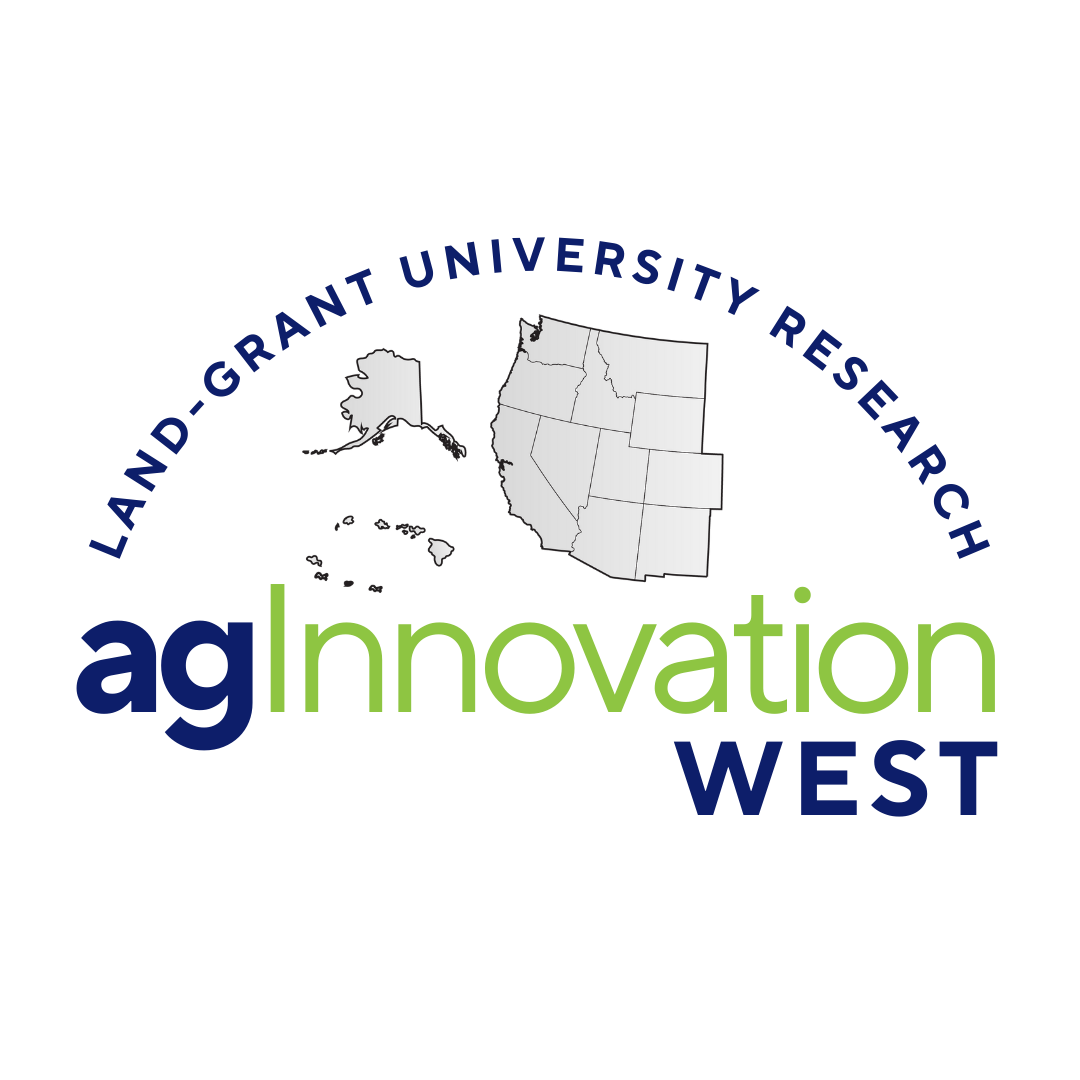
W3170: Beneficial Reuse of Residuals and Reclaimed Water: Impact on Soil Ecosystem and Human Health (formerly W2170)
(Multistate Research Project)
Status: Inactive/Terminating
W3170: Beneficial Reuse of Residuals and Reclaimed Water: Impact on Soil Ecosystem and Human Health (formerly W2170)
Duration: 10/01/2014 to 09/30/2019
Administrative Advisor(s):
NIFA Reps:
Non-Technical Summary
Statement of Issues and Justification
STATEMENT OF ISSUES: Millions of tons of residual by-products, such as urban wastes (biosolids, recycled water, food scraps and other municipal solid waste), agricultural waste (manure) and industrial sludges are produced in the U.S. On average, approximately 50% of biosolids, 98% of food scraps, and 45% of yard trimmings are currently disposed of in landfills or incinerated at substantial cost to the industry and public (King et al., 2011). Reuse of residuals as soil amendments offers the potential to substitute beneficial agronomic and environmental uses for disposal costs. Treated liquid wastes, such as wastewater effluent, recycled water and other non-potable waters, also present opportunities for beneficial reuse in lieu of surface water discharge or expensive treatment. There have been many obstacles to optimized use of residuals. These include a lack of research to optimize residual based product development, conflicting regulations or the absence of regulations on residuals use, and lack of public outreach and communication. There is increasing evidence that land application of a variety of residuals may provide agronomic and environmental benefits that were either not previously well understood and/or that are critical to addressing emerging environmental issues associated with climate change (Brown et al., 2011). The W2170 workgroup propose to continue the investigation of biogeochemical cycling of plant nutrients, the movement of trace element and trace organic contaminants (TOrCs) into the food chain, the potential toxicity of trace elements and TOrCs in residuals to the soil and water ecosystems, and their long-term bioavailability in residual-amended soils to develop additional knowledge to help promote residuals recycling practices that are protective of human health and the environment. These results will provide data for continuing risk assessment required by the USEPA Part 503 Rule for land-application of biosolids as well as for developing regulations for land-based recycling of residuals and reclaimed water. Research will also focus on benefits of reuse including field and watershed scale effects on soil quality, plant drought response, soil carbon sequestration, water quality, greenhouse gas emissions, and climate change impacts associated with soil-based reuse of residuals and reclaimed water. We will also explore the potential for residual based products to be used in urban areas including urban agriculture, restoration, and green stormwater infrastructures. W2170 members are conducting research, on both short- and long-term application sites, whose results will enable the development of guidelines for maximizing the beneficial uses of a considerable variety and number of residual by-products. JUSTIFICATION: The beneficial use of residuals requires both an understanding of potential hazards and value of the constituents in the by-products. Investigation of the fate and behavior of contaminants and nutrients in biosolids amended soils has been the historic focus of research of the W2170 (and its predecessors, W1170 and W170) multistate workgroup. Research conducted previously bu the workgroup formed the basis for the U.S. EPA Part 503 sludge rule, which is one of the few rules to include bioavailability assessments in the development of limits for critical contaminants (National Research Council, 2003). Two subsequent NAS reviews focused on the rule confirmed that scientific basis for this rule and risk assessment were valid (NRC, 2002). We are now faced with determining whether TOrCs merit regulation. Human and ecological risk assessment (HERA) science continues to mature with bioavailability-based risk assessment frameworks being developed and/or considered for implementation in the U.S., Canada, the European Union, Australia and other countries. Research is needed to provide the scientific basis for risk-based methods to evaluate residuals and residual-treated soils for adoption by HERA frameworks. Research needed to evaluate contaminants in residuals includes (i) trace element speciation by advanced spectroscopic methods and wet chemical speciation methods, (ii) field studies to investigate potential plant uptake of frequently detected trace elements and TOrCs in residuals-amended soils, (iii) in vitro methods correlated with human and ecological endpoints, and (iv) novel in vivo methods. Several members of the W2170 were extensively involved in the development of the Part 503 regulation and continue to be involved in the development of risk assessment for other residuals (e.g., the EPA risk assessment for land application of cement kiln dust and foundry sand) and for other pollutants not initially regulated or considered in Part 503 (e.g., barium, TOrCs). The scientific approach used in the development of Part 503 has been applied by this group to an expanded variety of residuals, contaminants and receptors. As the understanding of bioavailability is expanding, the group is broadening its focus to develop linkages between quantitative understanding of the contaminant forms and their bioavailability to a range of receptors. In the past, our group has conducted cooperative projects involving laboratory incubations, greenhouse studies, and x-ray adsorption spectroscopy to elucidate the role of organic and inorganic components of biosolids in binding metals (Brown et al., 2003a; Hettiarachchi et al., 2003; Ryan et al., 2003, 2004a; Scheckel et al., 2004; Hettiarachchi et al., 2006). We have demonstrated that biosolids and other soil amendments can reduce the bioavailability of metals in contaminated systems (e.g., Hettiarachchi and Pierzynski, 2002; Brown et al 2003b; Brown et al., 2007; DeVolder et al., 2003; Basta et al., 2001), while developing various extractants to assess bioavailability of mineral and bioavailable fractions of inorganic contaminants (e.g., Rodriguez et al., 2003; Basta et al., 2003; Schroder et al., 2003; Brown et al., 2004; Ryan et al., 2004). As the potential for metals to affect a range of receptors is more fully understood, research has broadened to encompass a range of measurement endpoints. The goal of this research is to evaluate functions of the restored ecosystem utilizing tools such as in vivo and in vitro assays, toxicity assays, and measures of microbial community dynamics (Alexander et al., 2003; Basta et al., 2003; Schroder et al., 2003; Brown et al., 2004b). As a result of cooperative research conducted by members of W2170, alternative in situ remedial options have been included on a number of EPA Superfund National Priority List (NPL) sites. These include use of biosolids to rehabilitate metal-contaminated ecosystems. The tools developed for this research have also been applied to gain a fuller understanding of the functioning of biosolids-amended soils. The sustainability of biosolids application to agricultural lands has been demonstrated by evaluating the effect of biosolids application on soil function. Potential receptors have included earthworms and soil microorganisms. While important initial research has been done in this area and implications of this research are being recognized in the remediation of contaminated sites, this type of work is still at a developmental state. The multistate research group will continue research to improve our understanding of this field. Another area of concern is the fate of residuals-borne trace organic chemicals (TOrCs) in soils. Classes of TOrCs include estrogenic compounds, personal care products, and pharmaceuticals (Topp and Clucci, 2004; Xia and Pillar, 2004). A wide range of TOrCs in streams in the vicinity of wastewater treatment plants and confined animal feeding operations has been frequently reported. The fate and persistence of these compounds during biosolids stabilization processes or following land application of residuals and non-potable water is not fully understood. Persistence in soils and potential for ecosystem effects as result of land application of residuals and non-potable water containing these micro-constituents is a critical area requiring additional research. Impending water shortages are motivating renewed interest in the use of organic residuals and the intentional reuse of degraded waters (OConnor et al, 2008). Soil application of organic amendments for field crop production may have an ameliorative effect on drought-stressed crops. Heckman et al. (1987) found that field grown soybeans fertilized with biosolids had increased drought resistance and nitrogen fixation than the control treatment. Zhang et al. (2005) determined that biosolids subjected to various treatment processes enhanced endogenous antioxidant enzyme activity, photochemical efficiency, and drought resistance of tall fescue. Cogger et al. (2012) showed increased yields in dry land wheat fertilized with biosolids in comparison to synthetic fertilizer. This increase may be the result of increased soil water holding capacity in the biosolids amended soils (Brown et al., 2011). Fresh water sources for irrigation are limited. While the application of treated or partially treated wastewater effluents to cropped and forested lands has long been practiced, other sources of degraded water (stormwater, irrigation return flow, gray water, and concentrated animal feeding operations [CAFO] effluents) are available to meet anticipated shortfalls. Since many of the major reuse opportunities involve water applications to soil systems (e.g., irrigation), addressing the benefits, risks, and sustainability of degraded water reuse logically fits within the scope of the proposed research project. Some of the greatest challenges to expand the beneficial use of reclaimed water and treated wastewater effluent are concerns associated with TOrCs possessing endocrine disrupting activity (Miller, 2006). Many pharmaceuticals, personal care products, plastics, pesticides and industrial by-products possess such activity. The endocrine disrupting TOrCs are frequently detected in municipal, industrial, and agricultural wastewater but information on their environmental effects and fate in treated soils is limited. Endocrine disrupting compounds (EDCs) interfere with natural hormones causing reproductive and growth problems in animals, particularly aquatic organisms (Xia et al., 2005). A major concern is the ecological impacts at trace concentrations (~1 ng/L) of EDCs in surface waters from wastewater effluents or leachate from residuals-amended soils (Koplin et al., 2004). Soil organic matter increases with long-term irrigation using reclaimed wastewater (Quin and Mecham, 2005; Walker and Lin, 2008), so hydrophobic EDCs retention in soil should be enhanced (Wright et al., 2010). However, some EDCs are hydrophilic and have structures that resist enzymatic attack (Metcalf and Eddy, 2007), and their persistence and impact are less certain. Field research in a turfgrass system at the University of California, Riverside confirmed that these compounds have very limited mobility, even under heavy irrigation (Xu et al., 2009). Nevertheless, there are few published studies on the presence, mobility, and degradability of EDCs at effluent irrigation sites (see Pedersen et al., 2003; Kinney et al., 2006). The impact on groundwater beneath spray irrigation sites has received very limited attention. In summary, research on contaminant bioavailability in both residuals amended soils and in contaminated soils restored with residuals is incomplete. New ecological endpoints must be investigated to improve risk assessment to ensure human health and environmental safety. Research is also needed to maximize yields with the the use of residuals and reclaimed wastewater. Both offer potential alternatives to current, sometimes environmentally degrading, agricultural and land restoration practices. The experience and expertise of W-2170 members in addressing these issues is widely recognized and is well positioned for continued success. The W-2170 membership offers the advantage of including institutions and entities (universities, USDA-ARS, USEPA, municipal government utilities) from across the entire United States. Such collaboration enables discoveries to account for widespread differences in climate, soils and types and sources of residuals, whose fate, transport, and impact will vary across these factors.
