change in knowledge • Increase in the alfalfa germplasm available to breeders in North America to develop improved cultivars more resilient and better adapted to climate stress in the future. • Increase in improved forage cultivars that enhance the economics of livestock producers. Seed sales of new cultivars, when available. • Increase in scientific knowledge through journal papers that will assist other scientists in future plant breeding activities. • Increase in understanding the geographic distributions of pathogen communities and species diversity affecting switchgrass.
- Change in action • Increase preliminary data to enable future grant proposal development (e.g., alfalfa germplasm structure and use; switchgrass QTL mapping and marker development for pathogen resistance; water soluble carbohydrate analysis in grasses; bioactive compounds in trefoil.) • Increase availability of disease resistance markers to accelerate selective breeding of switchgrass cultivars adapted to regional abiotic stressors and pathogen communities. • Data that provide producers with options for cultivar selection, planting and harvesting to optimize production. • Increase in improved germplasm will be provide material for further breeding and/or immediate commercialization with improved adaptation and resilience for production and persistence under pasture and hay production systems.
- Change in condition • Increased acreage of cultivars with high forage yield and quality will result in enhanced economic vitality of forage and livestock production operations.
Milestones
(2017): Evaluate establish nurseries containing germplasm from the first version of the four northern pools (collectively N1.0). Evaluate existing NPGS germplasm nurseries from southern pools (NPGS-S-2016). Screen additional germplasm from NPGS for inclusion in northern pools (NPGS-N-2017) and southern germplasm pools (NPGS-S-2017). Evaluate, analyze and summarize general patterns of diseases – and their causal fungi - across field sites. Collect landscape level data – weather, prevalence of alternate host(s), soil fertility, and vegetation type neighboring fields. Establish grass evaluation nurseries. Establish birdsfoot trefoil research plots. Establish GxE evaluation trials.(2018): Final evaluation year of N1.0 make selections to form N2.0. Select out of NPGS-S-2016 to form S1.0 (collectively southern germplasm pools). Continue to evaluate NPGS-N-2017 and NPGS-S-2107. Re-execute disease surveys and fungal species identification. Analyze two-year data sets to assist in the identification of switchgrass cultivars and breeding lines expressing phenotypically differing levels of disease resistance and severity (i.e., unique host-pathogen combinations). Collect phenotypic data from grass trials. Obtain herbage samples for the assessment of isoflavone content, as well as evaluate plant persistence and herbage yield. Continue harvesting GxE evaluation trials.
(2019): Final evaluation year of NPGS-N-2017 and NPGS-S-2107 make selections to form N1.1 and S1.1. Conduct a larger seed increase of S1.0 and N2.0. Begin registration process of N1.0 populations as germplasm for inclusion in NPGS. Begin multi-location testing of S1.0. Conduct disease and pathogen surveys. Analyze three-year data and conduct phylogenetic analyzes for rust and smut fungi. Submit fungal DNA data to public genetic databases. Continue phenotypic evaluations for grass trials for a second year. Obtain herbage samples for the assessment of isoflavone content, as well as evaluate plant persistence and herbage yield for a second year. Plant additional GxE trials and continue harvesting existing trials.
(2020): Begin evaluation nursery testing of N1.1, S1.1. Germplasm characterization testing of N2.0. Continue evaluating S1.0. Execute final surveys for diseases and pathogens. Integrate survey and landscape level data to examine predictive environmental variables contributing to the severity of individual switchgrass diseases. Determine switchgrass plants with resistance to one or more diseases and mark them for vegetative propagation. Create crossing blocks to develop improved grass germplasm populations. Complete statistical analysis for trefoil study and discuss project results. Analyze data of evaluation trials. Continue to establish new trials and harvest existing trials.
(2021): Final year evaluation of S1.0 make selections to form S2.0. Continue evaluation of N1.1 and S1.1. Continue N2.0 characterization testing. Summarize project results and prepare manuscript for publication in a peer-reviewed journal. Utilize these data as leverage in procuring additional funding for quantitative trait loci analyses of resistant germplasm and, thereafter, the development of molecular markers in switchgrass for the identification of genes conferring resistance to one or more diseases. Seed production of grass germplasms. Data analysis, writing, and publication of journal papers for all projects (likely begins in previous years) Investigate relationships among species for GxE.
