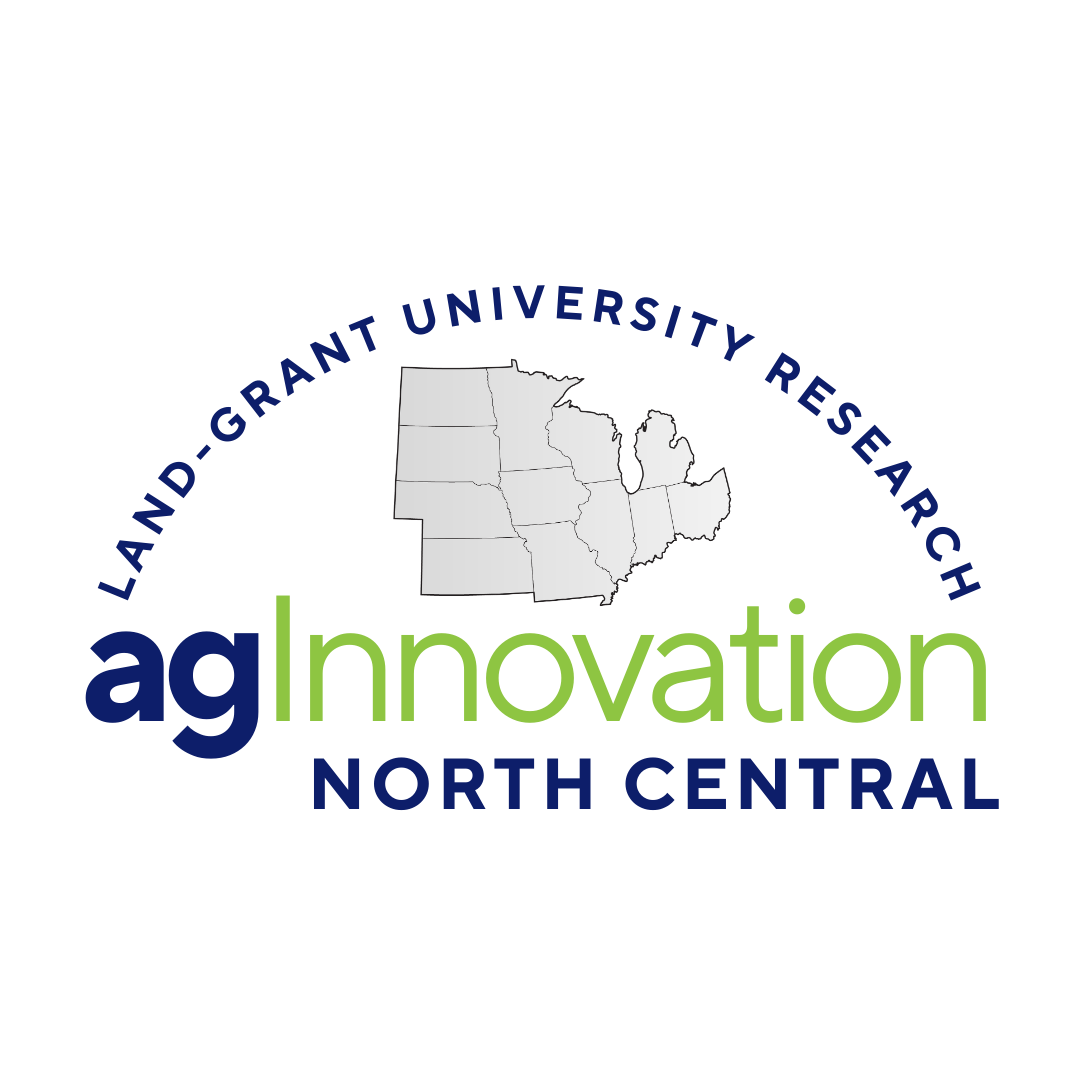
NC_OLD1186: Water Management and Quality for Ornamental Crop Production and Health
(Multistate Research Project)
Status: Inactive/Terminating
NC_OLD1186: Water Management and Quality for Ornamental Crop Production and Health
Duration: 10/01/2010 to 09/30/2015
Administrative Advisor(s):
NIFA Reps:
Non-Technical Summary
Statement of Issues and Justification
The ornamental plant industry ranks 5th (>$14.6 billion) in US agriculture commodities and is in the top 5 commodities for 26 states (USDA, 2004). Water issues, specifically irrigation scheduling, surface water management, salinity and runoff water quality are topics of major concern to ornamental producers. Drought, urban competition for water resources, and increasing legislation at state and county levels increase the need for ornamental producers to manage water more effectively and/or use alternative water sources that are often of inferior quality. Regardless of the area of the United States in which an operation is located, challenges exist regarding sufficient quantities of quality water sources. Legislation regarding water use and/or quality has been implemented in at least 8 states. Most field producers of nursery stock use irrigation at some point during the growing season. Many field producers use low-volume irrigation and some use such systems to deliver soluble fertilizers. While supplemental irrigation is beneficial in field production it is essential for container production. Container substrates need to be well drained and container volume limits the amount of available water, resulting in frequent irrigation and high water use. Almost all greenhouse crops are produced in containers. Over 75% of nursery crops in 17 of the major nursery producing states were grown in containers (USDA, 2007) and thus require irrigation. In Florida, container nurseries annually apply 56 to 120 inches per year in addition to the 40 to 50 inches of average annual rainfall. Container nurseries in Alabama were estimated to have used 30,000 to 40,000 acre-feet of water in 1985 (Fare et al., 1992) and container nursery production in Alabama has almost tripled since 1987 (USDA 1994, 2004). Amount of water applied, method of application, and irrigation frequency for Georgia nurseries has been summarized (Garber et al., 2002). Frequent irrigation along with high fertilizer and pesticide use can lead to significant losses of agricultural chemicals in runoff water that transports them to containment ponds and/or off-site into groundwater or surface water (Briggs et al., 1998, 2002; Cabrera, 2003; Camper et al., 1994). Irrigation water management is a key component in the nutrient management of ornamental crop production and in reducing