Related, Current and Previous Work
Objective 1. Evaluate the short- and long-term chemistry and bioavailability of nutrients and potentially toxic inorganic trace elements, and pharmaceuticals and personal care products (TOrCs) in residuals, reclaimed water, and amended soils to assess the environmental and human health risk-based effects of their application at a watershed scale. Early work on the effect of residuals application to agricultural or undisturbed soils focused on changes in total metal concentrations and plant available metal concentrations, as measured with traditional methods. Our understanding of ecosystem function and nutrient cycling has become increasingly sophisticated and shown that additional measures, using more sophisticated methods and a wider range of endpoints are needed. Bioavailability has traditionally been measured through plant assays or soil extractions. These extractions provide information on operationally defined fractions of metals in soils. Scheckel et al. (2003) showed that extractions, when used to evaluate in situ soil amendments, do not provide a quantitative understanding of the speciation of contaminants in soils. More recent research has focused on the use of x-ray adsorption spectroscopy to both define mineral form of the contaminants of concern and to link this information to measures of bioavailability (DAmore et al., 2005; Ryan et al., 2004b; Devolder et al., 2003, Brown et al., 2012). Recently, advanced spectroscopic methods in combination with sequential soil extractions have been used to determine chemical P species and kinetics and mechanisms of P release in biosolids-amended soils (Peak et al., 2012, Kar et al., 2011). Spectroscopy And Bioavailability Further research is needed to develop and evaluate advanced spectroscopic methods to predict the long-term bioavailability and toxicity of trace elements in residuals and residual-amended soils. Our ability to measure the form of a contaminant in soil may provide a more accurate assessment of the long-term fate and bioavailability of contaminants in amended soils. Prior research has focused on the plant uptake pathway (e.g., Basta et al., 2005), but there has been limited research on alternate exposure pathways in HERA for residual-treated soils. The research gaps on trace element bioavailability needed for a HERA framework are (i) development and application of methods to evaluate the soil ingestion (i.e. human and ecological) exposure pathway, (ii) development and evaluation of methods used to evaluate trace element bioavailability to ecological receptors and risk-based ecological endpoints, and (iii) development and application of advanced solid phase spectroscopic methods. Incidental ingestion is an important exposure pathway for assessing human health exposure and risk associated with trace-element contaminated soils. The bioavailability of Pb, As, and other soil contaminants can be determined by conducting dosing trials using acceptable surrogate animal models. However, to overcome the difficulty and expense associated with in vivo dosing trials, in vitro gastrointestinal (IVG) methods have been developed that simulate human gastrointestinal conditions. The IVG method may be an inexpensive and useful method to determine trace element bioaccessibility in residuals containing low pollutant concentrations. Unfortunately, there is no information evaluating the ability of IVG methods as a method to evaluate soil ingestion in a HERA framework for residuals (Juhasz and Basta, 2013). In order to define cleanup and remediation standards for contaminated sites, site-specific data is required to ensure an accurate assessment of potential environmental and human health risk. Relative bioavailability (RBA), a type of IVG, is a measure of the amount of the contaminant that is absorbed by a receptor as a result of contaminated soil exposure (i.e. incidental ingestion of soil). It is dependent on mineralogy, soil properties and the residence time of the contaminant in the soil (Ruby et al. 1999). RBA is often less than the assumed 100%. This may have a significant influence on human exposure and risk assessment. Adjustments to RBA may be achieved by conducting in vivo bioavailability or in vitro bioaccessibility studies. Currently, in vivo assays (e.g. swine, primate, rodent) are the method of choice, however, these assays are complicated, expensive and time consuming (Juhasz and Basta, 2013). To overcome the difficulty and expense associated with in vivo trials, research has been directed toward the development of in vitro methods to simulate human GI conditions and predict bioavailable Pb. Over the past 2 decades, a limited number of studies have established the relationship between in vivo RBA and in vitro bioaccessibility for As, Cd, and Pb (Basta et al. 2007; Denys et al., 2012; Hettiarachchi et al., 2003; Juhasz et al. 2009; Rodriguez et al. 1999; Ryan et al., 2004; Smith et al., 2011). Currently, the Relative Bioaccessibility Leaching Procedure (RBALP) developed by Drexler and Brattin (2007) is accepted by EPA use in human health risk assessment (USEPA, 2007b). However, the viability for this test for sites outside of the CERCLA or NPL programs has not been demonstrated. Recently, Basta (2013) reported that RBALP did not predict reductions in bioavailable Pb for soil treatments previously shown to reduce bioavailable Pb (Scheckel et al., 2009). This is a serious obstacle because in situ remediation methods cannot be evaluated without an accurate in vitro method to access their ability to reduce Pb bioavailability and human exposure. The research objective of the projects specific task is to develop and evaluate in vitro (including chemical speciation) and novel in vivo methods to correlate human and ecological health responses with risk-based bioavailability of trace elements in residuals and residual-treated soils Trace Organic Chemicals (TOrCs) There are concerns on the fate of residuals-borne trace organic chemicals (TOrCs) in soils. Classes of TOrCs include estrogenic compounds, personal care products, and pharmaceuticals (Topp and Clucci, 2004; Xia and Pillar, 2004). Presence of a wide range of TOrCs in streams in the vicinity of wastewater treatment plants and confined animal feeding operations has been frequently reported (Koplin et al., 2004; Kinney et al 2006; Stumpf et al.,1999). The fate and persistence of these compounds during biosolids stabilization processes or following land application of residuals and non-potable water is not known. Persistence in soils and potential for ecosystem effects as result of land application of residuals and non-potable water containing these micro-constituents will require research. Most of the research emphasis on TOrCs has been on their presence in effluent-impacted surface waters (Koplin et al., 2004; Kinney et al.,2006). There has also been research on impact of TOrCs in soil systems. TOrCs can be added to soils via irrigation of non potatble water, manures and biosolids. However, the following questions remain un-answered: 1) Can biosolids/manures provide unique micro-sites to enhance or hinder abiotic and biotic degradation of TOrCs within biosolids matrixes; 2) How do TOrCs partitioned in manures/biosolids diffuse out of biosolids/manures matrixes into the soil after application and what are the environmental factors affecting this process; and 3) During amendment decomposition process, can amendment-partitioned TOrCs be co-transformed or are they simply released to the soil? To date, many published studies on the environmental fate and transport of TOrCs were conducted with compounds added directly to soil outside of the amendment matrix (USEPA, 1998; Kreuzig et al., 2003; Jones et al., 2005; Richter et al., 2007). It has become evident that soil only systems for assessing fate and transport of TOrCs are not appropriate due to the following reasons: 1) many TOrCs are closely associated with biosolids/manures and enter the soil system in amendment-partitioned states 2) the physical, chemical, and biological behavior of residual-partitioned TOrCs are not well understood but are expected to be different from compound directly applied to soils; and 3) biosolids/manure can alter the physical, chemical, and biological properties of a soil, all of which can have significant impact on environmental fate of TOrCs in a soil. Hence, it is important to establish standard guidelines that include amendment-impacted soil systems in laboratory experiments that assess environmental fate and transport of TOrCs. A recent study by Walters et al. (2010) on transformation of 14 biosolids-partitioned TOrCs demonstrated discrepancies between model predicted half-lives based on laboratory studies of soil only systems and those in a biosolids-amended soil system. This study also showed a lack of correlation between log Kow of a compound and its degradation half-life. For example, Azithromycin which is soluble in water and has log Kow of 0.14 is more persistent than Triclosan which is highly hydrophobic (log Kow=4.22). Although strong evidence has suggested biosolids amendment could increase the persistence of TCS and TCC as well as other TOrCs in certain soils, the mechanisms of this effect remain unclear. In addition, it is also unknown to what extent that amendment of different types of biosolids could affect the persistence of TOrCs in a wider range of soils. Antibiotic Resistance There is currently a concern that land application of biosolids could be contributing to the development and dissemination of resistance to antibiotics of clinical importance (Finley et al. 2013; Gaze et al., 2013). The potential human health risks from this potential phenomenon are unknown, and need to be considered within a risk assessment framework (Ashbolt et al. 2013). In the UK, the abundance of integrons associated with resistance to antibiotics and biocides was increased in soils receiving biosolids compared to unamended soils (Gaze et al. 2011). Some antibiotic resistance genes were enriched in Michigan soils treated with biosolids (Munir and Xagoraraki 2011). Overall, there is very little information and much uncertainty about the soil fate of antibiotic resistance genes carried in organic amendments, their mobility (horizontal transfer) into members of the natural soil flora, their potential for contaminating crops grown in biosolids-fertilized ground, and their significance within the overall problem of antibiotic resistance. Objective 2. Evaluate the uses and associated agronomic and environmental benefits for residuals in agricultural and urban systems. Soil Fertility, Tilth And Biosolids Understanding the rate of conversion of biosolids organic N to ammonium and nitrate (mineralization) and volatilization loss via ammonia is important to ensure that biosolids rates are efficiently applied for growth or vegetation with reduced impacts on water quality. Biosolids mineralization is primarily dependent on the soil environment and the type of biosolids (Smith et al., 1998). Other factors that affect mineralization rate of organic N include the method of biosolids application (Cripps et al., 1992; Cartron and Weil, 1998). Gilmour (1998) found that surface application likely slows decomposition and attendant N mineralization rates of the organic N pool. By contrast Li et al. (2013) determined that the N availability of lime stabilized and anaerobically digested biosolids were similar whether the residuals were incorporated by disking into soils or remained on the surface of no-till soils in Virginia. The effect of incorporation requires additional work as no-till practices are being increasing adopted for lands where biosolids are applied. There is research currently being conducted in Virginia (Evanylo) and Colorado (Barbarick) to refine biosolids application timing and rates on soils of varying texture to minimize N leaching in corn-wheat-soybean rotations. Colorado State University researchers (Barbarick et al, 2010, 2012) determined