Projected Participation
View Appendix E: ParticipationOutreach Plan
All members of the technical committee are involved in outreach to the scientific community, the seed industry, and the farm community in their region. The primary means of outreach to the scientific community include publications in peer reviewed journals and presentations at conferences. Many of the members of the technical committee have active breeding programs that release cultivars, and these members typically have connections with seed companies to market their cultivars. The technical committee will work to enhance communication between scientists and industry colleagues to more effectively transfer results to industry and also to ensure research is being conducted on topics of relevance to the industry. In the current proposal, the alfalfa germplasm project already has S&W Seeds as a collaborating member and will involve other alfalfa companies as the project continues. Similar efforts to engage with seed companies will be made for the other objectives. For all objectives, the ultimate goal is to develop cultivars that can be licensed to seed companies and sold to farmers or other users. Finally, all members of the committee routinely speak at extension or grower meetings in their respective locations and work to ensure that extension personnel know about and are conversant on their research programs.
Throughout the life of the project, we will regularly invite other forage breeders, pathologists, entomologists, physiologists, and agronomists in the public and private sectors to collaborate on aspects of the projects, as needed. Any of these participants are also welcome to join the project as official members. Our goal is to be as inclusive as we can be to ensure we reach our objectives.
Organization/Governance
This project is organized by objective, with each objective having one or more lead scientists. Like the current NE1010 project, the lead scientists will prepare annual summaries of research in their objective (or sub-objective) and lead the discussion at the annual meeting. These scientists are tasked with keeping the objective moving forward, to meeting the objectives in a timely manner, and tracking to ensure that each participant is keeping their part of the project going according to plan. All other participants contribute updates on their work.
The annual meetings have a chair and a secretary, who typically rotates to chair the succeeding year. The secretary for the next meeting is elected by the membership each year.
Literature Cited
Aguirre AA, Studer B, Frei U, Lübberstedt. 2012. Prospects for hybrid breeding in bioenergy grasses. Bioenergy Research 5: 10–19.
Anonymous. 1970. Index of Plant Diseases in the United States. United States Department of Agriculture, Agricultural Handbook No. 165. U.S. Government Printing Office, Washington, D.C. 531 p.
Arthur JC. 1934. Manual of the rusts in United States and Canada. Purdue Research Foundation, Lafayette, IN. The Science Press Printing Co., Lancaster, PA. 438 p.
Aspinwall MJ, Lowry DB, Taylor SH, Juenger TE, Hawkes CV, Johnson M-VV, Kiniry JR, Fay PA. 2013. Genotypic variation in traits linked to climate and aboveground productivity in a widespread C4 grass: evidence for functional trait syndrome. New Phytologist 199: 966–980.
Barnes, D. K., Bingham, E. T., Murphy, R. P., Hunt, O. J., Beard, D. F., Skrdla, W. H., & Teuber, L. R. 1977. Alfalfa germplasm in the United States: genetic vulnerability, use, and maintenance. USDA-ARS Tech. Bull,1571.
Bhandari, H.S., C.A. Pierce, L.W. Murray, and I.M. Ray. 2007. Combining abilities and heterosis for forage yield among high-yielding accessions of the alfalfa core collection. Crop Sci. 47:665-671.
Boateng, A.A., Banowetz, G.M., Steiner, J.J., Barton, T.F., Taylor, D.G., Hicks, K.B., El Nashaar, H., Sethi, V.K. 2007. Gasification of kentucky bluegrass (Poa pratensis L.) straw in a farm-scale reactor. Biomass and Bioenergy, 31:153-161.
Burrill TJ. 1884. New species of Uredineae (collection made by A. B. Seymour). Botanical Gazette 9: 187–191.