that the long term application of biosolids to dryland, no-till wheat-fallow and wheat-corn-fallow agro-ecosystems provided ample N and improved soil moisture retention and vegetative cover that reduced erosion. Biosolids and N fertilizer produced similar wheat and corn yields, indicating that biosolids PAN was not limiting. Virginia Tech researchers (Li et al., 2013) demonstrated beneficial effects of biosolids on drought-amelioration, C sequestration and N availability in a corn-soybean rotation under both conventional and no-tillage practices on coarse-textured soils from 2009-2011. Land application of biosolids has been shown to result in substantial increase in soil organic matter content (Tian et al., 2009, Brown et al., 2011, Spargo et al. 2008; Rostagno and Sosebee, 2001; Moffet et al., 2005; Wallace, 2008). For example, Five years after application of biosolids to a silt loam at 60 dry Mg ha1 the organic C contents in different soil size fractions were more than 3 times as much as that in the control soil (Wallace, 2008). In a separate study, the soil organic matter content was 1.5 times of that in the control soil for a clay loam soil receiving biosolids application at 50 dry Mg ha1 year-1 for 3 years (Tsadilas et al., 2005). As a result of increased soil organic matter content, soil physical properties such as the number and size of soil aggregates and their stability, porosity, hydraulic conductivity, and soil water holding capacity can be significantly enhanced. Increased soil aggregation tends to enhance soil porosity and, therefore, soil hydraulic conductivity (Tsadilas et al., 2005). Potentially as a result of improvement in soil physical properties and other factors, soil application of organic amendments for field crop production may have an ameliorative effect on drought-stressed crops. Sahs and Lesoing (1985) observed higher sweet corn yields in plots amended with beef feedlot manure than those that were inorganically fertilized during drought years. Heckman et al. (1987) found that field grown soybeans fertilized with biosolids had increased drought resistance and nitrogen fixation than the control treatment. Zhang et al. (2005) determined that biosolids subjected to various treatment processes enhanced endogenous antioxidant enzyme activity, photochemical efficiency, and drought resistance of tall fescue. As food security and drought tolerance become increasingly important, maximizing benefits associated with the use of biosolids will be a focus of land application research. Biosolids, Soil Health And Climate Change Agriculture and other land uses are both sources of greenhouse gases and sinks for terrestrial carbon sequestration. Deforestation and conventional tillage practices were the largest source of CO2 release to the atmosphere until the 1970s (Lal, 2007). Altering land use practices to minimize oxidation of soil carbon and increase rates of sequestration in soils has the potential to reduce GHG. Different biosolids end use/disposal options have the potential to result in significant greenhouse gas emissions and/ or carbon sequestration. Biosolids are a mixture of short cycle fixed carbon, water and nutrients. If the short cycle carbon decomposes and emits CO2, there is no net impact on the carbon cycle. If, as a result of different end use/disposal options however, a portion of this carbon remains fixed or if the carbon is emitted as CH4, the end impact of biosolids on the carbon cycle becomes either positive or negative (Bolan et al., 2013; Brown et al., 2012; Thangarajan et al., 2013; Tian et al., 2009). Using nutrients in biosolids as substitutes for synthetic fertilizer will have an impact on the carbon cycle based on the avoidance of fossil fuel use from manufacturing synthetic fertilizer. On the other hand, if the N in biosolids is returned to the atmosphere as N2O as a result of biosolids end use/disposal practices a significant GHG debit will be accrued (Brown et al., 2010; Thankgarajan et al., 2013). The GHG balance for different biosolids end use/disposal practices was evaluated (Brown et al., 2011). Anaerobic digestion plus land application was the overall best practice for GHG emissions and resulted in net GHG credits. In contrast, combustion, particularly at lower temperatures resulted in significant emissions as a result of the formation of N2O. Higher burn temperatures eliminate N2O formation but result in increased NOx emissions. Understanding the factors involved in transformation nitrogen that lead to release of N2O, and understanding how to minimize losses of N as N2O also has potential to reduce GHGs. Nitrogen availability in biosolids and other organic by-products will vary as a function of composition and processing technologies, as well as soil types and climates. Research is necessary to quantify N2O release from organic residual-amended soils under a range of soil types, land management practices and climatic conditions. The Intergovernmental Panel on Climate Change recognized the role of municipal biosolids and manures to substitute for synthetic fertilizers as well as a source of carbon in these systems (IPCC, 1996). Castillo et al. (2010), Dere and Stehouwer (2011), and Dere et al. (2011, 2012) replaced synthetic fertilizers with biosolids and manures for the cultivation of bioenergy crops. Our understanding of the role of soils in global climate change presents new opportunities and challenges for residuals management. Such by-products can be both a tool for increased carbon sequestration (via soil carbon additions and displacement of synthetic fertilizers) and a source of GHG emissions (via N2O emissions following land application). Urban Uses For Biosolids Markets for land application of biosolids have traditionally been identified well outside of urban boundaries. In fact agronomic or restoration end use options account for the vast majority of biosolids beneficial end use projects in the US (NEBRA, 2007). However, there is a significant potential for use of biosolids based products in urban areas. Use of biosolids in urban environments will likely be limited to materials that meet Class A pathogen destruction criteria. There are a wide range of potential end uses for different products. Examples include use of Class A materials for turf grass fertilization, urban agriculture, urban tree planting and forestry, and green stormwater infrastructure. Research is necessary to develop biosolids based products that are suitable for use for each of these cases. It is likely that urban customers will be more amendable to using a biosolids based product that has been tailored for particular end uses. Urban Soil Improvement Soils in urban landscapes are generally highly disturbed and heterogeneous, with little systematic pattern in their characteristics (Pouyat et al., 2010). The urban soil generally lacks the organic matter cycling and nutrient contribution that typifies the soil of the natural ecosystem. The soil organism population is limited, and the activity is reduced below natural soils levels. Biosolids can be valuable amendments for disturbed, urban soil by imparting beneficial effects that can improve soil physical, chemical, and biological properties and providing sources of nutrients for improving plant biomass. Biosolids can be utilized to improve soil physical, chemical, and biological properties of soils (Metzger and Yaron, 1987). Improved physical properties include reducing bulk density (Punshon et al., 2002), increasing water-holding capacity (Punshon et al., 2002), and improving pore size distribution (Pagliai and Antisari, 1993; Kirchmann and Gerzabek, 2002). Improved chemical properties include increasing cation exchange capacity and organic matter (Stoffella et al., 2003; Ozores-Hampton et al., 2011). Improved biological properties include increasing the diversity and amount of microbial communities and soil fauna, and increasing aboveground plant biomass yield (Sydnor and Redente, 2002). Schnell et al. (2010) showed that incorporating composted municipal biosolids (CMB) for the enhancement of soil physical properties and turfgrass sod establishment provided the best option for achieving benefits of CMB while reducing nutrient runoff loss. Vietor et al. (2010) found that composted biosolids can enhance turfgrass establishment and soil properties, but nonpoint-source runoff losses can occur during the production; however, incorporation of alum effectively reduced soluble-reactive P runoff from CB, soil, and turfgrass sources during turfgrass establishment. Tree growth response for urban trees planted in biosolids amended soils has also been reported (Scharenbroch et al., 2013). Low Impact Development (LID) Removal of vegetation and creation of impervious surfaces due to urban development cause flooding; streambank erosion; impairment of water quality by sediment, nutrients, and heavy metals; and a decline in aquatic biota (National Research Council, 2008). State regulations promote Low Impact Development (LID), a holistic set of practices which seek to restore natural hydrology and water quality. Bioretention is an important LID treatment practice. A bioretention cell consists of a depression in the landscape that includes a vegetated layer, a mulch layer, a filter media (engineered soil mix of sand, soil, and organic matter) and, often, an underdrain and internal storage reservoirVegetated filtration systems with a anaerobic internal storage have been shown to promote high level N removal through denitrification and/or immobilization. Bioretention systems have been shown to reduce runoff volume, total suspended sediment (TSS), and total phosphorus (TP); however, others (Davis et al., 2009) have found them less effective for nitrogen. Design specifications for bioretention vary widely throughout the U.S. There has been little research performed on optimizing recipes for the performance of bioretention systems (Davis et al., 2009). The regulations for several states (VA, WA) greatly limit the type, amount, and source of organic matter in bioretention systems. Use of biosolids- and manure-based composts are often prohibited based on the expectation that they will leach excess nutrients and metals. Design specifications in VA and WA may be neither scientifically defensible nor protective of water quality, and the scientific basis and validity of current Virginia Department of Conservation and Recreation bioretention specifications (VDCR, 2011) have been questioned by Virginia Tech researchers. Considering the anticipated demand for bioretention systems, there is an urgent need to investigate bioretention media specifications and provide improved guidance for media design. Improving media specifications and communicating these results to stakeholders is critical to meeting state water quality goals. Due to the complex processes within a bioretention cell, it is nearly impossible to assess individual factors effecting performance. Mesocosm experiments are valuable because they simplify a complex full scale system, and individual factors can be evaluated through replication. By mimicking the common functions within bioretention systems in controlled conditions, key processes can be isolated for detailed investigation. Hsieh and Davis (2005), performing experiments with mesocosms employing synthetic runoff, showed 96% removal for TSS, 70% for TP; 9% for nitrate and 20% for ammonium. Both cell depth and media filtration significantly contributed to particulate capture, which had the effect of decreasing hydraulic conductivity. The retention and removal of nutrients in bioretention mesocosms were also investigated by Lucas and Greenway (2008). These mesocosms used different combinations of sandy loam, loamy sand and pea gravel so media mixes could be compared. A vegetated group with shrubs/grasses and a non-vegetated control group were also compared to evaluate the effects of plants. The observation demonstrated the vegetated loam mesocosms had higher nutrient retention. The increase in TN retention by vegetated systems also exceeded plants nitrogen uptake rates, suggesting that denitrification conditions were present in the media. The addition of water treatment residuals (WTRs) provided >94% TP retention (Lucas and Greenway, 2011). Researchers (Brown) from the University of Washington (WA) are determining if the phosphorus saturation index (PSI) is a suitable indicator for evaluation of compost mixtures for stormwater bioretention systems. In preliminary studies, Brown found that the PSI predicted leaching of total and dissolved P through bioretention media that included compost from biosolids, yard, food waste, and animal manure amended with various proportions of Fe WTRs. All mixtures reduced transport of total and dissolved Zn and Cu. Researchers from Virginia Tech (Evanylo, Daniels) are assessing media recipes for their capabilities to remove N, P, and heavy metals. Understanding the quality of C required to promote denitrification and properties of potentially P-sorbing constituents are critical for designing effective specifications. There is much work needed to assess bioretention components to ensure that regulatory design specifications are scientifically defensible. Life Cycle Assessment (LCA) The impact of different end uses of biosolids has traditionally been investigated using a soil science lens. The majority of these studies have concluded that biosolids, when used in accordance with regulatory guidelines are a beneficial soil amendment. Use of biosolids has been associated with increased crop yields, improved soil physical properties and enhanced soil carbon sequestration. This suggests that research can continue in a somewhat different vein: to understand the impact of different biosolids end use options from a broader perspective. Life cycle assessment is one tool that is frequently used to provide this type of perspective. A life cycle assessment of biosolids end use/disposal options focused on greenhouse gas emissions showed that different end use options of biosolids could result in significant carbon savings per dry ton. In contrast, disposal of biosolids typically resulted in significant CO2 emissions (Brown et al., 2010). Taking this type of investigation further, a recent study examined use of biosolids in the context of land use planning (Trlica and Brown, 2013). Here researchers looked at the carbon balance of degraded land either restored to forest with the application of biosolids or restored to a low- density subdivision. While construction of homes resulted in significant GHG emissions, restoration to forestry resulted in sequestration of more than 400 Mg CO2 per ha. Tools such as LCA have the potential to provide biosolids program managers with results that are easy to communicate within a municipal infrastructure. As wastewater treatment systems transition to resource recovery centers, this approach becomes even more critical (Brown, 2012; Miller, 2012). It is also an important component of sustainability assessments (Amajirionwu et al., 2008). These are tools that have been used as a way to engage the public in decision making re wastewater treatment and provide for a more nuanced discussion with the possibility of rational public participation (Goven and Langer, 2009). RELATED REGIONAL RESEARCH PROJECTS The following projects are the most closely related to our proposed project. Sewage Sludge, Manure And Other Residuals As Soil Amendments NCERA103: Specialized Soil Amendments and Products, Growth Stimulants and Soil Fertility Management Programs Contaminants/Nutrients WERA-103: Nutrient Management and Water Quality SERA017: Organization to Minimize Nutrient Loss from the Landscape functions as a resource for research and extension on issues related to agricultural management that affect nutrients, runoff, conservation and water quality. Same issues apply to land application of residuals and of great interest to W2170 members.
Objectives
-
Evaluate the short- and long-term chemistry and bioavailability of nutrients, potentially toxic inorganic trace elements, and pharmaceuticals and personal care products (TOrCs) in residuals, reclaimed water, and amended soils in order to assess the environmental and health risk-based effects of their application at a watershed scale. Specific tasks: (i) To develop and evaluate in vitro (including chemical speciation) and novel in vivo methods to correlate human and ecological health responses with risk-based bioavailability of trace elements and TOrCs in residuals and residual-treated soils. (ii) Predict the long-term bioavailability and toxicity of trace elements and TOrCs in residual-amended urban, agricultural and contaminated soils. (iii) Evaluate long-term effects of residuals application and reclaimed wastewater irrigation on fate and transport of nutrients, trace elements, TOrCs, and emergence/spread of antibiotic resistance in high application rate systems. (iv) Evaluate plant uptake and ecological effects of potentially toxic trace elements and TOrCs from soils amended with residuals and reclaimed wastewater.
-
Evaluate the uses and associated agronomic and environmental benefits for residuals in agricultural and urban systems. Specific tasks: (i) Evaluate the ability of in situ treatment of contaminated soil with residuals to reduce chemical contaminant bioavailability and toxicity. (ii) Determine the climate change impacts of organic residuals end use options (i.e., C sequestration, N2O emissions). (iii) Quantify sustainability impacts such as water quality (reduced N impairment) and quantity benefits (increased plant available water, increased drought tolerance) and soil quality improvements associated with a range of organic residuals end uses. (iv) Explore the potential for waste by-products to be used in urban areas including urban agriculture, stormwater infrastructure, green roofs, and in urban green space. (v) Evaluate ecosystem services of degraded urban soils amended with residuals. (vi) Use tools such as life cycle assessment to understand and compare the impacts of a range of residuals end use/disposal options.
Methods
Objective 1. Evaluate the short- and long-term chemistry and bioavailability of nutrients and potentially toxic inorganic trace elements, and pharmaceuticals and personal care products (TOrCs) in residuals, reclaimed water, and amended soils in order to assess the environmental and human health risk-based effects of their application at a watershed scale. We are interested in a science-based understanding of fate of trace inorganic and organic contaminants in residuals and reclaimed waters as well as potential benefits in soils including urban soils, brownfields, Superfund sites, and contaminated sites that do not have such designations. Contaminant reaction products will be positively identified and the reduction in contaminant bioavailability to plants and humans will be accurately estimated. This will be accomplished speciation studies, in vitro and in vivo studies. Studies will be conducted in laboratory, greenhouse and field settings. Outcomes of this project will improve our mechanistic understanding of transformation of contaminants in residuals and reclaimed waters in soils; their plant and human bioavailability; and human health risk and exposure associated with contaminants. Our research efforts will identify or develop in vitro gastrointestinal laboratory methods that will accurately predict reductions in contaminant bioavailability in residual treated soil. Collaborators for these tasks will be represented by researchers from CA, CO, FL, IL, IN, KS, OH, UT, VA, WA, USDA, USEPA, and AgriFood Canada. In vitro bioaccessible (IVBA) contaminants will be determined for contaminated soils subjected to in situ remediation treatments using municipal or industrial residuals. In vitro extraction methods will include the Relative Bioavailability Leaching Procedure of Drexler and Brattin (2007) and other published methods that have conducted in vitro-in vivo correlation (IVIVC) studies. In vitro methods that accurately predict bioavailable contaminant will be determined by constructing IVIVC plots for all treated soils. Our project will elucidate the biochemical mechanism responsible for measuring bioavailable species of contaminants of concern by in vitro gastrointestinal extraction from in situ remediated soils. The underlying mechanism responsible for accurate prediction methods will be determined by biogeochemical modeling of the in vitro extraction solutions. The effect of in vitro extraction solution parameters (i.e., glycine concentration, pH, etc.) on contaminant solubility and activity will be determined. Collaborators for these tasks will be represented by researchers from IL, KS, OH, USDA, and USEPA. To investigate the impact of biosolids amendment on transformation of a range of TOrCs with different hydrophobicity in soils of various properties, USEPAs fate, transport and transformation test standard guidelines (USEPA, 1998; USEPA, 2008a, 2008b) will be used for soil only systems and biosolids-soil systems. The TOrCs to be investigated for this project have a wide range of logKow values and water solubility. They had been frequently detected in biosolids at high levels (Kinney et al, 2006; Xia et al., 2010) due to their frequent usages. Surface (0-15 cm) soil samples with no known history of biosolids application will be collected to represent the three soil orders that are recommended in the USEPAs fate, transport and transformation test standard guidelines: Spodosol, Alfisol, and Entisol (USEPA, 1998). To allow robust statistical analysis, five soil series with a wide range of soil properties (pH, CEC, organic matter content, mineral composition, surface area, soil aggregate composition and stability) will be obtained from each soil order. The properties of each soil will be determined using standard soil testing methods (James et al., 1990). Biosolids from two different sources will be used for this project. The physical and chemical properties of each biosolids sample will be characterized using methods by Mao and coworkers (2003). Concentrations of the target TOrCs in the two biosolids will be analyzed using EPA methods 1694 and 1614 (USEPA, 2007a, 2007b). For the batch and incubation experiments, biosolids will be mixed with each soil sample at a ratio to simulate biosolids land applied soils, which is equivalent to an application rate of about 8-10 Mg/ha (dry weight basis, mean application rate for agronomic crops), assuming that biosolids are mixed with the top 15 cm of soil with a bulk density of 1.4 g cm-2. The final concentration of target TOrCs in the biosolids-soil mixture will be calculated. To understand the major mechanisms determining transformation and bioavailability of biosolids-borne TOrCs in various soil systems, the following activities are proposed: 1) characterization of micromorphology biosolid aggregates using optical microscopy; 2) investigation of the concentration and bioavailability distribution pattern of TOrCs within biosolids aggregates; 3) investigation of the rate of PPCP diffusion out of biosolid aggregates; 4) characterization of PPCP co-transformation and/or release at different biosolids decomposition stage. Biosolids aggregates with different sizes will be randomly collected from the field 1-2 days after biosolids surface application and 1, 3, 6, 12, 24, and 36 months thereafter or until no visible biosolids aggregates are observed on the soil surface. Aggregates collected at each sampling time will be subjected to the proposed activities 1 and 2. Activities 3 and 4 will only apply to the aggregates from the first collection. Collected aggregates will be stored at 2°C in refrigerator and processed within a week. Collaborators for the tasks addressing TorCs will be represented by researchers from CA, CO, FL, IL, IN, OH, PA, UT, VA, WA, USEPA and Agrifood Canada. OUTPUTS Outcomes of this objective will improve our mechanistic understanding of transformation of contaminants in residuals and reclaimed waters; residual application induced transformation of soil contaminants on plant and human bioavailability; and human health risk and exposure associated with land application of residuals and reclaimed waters. Thorough mechanistic understanding and knowledge gathered at tested sites would be applicable to many other sites. The proposed project will enhance the efficacy of using residuals as soil amendments thereof on stabilization of contaminated and/or degraded urban soils, brownfields and Superfund soils. The proposed study will also identify and/or develop an in vitro gastrointestinal test method able to predict bioavailability of trace inorganics in residue amended soils/residue amended contaminated soils. Due to their