Busbice, T.H., and J.O. Rawlings. 1974. Combining ability in crosses within and between diverse groups of alfalfa introductions. Euphytica 23:86-94.
Casler MD, Tobias CM, Kaeppler SM, Buell CR, Wang Z-Y, Cao P, Schmutz J, Ronald P. 2011. The switchgrass genome: tools and strategies. The Plant Genome 4: 273–282.
Casler MD, Vogel KP. 2014. Selection for biomass yield in upland, lowland, and hybrid switchgrass. Crop Science 54: 1–11.
Cornelius DR, Johnston CO. 1941. Differences in plant type and reaction to rust among several collections of Panicum virgatum L. Journal of the American Society of Agronomy 33: 115–124.
Crouch JA, Beirn LA, Cortese LM, Bonos SA, Clarke BB. 2009. Anthracnose disease of switchgrass caused by the novel fungal species Colletotrichum navitas. Mycological Research 113: 1411–1421.
Cummins GB. 1971. The Rust Fungi of Cereals, Grasses and Bamboos, 2nd edn. Springer-Verlag, New York. 570 p.
Dudley, J. W., and R.L. Davis. 1966. Preliminary groupings of plant introductions of alfalfa (Medicago sativa, L.) for heterosis studies. Crop Sci. 6:597-600.
Elden, T.C., and M. McCaslin. 1997. Potato leafhopper (Homoptera: Cicadelidae) resistance in perennial glandular-haired alfalfa clones. J. Econ. Entomol. 90:842-847.
Elgin, J.H. Jr., R.E. Welty, and D.B. Gilchrist. 1988. Breeding for disease and nematode resistance. In Alfalfa and alfalfa improvement (Ed. A.A. Hanson, D.K. Barnes, and R.R. Hill Jr.). ASA-CSSA-SSSA, Madison, WI. p827-858.
Ellis JG, Lagudah ES, Spielmeyer W, Dodds PN. 2014. The past, present and future of breeding rust resistant wheat. Frontiers in Plant Science 5 (Article 641): 1–13.
Fajolu OL, Vu AL, Dee MM, Zale J, Gwinn KD, Ownley BH. 2012. First report of leaf spot and necrotic roots on switchgrass caused by Curvularia lunata var. aeria in the United States. Plant Disease 96: 1372.
Farr DF, Rossman AY. 2016. Fungal Databases, Systematic Mycology and Microbiology Laboratory, ARS, USDA. http://nt.ars-grin.gov/fungaldatabases/. Accessed 26 July 2016.
Frazier T, Shen Z, Zhao B, Bush E. 2013. First report of Puccinia emaculata infection on switchgrass in Virginia. Plant Disease 97: 424.
Gilley M, Tomaso-Peterson M, Orquera GK, Marek SM. 2013. First report of rust caused by Puccinia emaculata on switchgrass in Mississippi. Journal of the Mississippi Academy of Science 58: 197–200.
Gravert C, Munkvold G, 2002. Fungi and diseases associated with cultivated switchgrass in Iowa. Journal Iowa Academy of Science 109: 30–34.
Gravert CE, Tiffany LH, Munkvold GP. 2000. Outbreak of smut caused by Tilletia maclaganii on cultivated switchgrass in Iowa. Plant Disease 84: 596.
Gustafson DM, Boe A, Jin Y. 2003. Genetic variation for Puccinia emaculata infection in switchgrass. Crop Science 43: 755–759.
Hirsch RL, TeBeest DO, Bluhm BH, West CP. 2010. First report of rust caused by Puccinia emaculata on switchgrass in Arkansas. Plant Disease 94: 381.
Hoste, H., Jackson, F., Athanasiadou, S., Thamsborg, S., and Hoskin, S. 2006. Trends in Parasitology 22 (6).
Hulbert S, Pumphrey M. 2014. A time for more booms and fewer busts? Unraveling cereal-rust interactions. Molecular Plant-Microbe Interactions 27: 207–214.
İlhan, D., X. Li, E. C. Brummer, and M. Şakiroğlu. 2016. Genetic diversity and population structure of tetraploid accessions of the Medicago sativa–falcata complex. Crop Sci. 56:1146-1156. doi:10.2135/cropsci2015.12.0750.