simplicity, speed and affordability, in vitro assays that simulate conditions in the gastrointestinal tract is an attractive alternative for predicting the ability of in situ treatment of contaminated soil to reduce contaminant relative bioavailability (RBA). We will identify and validate in vitro methods for use and remove regulatory barriers for acceptance and implementation of new in situ soil remediation technologies using industrial and municipal residuals. Higher sorption coefficients and lower desorption coefficients for all six tested TOrCs are expected in biosolids amended soil than soil only. Similar observation would be expected for the transformation rates of the target TOrCs. However, the degree of the difference between the biosolids-soil and soil only systems depends on the properties of a soil and the biosolids. This study will provide, for the first time, micromorphological information on biosolid aggregates. Vastly variable micromorphology would be expected for each individual biosolid aggregate and for biosolid aggregates from different sources (e.g. aerobically and anaerobically digested biosolids). The TOrCs concentration and bioavailability distribution pattern are expected to correlate with the microbial profiles within biosolid aggregates. TOrCs with different physico-chemical properties will exhibit different diffusion rates out of a biosolid aggregate. During biosolids aggregates decomposition, biosolids-borne TOrCs are expected to co-transformed as well as released into the surrounding soil environment. The rates of PPCP co-transformation and released are expected to be largely affected by the PPCP concentration distribution and bioavailability within biosolid aggregates, its diffusion rate, and associated microbial community profiles. Laboratory results generated from this study will be used to assess the field observations. The physico-chemical states of TOrCs partitioned in biosolids matrixes largely affect their environmental fate. The decomposition of land applied biosolids will change the physic-chemical states of biosolids-borne TOrCs in the biosolids matrixes. It is also expected that soil property changes due to biosolids application affect the fate of TOrCs, however, the degree of this effect also depends on the physico-chemical properties of TOrCs. IMPACT In order for in vitro assays to be used as a surrogate measurement of contaminant RBA, instead of animal models, the correlation between RBA and bioaccessibility, and the validation of the relationship, is a prerequisite for scientific as well as regulatory acceptance. At present, an in vitro method has not been validated to evaluate reductions in bioavailability from in situ remediation of soil by using residuals. Validation of an in vitro method is an essential requisite for the innovative approaches and technologies where residuals are used for soil remediation. We will identify and validate in vitro methods for use and remove regulatory barriers for acceptance and implementation of new in situ soil remediation technologies using industrial and municipal residuals. To date, many published studies on the environmental fate and transport of trace organic compounds (TOrCs) have been conducted using experimental design of laboratory tests with soil only systems in accordance with standard guidelines that have been established for pesticides. The proposed comprehensive laboratory and field investigation will generate systematic and mechanistic understanding of fate and transport of biosolids-borne TOrCs in biosolids-impacted soil systems. This research will obtain, for the first time, information on micro-scale physico-chemical states, transformation, and bioavailability of TOrCs within biosolids matrixes. The information generated will be utilized to better understand, at macro- and field-scale the environmental behavior of biosolids-borne TOrCs in a wide range biosolids-soil systems. Ultimately, knowledge gained from this study will help environmental engineers design better biosolids land management systems to minimize environmental impact of biosolids-borne TOrCs. Research findings will be conveyed to state and local regulators, decision makers, farmers, waste and land managers, extension educators, and citizens via various communication outlets. Results from this work can be used by decision-makers, researchers, and engineers to develop or modify regulations and biosolids management strategies to minimize negative environmental impact of TOrCs. Methods Objective 2. Evaluate the uses and associated agronomic and environmental benefits for residuals in agricultural and urban systems. Growing food in urban areas is gaining popularity but contaminants such as trace elements and or TOrCs are commonly found in urban soils. We are interested in science-based approaches to evaluate soil contaminant impact on health and environment and in situ remediation of trace elements in contaminated soils and using residuals to reduce bioavailability and toxicity of commonly found contaminants. On-going research projects at Kansas State University (KS), USEPA-ORD, University of Washington (WA), Ohio State University (OH), Virginia Tech (VA), and MWRD (IL) are currently testing several different combinations of inorganic and organic amendments to effectively stabilize both Pb and As in soils at several urban sites. These research efforts will improve fundamental understanding and permanence/longevity of contaminant stabilization in soil upon in situ residual treatment additions. We are planning to use different urban Superfund and brownfields sites representing a range of Pb levels in the presence or absence of co-contaminants such as As. Understanding the effect and behavior of co- contaminants that exhibit different chemical behavior than Pb are essential for devising methods for in situ stabilization of soil Pb and immobilization co-contaminants simultaneously. Laboratory, greenhouse and field research designed to assess the ability of residuals to reduce contaminant bioavailability in Brownfields, Superfund and other contaminated soils will be conducted. Chemical and biological methods used to evaluate reduction in risk from contaminants to human and ecological receptors will be evaluated. Laboratory incubation experiments at KSU (KS), UW (WA), Virginia Tech (VA), and OSU will be conducted on different surface soils (0- 15 cm) collected from selected urban Superfund and brownfields sites to determine the effectiveness of residuals rich in C, P and Fe (for example animal manure, composted biosolids) of reducing Pb and other trace element bioavailability in urban soils. The inclusion of C-rich P sources, such as leafy compost, animal manure and composted biosolids, are particularly important because most urban gardeners are using composted organic materials, and past research has shown mixed results for Pb and other trace element stabilization using C-rich amendments (Farfel et al., 2005; Brown et al., 2004; Attanayake et al. 2013). Following incubation, changes in contaminant chemistry will be measured as described under Objective 1 using direct spectroscopic and indirect wet chemical methods to evaluate treatment effectiveness. Selected treatments based on initial laboratory incubation experiment results will be further tested under greenhouse conditions in the presence of plants. Vegetable crop species and common turf grass species (such as tall fescue) will be used as they would be more relevant to urban sites. Plants will be grown for about eight to twelve weeks after which edible portions or above ground parts of plants will be harvested for analysis. Using recycled organic residuals as soil amendments and nutrient sources may reduce the net energy costs associated with mining and synthesizing commercial fertilizers and methane lost from landfilled residuals. We understand the processes that generate the potent greenhouse gas, N2O, from soil N fractions, but our understanding of the rate that N2O forms from N sources under various management practices is incomplete. Laboratory, greenhouse and field research will assess the effects of residuals and their management on climate change through the production of greenhouse gases. Researchers at University of Washington (WA) and Virginia Tech (VT) will compare greenhouse gas equivalencies using life cycle assessment of the production, transport and land application of synthetic fertilizers with organic amendments. Using both published data and new field data, we will compare the effects of use of synthesized and recycled amendments on soil and plant C sequestration and emission of CO2, CH4, and N2O in agricultural and disturbed (i.e., reclaimed mineland, urban lands) soil systems. Nitrogen is the greatest pollutant of ground and surface waters and, through transformation to N2O, a significant air quality pollutant. While we understand the properties of organic residuals and environmental conditions that influence rate and amount of organic N that becomes plant-available, our abilities to predict plant available N (PAN) are still limited by weather variability. We require better regional-specific PAN coefficients for various crops. To improve N use efficiency, we need to refine our application timing recommendations for soils that vary in water and N transport. Field biosolids-based N research will be conducted to further refine PAN rates and application timing across climatic regions and soil types to optimize N use efficiency and minimize N loss for agronomic (esp., corn, wheat, hay and forages) crops (VA, WA, MN, MRWD). We have observed that crops treated with biosolids grow and yield better than crops grown on similar soils fertilized with synthetic fertilizer. Both increased water-holding capacity and plant biostimulant effects appear to play a role in beneficial response to drought. Field research will be conducted (MWRD, WA, VA) to to assess the effects and practical ramifications of residuals on soil water holding capacity, plant available water, and biologically-induced (i.e., biostimulant) plant drought tolerance in varying geographical regions, climates, soils, and ecosystems (i.e., agriculture, urban lands). We will perform laboratory and greenhouse studies to identify and quantify the mechanisms that elicit these benefits. Organic by-products possess attributes that could improve disturbed urban soils, supply an inexpensive source of plant nutrients, and enhance the value of inorganic residuals employed for low impact development (LID) and stormwater control. We will continue assessing organic by-products from a variety of processes and sources for their nutrient-supply and soil property-enhancing benefits in urban ecosystems. We will investigate the potential to use biosolids composts and biosolids-based soil blends, likely in combination with water treatment residuals, for use in bioretention systems (both mesocosms and full scale) for LID stormwater management projects and for remediating urban soils. Universities and agencies conducting research in these areas include Uniesity of Florida (FL), Kansas State University (KS), Metropolitan Water Reclamation District of Greater Chicago (IL), University of Minnesota (MN), Ohio State University(OH), Penn State University (PA), Virginia Tech (VA), University of Washington (WA), and the USDA-ARS.Measurement of Progress and Results
Outputs
- We will develop and evaluate the success and potential of specific residuals to be used as soil treatment technologies to remediate contaminated soils. The output will be based on scientific methods recognized by USEPA and state regulatory agencies to evaluate human and ecological risk.
- We will generate data to determine the potential climate change benefits (i.e., C sequestration, reduced N2O emissions) of substituting biosolids and other organic residuals for commercial fertilizers for fertilizing agricultural soils and reclaiming disturbed lands.
- The research will refine the model used for calculating PAN in residuals for appropriate application rates in varying climatic regions, soil types, biosolids treatment processes, and agronomic practices (e.g., no-till vs tilled agriculture, application timing).
- Mechanistic understanding of the effect of land-applied organic residuals as biostimulants will validate biological benefits of such residuals beyond soil chemical and physical properties enhancement.
- Understanding the mechanism for organic matter facilitated denitrification in bioretention systems will facilitate incorporation of organic residuals in design specifications for constructing functional urban green infrastructures.
Outcomes or Projected Impacts
- The research results will enable: 1) policy makers and federal, state and local government to implement cost-effective, efficient and sustainable approaches for remediating contaminated soils in urban areas including urban brownfields
- 2) scientists to provide regulators, agronomists, and land managers with improved recommendations for managing N and P in organic residuals to ensure cleaner air and water, while maintaining agriculture productivity
- 3) land managers and waste managers to help mitigate detrimental effects of climate change
- 4) the recommendation of amendment types and rates to enhance the health of degraded urban soils and the people living on them
- 5) development of bioretention design specifications to optimize N, P, and metal removal and promote plant growth.
Milestones
(2015): Generate data from field, greenhouse, and laboratory studies to (a) improve plant available nitrogen (PAN) rates for varying climatic regions, soil types, and biosolids treatment processes, (b) improve understanding of the stability of Fe- and Al-bound P with specialized sorbents, (c) verify the biological effects and benefits of biostimulants in land-applied organic residuals on chemical and physical properties enhancement of the environment.(2016): Generate data from field, greenhouse, and laboratory studies to determine the potential climate change benefits (i.e., C sequestration, reduced N2O emissions) of substituting biosolids and other organic residuals for commercial fertilizers for fertilizing agricultural soils and reclaiming disturbed lands.
(2017): Generate data to (a) improve mechanistic understanding of organic matter facilitated denitrification in bioretention systems, (b) develop design specifications for optimum N, P, and metal removal via bioretention, and (c) predict nutrient availability and C sequestration in organic residuals-amended urban soils (gardens and landscapes).
(2018): Compilation of information for supporting improved recommendations for managing nutrients in land applied organic residuals to ensure cleaner air and water while maintaining agriculture productivity.
(2019): Compilation of information on residuals use practices that affect greenhouse gas (GHG) emissions and soil quality.
Projected Participation
View Appendix E: ParticipationOutreach Plan
The W2170 group has traditionally included members of industry, such as Metropolitan Water Reclamation District of Greater Chicago (MWRDGC). The group maintains close contact with other industrial entities such as the Water Environment Federation, the Northwest Biosolids Management Association, and the National Association of Clean Water Agencies. Through direct participation in conferences and workshops, the research findings of the group are reported to industry cooperators. The W2170 group will continue to publish collaborative research reviews that are available to industry and academic partners. Several of the academic members of the group hold either part or full time extension appointments (FL, MN, PA, VA, WA), and their responsibility to stakeholders involve communication of the research conducted by the W2170 group. We will continue to convey an understanding of the science and technical solutions to ameliorate potential human health and environmental concerns through publication of extension bulletins, conducting of field demonstrations and wokshops, and direct contact with stakeholders, clientele and regulators. Members of the group also publish their findings in scientific journals and present results at national and international meetings. The W2170 group also includes members of government agencies, such as USEPA and USDA. As the nature of the research that forms the basis for the W2170 group is generally applied, communication of this work to stakeholders is a fundamental component of the groups mission. Members of the group will continue to participate in training sessions organized by local wastewater treatment agencies to provide information to members on important scientific issues such as N and P dynamics in land application of biosolids. Additionally regional and national level outreach activities will be organized during our group annual meetings as well as national/international conferences such as SSSA, ACS, SETAC Meetings.
Organization/Governance
The W2170 technical committee will consist of project leaders for the contributing states, the administrative advisor, and CSREES representatives. Voting membership includes all persons with contributing projects; however, only one vote is permitted for each research location. Co-chairpersons, one from the western and one from the other regions, will be elected at the first authorized committee meeting after the renewal project has been accepted. The co-chairpersons will serve multiple years, if so desired by project participants, and will be responsible for meeting arrangements, the annual reports, coordination of research activities, compilation of regional research data, and preparation of the renewal proposal. One representative each from the western and the other regions and a secretary will be elected annually. Office terms for annually elected positions begin immediately after completion of the annual committee meeting. An Executive Committee, consisting of the co-chairs, the secretary, and the two regional representatives, will serve as a guidance body in matters such as new project participant additions and meeting agenda. In addition to the official project representatives, other researchers with activities that contribute to the project have been and will continue to be invited to participate on a regular basis. These include, among others: Drs. Brobst and Scheckel from USEPA, Drs. Hundal and Kumar from the MWRDGC, Dr. Chris Higgins from Colorado School of Mines, and Dr. Topp from Agriculture and Agri-Food Canada. Representatives of other regional research and coordinating committees, state and federal agencies, and other federations and organizations are invited to attend the annual meetings and will be sent W2170 annual reports. SIGNATURES: Regional Project Title: Soil-based Reuse of Residuals and Water W-2170 Co-Chair Date W-2170 Co-Chair Date Administrative Advisor Date Chair, Regional Association of Directors Date Administrator, Cooperative State Research, Date Education and Extension Service
Literature Cited
Literature Cited Alexander, M., Hughes, J. B., Chaney, R. L., Cunningham, S. D., Harmsen, J., and Gestel, H. van. Chemical Measures of Bioavailability. p. 345-362. In Lanno, R. P. (eds.) Contaminated Soils: From Soil-Chemical Interactions to Ecosystem Management. Soc. Environ. Contam. Toxicol., Pensacola, FL. 2003. Amajirionwu, M., N. Connaughton, B. McCann, R. Moles, J. Bartlett, and B.ORegan. 2008. Indicators for managing biosolids in Ireland. J. Environ. Management 88:1361-1372 Ashbolt NJ, Amézquita A, Backhaus T, Borriello SP, Brandt KK, Collignon P, et al. 2013. Human health risk assessment (hhra) for environmental development and transfer of antibiotic resistance. Environ Health Perspect 121:993-1001. Attanayake, C.P., G.M. Hettiarachchi, A. Harms, D. Presley, S. Martin, and G.M. Pierzynski. 2014. Field Evaluations on Soil Plant Transfer of Lead from an Urban Garden Soil. J. Environ. Qual. doi:10.2134/jeq2013.07.0273. Barbarick, K.A., J.A. Ippolito, and J. McDaniel. 2010. Fifteen years of wheat yield, N-uptake, and soil nitrate-N dynamics in a biosolids-amended agroecosystem. J. Agic. Ecosys. Environ.116-120. Barbarick, K.A., J.A. Ippolito, J. McDaniel, N.C. Hansen, and G.A. Peterson. 2012. Biosolids application to no-till dryland agroecosystems. Agic. Ecosys. Environ. 150:72-81. Basta, N.T., R. Gradwohl, K.L. Snethen, and J.L. Schroder. 2001. Chemical immobilization of lead, zinc, and cadmium in smelter-contaminated soils using biosolids and rock phosphate. J. Environ. Qual. 30:1222-1230. Basta, N.T., R.R. Rodriguez, D.C. Ward, S.W. Casteel, and L.W. Pace. 2003. Chemical extraction methods to assess bioavailable As in contaminated soil and solid media. J. Environ. Qual. 32:876-884. Basta, N.T., J.A. Ryan, and R. L. Chaney. 2005. Trace element chemistry in residual-treated soil: Key concepts and metal bioavailability. J. Environ. Qual. 34: 49-63. Basta, N.T., J. N. Foster, E.A. Dayton, R. R. Rodriguez, and S.W. Casteel. 2007. The effect of dosing vehicle on arsenic bioaccessibility in smelter-contaminated soils. Invited manuscript. Basta, N. T., Foster, J. N., Dayton, E. A., Rodriguez, R. R., & Casteel, S. W. 2007. The effect of dosing vehicle on arsenic bioaccessibility in smelter-contaminated soils. J. Environ. Health Sci. A42:1275-1281. Basta NT. Are phosphorous in situ Pb stabilization treatments equal? Biogeochemical interactions affecting bioavailability and remediation of hazardous substances in the environment. In: Proceedings of the Lead Phosphate Amendments Symposium at the 246th American Chemical Society National Meeting, 2013. Indianapolis, Indiana. Bolan, N.S., A. Kunhikrishnan, R. Naidu. 2013. Carbon storage in a heavy clay soil landfill site after biosolids application. Sci. Tot. Environ. 465:216-225 Brown , S. , R. Chaney J. Halfrisch, and Q. Xue. 2003. Effect of Biosolids Processing on Lead Bioavailability in an Urban Soil. J. Environ. Qual. 32:100-108. Brown, S., C.L. Henry, R.Chaney, H. Compton, and P.S. DeVolder. 2003. Using municipal biosolids in combination with other residuals to restore metal-contaminated mining areas. Plant and Soil, 249: 203-215 Brown, S., R. Chaney, J. Hallfrisch, J.A. Ryan, and W.R. Berti. 2004. In situ soil treatments to reduce the phyto- and bioavailability of lead, zinc, and cadmium. J. Environ. Qual. 33: 522531. Brown , S. L., I. Clausen, M. A. Chappell, A. Williams, K. G. Scheckel, M. Neuville, and G. M. Hettiarachchi. 