Kenaley SC, Hudler GW, Bergstrom GC. 2016. Detection and phylogenetic relationships of Puccinia emaculata and Uromyces graminicola (Pucciniales) on switchgrass in New York State using rDNA sequence information. Fungal Biology 120: 791–806.
Knowles, R.P., Baron, V.S., McCartney, D.H.: Meadow bromegrass. – Agriculture Canada Publication188/E, Ottawa 1993.
Lamb, J.F.S., D.A. Samac, and C.C. Sheaffer. 1997. Alfalfa variety development. Minnesota agripower project, task II research report. Web. doi:10.2172/621877.
Lee, M.R.F., L.J. Harris, J.M. Moorby, M.O. Humphreys, M.K. Theodorou, J.C. McRae, and N.D. Scollan. 2002. Rumen metabolism and nitrogen flow to the small intestine in steers offered Lolium perenne containing different levels of water-soluble carbohydrate. Animal Science 74:587-596.
Lenne JM. 1990. World list of fungal diseases of tropical pasture species. Phytopathological Papers No. 31. CAB International, Oxon, UK. 162 p.
Li, X. and E.C. Brummer. 2012. Applied genetics and genomics in alfalfa breeding. Agronomy, 2:40-61. doi:10.3390/agronomy2010040.
Li, X., Y. Han, Y. Wei, A. Acharya, A.D. Farmer, J. Ho, M.J. Monteros, and E.C. Brummer. 2014. Development of an alfalfa SNP array and its use to evaluate patterns of population structure and linkage disequilibrium. PLoS One 9:e84329. doi: 10.1371/journal.pone.0084329.
Lowry DB, Behrman KD, Grabowski P, Morris GP, Kiniry JR, Juenger TE. 2014. Adaptations between ecotypes and along environmental gradients in Panicum virgatum. American Naturalist 183: 682–692.
Marley C.L., Cook, R., Barrett, J., Keating, R., and Lampkin N.H. 2006. Veterinary Parasitology 138:280–290.
Marley, C.L., Fraser, M.D., Fychan, R., Theobald, V.J., and Jones, R. 2005. Veterinary Parasitology 131: 267–282
McMillan C. 1964. Ecotypic differentiation within four North American prairie grasses. I. Morphological variation within transplanted community fractions. American Journal of Botany 51: 1119–1128.
Miller, L.A., J.M. Moorby, D.R. Davies, M.O. Humphreys, N.D. Scollan, J.C. McRae, and M.K. Theodorou. 2001. Increased concentration of water-soluble carbohydrate in perennial ryegrass (Lolium perenne L.): milk production from late-lactation dairy cows. Grass and Forage Science 56:383-394.
Mundt CC. 2014. Durable resistance: a key to sustainable management of pathogens and pests. Infection, Genetics and Evolution 27: 446–455.
Parish DJ, Casler MD, Monti A. 2012. The evolution of switchgrass as an energy crop. Pp. 1-28 in A. Monti (ed), Switchgrass, Green Energy and Technology. Springer-Verlag, London, England.
Ramachar P, Cummins G. 1963. The species of Uromyces on the tribe Paniceae. Mycopathologia et Mycologia Applicata 19: 49–61.
Ramachar P, Cummins G. 1965. The species of Puccinia on the tribe Paniceae. Mycopathologia et Mycologia Applicata 25: 7–60.
Riday, H., and E.C. Brummer. 2002. Forage yield heterosis in alfalfa. Crop Sci. 42:716-723.
Riday, H., and S. Wagner. 2012. Improvements in alfalfa subspecies Falcata germplasms and their hybrid performance. Jul. 10-12, Proc. 2012 Joint Conference NAAIC, Trifolium, and Grass Breeders, Ithaca, NY.
Rouquette, F.M., Jr., L.A. Redmon, G.E. Aiken, G.M. Hill, L.E. Sollenberger, and J. Andrae. 2009. ASAS centennial paper: Future needs research and extension forage utilization. J. Anim. Sci. 87:438-446.