2012. Lead and As speciation and bioaccessibility in compost amended contaminated soils. J. Environ. Qual. 41:1612-1622 Brown, S. 2012. Wastewater treatment facilities as renewable resource centers. Biocycle March 2012: 41-44. Brown, S., A. Carpenter, and N. Beecher. 2010. Calculator tool for determining greenhouse gas emissions for biosolids processing and end use. Environ. Sci. & Tech. 44: 9505-9515. Brown, S., K. Kurtz, A. Bary, and C. Cogger. 2011. Long-term effects of organic amendments on soil carbon storage and physical properties. Environ. Sci. & Tech. dx.doi.org/10.1021/es2010418 Cartron, J.M., Weil, R.R., 1998. Seasonal trends in soil nitrogen from injected or surfaceincorporated sewage sludge applied to corn. Communications in Soil Science and Plant Analysis 29, 121-139. Castillo, M. S., L. E. Sollenberger, J.M.B Vendramini, K.R. Woodard, G.A. OConnor, Y.C. Newman, M.L. Silveira, and J.B. Sartain. 2010. Municipal biosolids as an alternative nutrient source for bioenergy crops: I. Elephantgrass biomass production and soil responses. Agron. J. 102: 1308-1313. Cripps, R.W., Winfree, S.K., Reagan, J.L., 1992. Effects of sewage sludge application method on corn production. Communications in Soil Science and Plant Analysis 23, 1705-1715. DAmore, J.M., S.R. Al-Abed, K.G. Scheckel, and J.A. Ryan. 2005. Methods and Techniques for the Speciation of Metals in Contaminated Soils: A Comprehensive Review. J. Environ. Qual. 34: 1707-1745. Daniels W. L, A. F. Wick, N.W. Haus, C. Carter and G.R. Whittecar. 2010. Beneficial utilization of dredge sediments for land rehabilitation. P. 247-254 In: Fox, H.R. and H.M. Moore (eds.) Restoration and Recovery: Regenerating Land and Communities. Glamorgan, Wales. Whittles Pub., Dunbeath, Scotland, September 7-9. Davis, A.P., Hunt, W.F., Traver, R.G. and Clar, M., 2009. Bioretention technology: Overview of current practice and future needs. Journal of Environmental Engineering, 135 (3), 109-117 Available from: http://link.aip.org/link/?QEE/135/109/1. Denys S, Caboche J, Tack K, Rychen G, Wragg J, Cave M, et al. 2012. In vivo validation of the unified BARGE method to assess the bioaccessibility of arsenic, antimony, cadmium, and lead in soils. Environ Sci Technol 46(11):6252-6260. Drexler JW, Brattin WJ. 2007. An in vitro procedure for estimation of lead relative bioavailability: With validation. Human and Ecological Risk Assessment 13(2):383-401. Dere, A.L, R.C. Stehouwer, 2011. Labile and stable nitrogen and carbon in mine soil reclaimed with manure-based amendments. Soil Sci. Soc. Am. J. 75:890-897. Dere, A.L,, R.C. Stehouwer, E. Aboukila and K.E. McDonald. 2012. Nutrient leaching and soil retention in mined land reclaimed with stabilized manure. J. Environ. Qual. 20120:0 - doi 10.2134/jeq2012.0036. Dere, A.L., R.C. Stehouwer and K.E. McDonald. 2011. Nutrient leaching and switchgrass growth in mine soil columns amended with poultry manure. Soil Science 176:84-90. DeVolder, P., S.L. Brown, D. Hesterberg and K. Pandya. 2003. Metal bioavailability and speciation in a wetland tailings repository amended with biosolids compost, wood ash, and sulfate. J. Environ. Qual., 32 (3): 851-864. Farfel, M. R., A.O. Orlova, S.J. Lees, R.L. Chaney, C. Rohde, and P.J. Ashley. 2005. Biosolids application for reducing urban soil lead hazards. Sci. Total Environ. 340:81-95. Finley RL, Collignon P, Larsson DGJ, McEwen SA, Li X-Z, Gaze WH, et al. 2013. The scourge of antibiotic resistance: The important role of the environment. Clin Infect Dis 57:704-710. Gaze WH, S. M. Krone, D. G. J. Larsson, X.-Z. Li, J. Robinson, P. Simonet, K. Smalla, M. Timinouni, E. Topp, E. M. Wellington, G. D. Wright, Yong-Guan Zhu. 2013. The impact of humans on the evolution and mobilization of the environmental antibiotic resistome. Emerging Infectious Diseases [Internet Gaze WH, Zhang LH, Abdouslam NA, Hawkey PM, Calvo-Bado L, Royle J, et al. 2011. Impacts of anthropogenic activity on the ecology of class 1 integrons and integron-associated genes in the environment. ISME J 5:1253-1261. Gilmour, J.T., Clark, M.D., 1988. Nitrogen Release from Wastewater Sludge: A Site Specific Approach. Journal (Water Pollution Control Federation) 60, 494-498. Goven, J. and E.R. Langer. 2009. The potential of public engagement in sustainable waste management: designing the future for biosolids in New Zealand. J. Environmental Management 90:921-930. Heckman, J.R., J.S. Angle, and R.L. Chaney. 1987. Residual effects of sewage sludge on soybeans: II. Accumulation of soil and symbiotically fixed nitrogen. J. Environ. Qual. 16:118-124. Hettiarachchi, G. M. and G. M. Pierzynski. 2002. In situ stabilization of soil lead using phosphorus and manganese oxide: Influence of plant growth. J. Environ. Qual. 31: 564-572. Hettiarachchi, G. M., G. M. Pierzynski, F. W. Oehme, O. Sonmez, and J.A. Ryan. 2003a. Treatment of contaminated soil with phosphorus and manganese oxide reduces absorption of lead by Sprague-Dawley rats. J. Environ. Qual. 32: 1335-1345. Hettiarachchi, G. M., J. A. Ryan, R. L. Chaney, and C.M. La Fleur. 2003b. Adsorption of cadmium onto different fractions of biosolids-amended soils. J. Environ. Qual. 32: 1684-1693. Hettiarachchi, G.M., J. A. Ryan, K. G. Scheckel, S. R. Sutton, and M. Newville. 2006. µ-XANES and µ-XRF investigations of metal binding mechanisms in biosolids. J. Environ. Qual. 35: 342-351. Hsieh, C.-h., and A. P. Davis. 2005. Evaluation and optimization of bioretention media for treatment of urban storm water runoff. Journal of Environmental Engineering 131(11):1521-1531. James, D. W., and K. L. Wells, Soil sample collection and handling: technique based on source and degree of field variability. In Soil Testing and Plant Analysis, Westerman, R. L., Ed. Soil Science Society of America, Inc.: Madison, WI, USA, 1990; pp 25-44. Jones, A. D.; Bruland, G. L.; Agrawal, S. G.; Vasudevan, D., Factors influencing the sorption of oxytetracycline to soils. Environmental Toxicology and Chemistry 2005, 24 (4), 761-70. Juhasz AL, Weber J, Smith E, Naidu R, Marschner B, Rees M, et al. 2009. Evaluation of SBRC-gastric and SBRC-intestinal methods for the prediction of in vivo relative lead bioavailability in contaminated soils. Environ Sci Technol 43(12):4503-4509. Juhasz, A.L., N.T. Basta, and E. Smith. 2013. What is required for the validation of in vitro assays for predicting contaminant relative bioavailability? Considerations and criteria. Environmental Pollution 180:372-375. Kar, G., L.S. Hundal, J.J. Schoenau, and D. Peak. 2011. Direct chemical speciation of P in sequential chemical extraction residues using P K-edge XANES spectroscopy. Soil Sci. 176(11):589-595. King, G.M., G.OConnor, S. Brown, C. Gerba, J. Brooks, and I. Pepper. 2011. Sustainable management of organic residuals: microbial contributions, microbial solutions. ASM Press, Washington, D.C. http://asm.org/index.php/policy/biorep8-2011.html Kinney, C.A., E.T. Furlong, S.L. Werner, and J.D. Cahill. 2006. Presence and distribution of wastewater-derived pharmaceuticals in soil irrigated with reclaimed wastewater. Environ. Toxic. Chem. 25:317-326. Kirchmann, H., Gerzabek, M.H. (Eds.), 2002. Pore size changes in a long-term field experiment with organic amendments. Elsevier Science B.V., Amsterdam, Netherlands. Koplin, D.W., M. Skopec, M.T. Meyer, E.T. Furlong, and S.D. Zaugg. 2004. Urban contribution of pharmaceuticals and other organic wastewater contaminants to streams during different flow conditions. Sci. Total Environ. 328: 1-3(?), 119-130. Kreuzig, R.; Kullmer, C.; Matthies, B.; Holtge, S.; Dieckmann, H., Fate and behaviour of pharmaceutical residues in soils. Fresenius Environ. Bull. 2003, 12 (6), 550-558. Lal, R. 2007. Soil science and the carbon civilization. Soil Sci. Soc. Am. J. 71:1425-1437. Li, J. G.K. Evanylo, X. Zhang, and EH. Ervin. 2013. Effects of biosolids treatment processes on nitrogen cycling and carbon accumulation under various tillage practices. J. Residuals Science and Technology 10:29-40. Lucas, W. C., and M. Greenway. 2008. Nutrient retention in vegetated and nonvegetated bioretention mesocosms. Journal of Irrigation and Drainage Engineering 134(5):613-623. Lucas, W. C., and M. Greenway. 2011. Phosphorus retention by bioretention mesocosms using media formulated for phosphorus sorption: response to accelerated loads. Journal of Irrigation and Drainage Engineering 137(Special):144-153. Biosolids Treatment Process and Soil Phosphorus Availability. J. Environ. Qual. 30, 1023-1033. Mao, J. D.; Hundal, L. S.; Schmidt-Rohr, K.; Thompson, M. L., Nuclear Magnetic Resonance and Diffuse-Reflectance Infrared Fourier Transform Spectroscopy of Biosolids-Derived Biocolloidal Organic Matter. Environmental Science & Technology 2003, 37 (9), 1751-1757. Metcalf & Eddy. 2007. Water reuse. Issues, technologies, and applications. McGraw-Hill Publisher, New York, NY. Metzger, L., Yaron, B., 1987. Influence of Sludge Organic Matter on Soil Physical Properties. In: Stewart, B.A. (Ed.), Advances in Soil Science. Springer New York, pp. 141-163. Miller, G.W. 2006. Integrated concepts in water reuse: Managing global water needs. Desalination. 187:65-75. Miller, G 2012. Getting minds out of the sewer. Science. 33:679-680 Minca, K. K., and Basta, N. T. 2013. Comparison of plant nutrient and environmental soil tests to predict Pb in urban soils. Science of the Total Environment. 445:57-63. Moffet, C. A.; Zartman, R. E.; Wester, D. B.; Sosebee, R. E., Surface Biosolids Application: Effects on Infiltration, Erosion, and Soil Organic Carbon in Chihuahuan Desert Grasslands and Shrublands. J. Environ. Qual. 2005, 34 (1), 299. Munir M, Xagoraraki I. 2011. Levels of antibiotic resistance genes in manure, biosolids, and fertilized soil. J. Environ. Qual. 40:248-255. National Research Council (NRC). 2002. Biosolids Applied to Land: Advancing Standards and Practices. National Academic Press, Washington, DC. National Research Council (NRC). 2008. Urban stormwater management in the United States. Washington, DC. NEBRA A National Biosolids Regulation, Quality, End Use & Disposal Survey 2007, http://www.nebiosolids.org/. OConnor, G.A., H.A. Elliott, and R.K. Bastian. 2008. Degraded water reuse: An overview. J. Environ. Qual. 37:S158-S168. Ozores-Hampton, M., Stansly, P.A., Salame, T.P., 2011. Soil Chemical, Physical, and Biological Properties of a Sandy Soil Subjected to Long-Term Organic Amendments. Journal of Sustainable Agriculture 35, 243-259. Pagliai, M., Antisari, L.V., 1993. Influence of waste organic matter on soil micro- and macrostructure. Bioresource Technology. 43, 205213. Peak, D., G. Kar, L.S. Hundal, and J.J. Schoenau. 2012. Kinetics and mechanisms of phosphorus release in a soil amended with biosolids or inorganic fertilizer. Soil Sci. 177(3): 183-187 Pedersen, J.A., M.A. Yeager, and I.H. Suffet. 2003. Xenobiotic organic compounds in runoff from fields irrigated with treated wastewater. J. Agric. Food Chem. 51:1360-1372. Pignatello, J. J.; Xing, B., Mechanisms of Slow Sorption of Organic Chemicals to Natural Particles. Environmental Science & Technology 1995, 30 (1), 1-11. Pouyat, R.V., Szlavecz, K., Yesilonis, I.D., Groffman, P.M., Schwarz, K., 2010. Chemical, Physical, and Biological Characteristics of Urban Soils. Urban Ecosystem Ecology agronomymonogra, 119-152. Punshon, T., Adriano, D.C., Weber, J.T., 2002. Restoration of drastically eroded land using coal fly ash and poultry biosolid. Science of The Total Environment 296, 209-225. Quaggiotti, S., B. Ruperti, D. Pizzeghello, O. Francioso, V. Tugnoli, and S. Nardi. 2004. Effect of low molecular size humic substances on nitrate uptake and expression of genes involved in nitrate transport in Maize (Zea mays L.). J. Exp. Bot. 55:803-813. Quin, Y. and B. Mecham. 2005. Long-term effects of recycled wastewater irrigation on soil chemical properties on golf course fairways. Agron. J. 97:717-721. Richter, O.; Kullmer, C.; Kreuzig, R., Metabolic Fate Modeling of Selected Human Pharmaceuticals in Soils. CLEAN Soil, Air, Water 2007, 35 (5), 495-503. Rodriguez, R.R., N.T. Basta, S.W. Casteel, and L.W. Pace. 1999. An in vitro gastrointestinal method to estimate bioavailable arsenic in contaminated soils and solid media. Environ. Sci. Technol. 33:642-649. Rodriguez, R.R., N.T. Basta, S.W. Casteel, F.P. Armstrong, and D.C. Ward. 2003. Chemical extraction methods to assess bioavailable As in contaminated soil and solid media. J. Environ. Qual. 32:876-884. Rostagno, C. M.; Sosebee, R. B., Surface application of biosolids in the Chihuahuan Desert: Effects on soil physical properties. Arid land research and management 2001, 15 (3), 233-244. Ryan, J.A., G.M. Hettiarachchi, and K.G. Scheckel. 2003. Effects of Biosolids on sorption qnd desorption behavior of cadmium in biosolids-amended soils. Annual Meeting of the Soil Science Society of America, Denver, CO. Ryan, J.A., W.R. Berti, S.L. Brown, S.W. Casteel, R.L. Chaney, M. Doolan, P. Grevatt, J. Hallfrisch, M. Maddaloni, D. Moseby, and K. Scheckel. 2004a. Reducing childrens risk to soil lead: summary of a field experiment. Environ. Sci. and Tech. 38:19a-24a. Ryan, J.A., G.M. Hettiarachchi, K.G. Scheckel, and R.L. Chaney. 2004b. Effect of biosolids application on soil metal chemistry and phytoavailability. Sustainable Land Application Conference, Lake Buena Vista, FL. Ruby, M.V., Schoof, R., Brattin, W., Goldade, M., Post, G., Harnois, M., Mosby, D.E., Casteel, S.W., Berti, W., Carpenter, M. 1999. Advances in evaluating the oral bioavailability of inorganics in soil for use in human health risk assessment. Environ. Sci. Technol. 33:3697-3705. Sahs, W. W., and G. Lesoing. 1985. Crop rotations and manure versus agricultural chemicals in dry land grain production. J. Soil Water Cons. 40:511-516. Sarkar, D. and G.A. OConnor. 2004. Plant and soil responses to biosolids-P in two Florida soils with high P content. Commun. Soil Sci. Pl. Anal. 35:1569-1589. Scheckel, K. G.; C.A. Impellitteri, J.A. Ryan, J. A.and T. McEvoy. 2003. Assessment of a Sequential Extraction Procedure for Perturbed Lead-Contaminated Samples with and without Phosphorus Amendments Environ. Sci. Tech. 37:1892-1898. Scheckel, K.G., G.M. Hettiarachchi, and J.A. Ryan. 2004.Spectroscopic approaches to defining the inorganic and organic constituents of biosolids. Sustainable Land Application Conference, Lake Buena Vista, FL. Smith, S.R., Woods, V., Evans, T.D., 1998. Nitrate dynamics in biosolids-treated soils. I. Influence of biosolids type and soil type. Bioresource Technology 66, 139-149. Scharenbroch, B.C., E.N. Meza, M. Catania, and K. Fite. 2013. Biochar and Biosolids Increase Tree Growth and Improve Soil Quality for Urban Landscapes. J. Environ. Qual. 42:13721385 Schroder, J.L., N.T. Basta, S.W. Casteel, and J. Si. 2003. An in vitro method to estimate bioavailable cadmium in contaminated soil. Environ. Sci. Technol. 37:1365-1370. Schnell, R.W., Vietor, D.M., Munster, C.L., White, R.H., Provin, T.L., 2010. Effect of turfgrass establishment practices and composted biosolids on water quality. Journal of Environmental Quality 39, 697-705. Spargo, J.T., M.M. Alley, R.F. Follett, J. V. Wallace. 2008 Soil carbon sequestration with continuous no-till management of grain cropping systems in the Virginia Coastal Plain. In review. Stoffella, P.J., Ozores-Hampton, M., Roe, N.E., Li, Y.C., Obreza, T.A., 2003. Compost utilization in vegetable crop production systems. Acta horticulturae, 125-128. Stumpf, M., F.T. Ternes, R-D. Wilken, S.V. Rodrigues, W. Baumann. 1999. Polar drug residues in sewage and natural waters in the state of Rio de Janeiro, Brazil. Sci. Total. Env. 225:135-141. Sydnor, M.E.W., Redente, E.F., 2002. Reclamation of High-Elevation, Acidic Mine Waste with Organic Amendments and Topsoil. J. Environ. Qual. 31, 1528-1537. Thangarajan, R. N.S. Bolan, G. Tian, R. Naidu, A. Kunhikrishnan. 2013. Role of organic amendment application on greenhouse gas emission from soil. Sci. Tot. Environ. 465:72-96 Tian G, Granato T, Cox A, Pietz R, Carlson C, Abedin Z. Soil carbon sequestration resulting from long-term application of biosolids for land reclamation. J Environ Qual 2009;38:6174. Tian, G., T.C. Granato, A.E. Cox, R.I. Pietz, C.R. Carlson, Jr., and Z. Abedin. 2009. Soil carbon sequestration resulting from long-term application of biosolids for land reclamation. J. Environ. Qual. 38:61-74. Topp, E. and M. Colucci. 2004. Persistence of some estrogenic chemicals in agricultural soils. Sustainable Land Application Conference. Lake Buena Vista, FL. Trlica, A. and S. Brown. 2013. Greenhouse gas emissions and the interrelation of urban and forest sectors in reclaiming one hectare of land in the Pacific Northwest. Environ. Sci. Technol., 2013, 47 (13), pp 72507259 Tsadilas, C. D.; Mitsios, I. K.; Golia, E., Influence of Biosolids Application on Some Soil Physical Properties. Communications in Soil Science & Plant Analysis 2005, 36 (4-6), 709-716. USEPA Method 1614: Brominated Diphenyl Ethers in Water Soil, Sediment and Tissue by HRGC/HRMS. 2007. U.S. Environmental Protection Agency, Office of Water, Office of Science and Technology Engineering and Analysis Division (4303T), Washington, DC. EPA-821-R-07-005. USEPA Method 1694: Pharmaceuticals and Personal Care Products in Water, Soil, Sediment, and Biosolids by HPLC/MS/MS. 2007. U.S. Environmental Protection Agency, Office of Water, Office of Science and Technology Engineering and Analysis Division (4303T), Washington, DC. EPA-821-R-08-002. USEPA. 2008. Fate, Transport and Transformation Test Guidelines: OPPTS 835.0001 Principles and Strategies Related to Biodegradation Testing of Organic Chemicals under the Toxic Substances Control Act (TSCA) 2008. USEPA. 2008. Fate, Transport and Transformation Test Guidelines: OPPTS 835.1230 Adsorption/Desorption (Batch Equilibrium). 2008. USEPA. 1998. Fate, Transport and Transformation Test Guidelines: Sediment and Soil Adsorption/Desorption Isotherm. EPA 712C98048. 1998. Vietor, D.M., Schnell, R.W., Munster, C.L., Provin, T.L., White, R.H., 2010. Biosolid and Alum effects on runoff losses during turfgrass establishment. Bioresource Technology 101, 3246-3252. Walker, C. and H.S. Lin. 2008. Soil property changes after four decades of wastewater irrigation: A landscape perspective. Caterna 73:63-74. Wallace, B. M. 2008. Biosolids increase soil aggregation and protection of soil carbon five years after application on a crested wheatgrass pasture. J. Environ. Qual. 38:291-298. Walters, E.; McClellan, K.; Halden, R. U., Occurrence and loss over three years of 72 pharmaceuticals and personal care products from biosolidssoil mixtures in outdoor mesocosms. Water research (Oxford) 2010, 44 (20), 6011-6020. Writer, J. H., L. B. Barber, G. K. Brown, H. E. Taylor, R. L. Kiesling, M. L. Ferrey, N. D. Jahns, S. E. Bartell, and H. L. Schoenfuss. 2010. Anthropogenic tracers, endocrine disrupting chemicals, and endocrine disruption in Minnesota lakes. Science of the Total Environment 409, 100-111. Xia, K. and G. Pillar. 2004. Fate of personal care products and other organic chemicals in biosolids. Sustainable Land Application Conference. Lake Buena Vista, FL. Xia, K., A. Bhandari, K. Das, and G. Pillar. 2005. Occurrence and fate of pharmaceuticals and personal care products (PPCPs) in biosolids. J. Environ. Qual. 34:91-104. Xia, K.; Hundal, L. S.; Kumar, K.; Armbrust, K.; Cox, A. E.; Granato, T. C., Triclocarban, triclosan, polybrominated diphenyl ethers, and 4-nonylphenol in biosolids and in soil receiving 33-year biosolids application. Environmental Toxicology and Chemistry 2010, 29 (3), 597-605. Xu, J.; Wu, L.; Chang, A. C., Degradation and adsorption of selected pharmaceuticals and personal care products (TOrCs) in agricultural soils. Chemosphere (Oxford) 2009, 77 (10), 1299-305. Zhang, X., E. Ervin, G. Evanylo, C. Sherony, and C. Peot. 2005. Biosolids impact on tall fescue drought resistance. J. Residuals Science & Technology 2:173-180.