Şakiroğlu, M., J.J. Doyle, and E.C. Brummer. 2010. Inferring population structure and genetic diversity of a broad range of wild diploid alfalfa (Medicago sativa L.) accessions using SSR markers. Theor. Appl. Genet. 121:403-415.
Samac, D. A. 2014. The alfalfa research program in USDA-ARS; the USDA-ARS alfalfa roadmap: improving alfalfa for the 21st century farms and markets. North Amer. Alfalfa Improv. Conf. 8-10 2014. Lethbridge, Alberta CAN. http://naaic.org/Meetings/National/2014meeting/NAAIC%20ARS%20research%20abstract%202014.pdf
Sanada, Y., T. Takai, T. Yamada. 2007. Ecotypic variation of water-soluble carbohydrate concentration and winter hardiness in cocksfoot (Dactylis glomerata L.). Euphytica 153:267-280.
Schmer MR, Vogel KP, Mitchell RB, Perrin RK. 2008. Net energy of cellulosic ethanol from switchgrass. Proceedings of the National Academy of Sciences of the United States of America 105: 464–469.
Schrotenboer AC, Allens MS, Malmstrom CM. 2011. Modification of native grasses for biofuel production may increase virus susceptibility. GCB Bioenergy 3: 360–374.
Serba DD, Uppalapati SR, Mukherjee S, Krom N, Tang Y, Mysore KS, Saha MC. 2015. Transcriptome profiling of rust resistance in switchgrass using RNA-seq analysis. The Plant Genome 8.
Stewart A, Cromey M. 2011. Identifying disease threats and management practices for bio-energy crops. Current Opinion in Environmental Sustainability 3: 75–80.
Strange RN, Scott PR. 2005. Plant disease: a threat to global food security. Annual Review of Phytopathology 43: 83–116.
Sykes VR, Allen FL, Mielenz JR, Stewart Jr. CN, Windham MT, Hamilton CY, Rodriguez Jr. M, Yee KL. Reduction of ethanol yield from switchgrass infected with rust caused by Puccinia emaculata. Bioenergy Research 9: 239–247.
Thomsen PM, Brummer EC, Shriver J, Munkvold GP. 2008. Biomass yield reductions in switchgrass due to smut caused by Tilletia maclaganii. Online. Plant Health Progress; http://dx.doi.org/10.1094/php-2008-0317-01-RS
Undersander, D. 2014. United States research update. North Amer. Alfalfa Improv. Conf. 8-10 2014. Lethbridge, Alberta CAN. http://naaic.org/Meetings/National/2014meeting/Presentations/Undersander,%20USResearchUpdate.pdf
Uppalapati SR, Serba DD, Ishiga Y, Szabo LJ, Mittal S, Bhandari HS, Bouton JH, Mysore KS, Saha MC. 2013. Characterization of the rust fungus, Puccinia emaculata, and evaluation of genetic variability for rust resistance in switchgrass populations. Bioenergy Research 6: 458–68.
USDA National Agricultural Statistics. 2013. https://www.nass.usda.gov/Statistics_by_Subject/
Volaire, F., and H. Thomas. 1995. Effects of drought on water relations, mineral uptake, water-soluble carbohydrate accumulation and survival of two contrasting populations of cocksfoot (Dactylis glomerata L.). Annals of Botany 75:513-524.
Waghorn, G.C., McNabb, W.C., 2003. Consequences of plant phenolic compounds for productivity and health of ruminants. Proc. Nutr. Soc. 62, 383–392.
Zale J, Freshour L, Agarwal S, Sorochan J, Ownley BH, Gwinn KD, Castlebury LA. 2008. First report of rust on switchgrass (Panicum virgatum) caused by Puccinia emaculata in Tennessee. Plant Disease 92: 1710.
Zhu Q, Bennetzen JL, Smith SM. 2013. Isolation and diversity analysis of resistance gene homologues from switchgrass. G3: 1031–1042.