the impact of runoff water on local water (Tyler et al., 1996; Lea-Cox et al., 2001; Ross et al., 2002). Recycling water includes another set of issues for growers, primarily in the form of disease and salinity management. Emerging constraints on water use and quality means that the ornamental industry needs to find ways to manage water without detracting from production schedules and crop quality. Water conservation and quality are top priority issues in agriculture. Research and extension projects that are designed to address these issues are needed in ornamental production (Ogg and Keith, 2002). Precision water management and resource efficiency were rated at the top of the issue/need/concern list developed at the joint USDA, ARS, NASA and NSF workshop Engineering Solutions for Specialty Crop Challenges (USDA, 2007). Furthermore, the United States Environmental Protection Agency (EPA) is enforcing federal legislation requiring states to implement Total Maximum Daily Load (TMDL) programs for watersheds (Lea-Cox and Ross, 2001). Since few states have the expertise to integrate all these issues we need a national multi-disciplinary approach to water management. There are five interrelated areas relevant to this project: 1. Source water management and quality, 2. Irrigation management, 3. Runoff water management and quality, 4. Substrate and nutrition management, and 5. Pathogens and crop health management. 1.1 Source Water Management and Quality There are four primary water sources available for ornamental production: groundwater, surface water, reclaimed water, and recycled tailwater from runoff. All of these water sources can have quality issues that require management before they can be used for plant production. Additionally in some areas of the country, due to increased demand for freshwater, agriculture is being forced to use alternative water sources, such as reclaimed or recycled water, which may have quality management issues. Plant producers therefore must develop new technologies to remove harmful contaminants when necessary, modify horticultural practices, and/or develop or choose crops which are more tolerant of lower quality water. Many areas of the country have groundwater supplies that are being contaminated by saltwater intrusion as a result of removing groundwater faster than it is being recharged. This problem is occurring in both coastal areas where seawater is intruding and other inland areas with saline deep aquifers (Atkinson, 1986, Barlow, 2005). Groundwater is also being contaminated by infiltration of contaminants from nearby industrial, urban, and agricultural operations. In other regions of the U.S., groundwater is impaired by natural geological features, making water alkaline, sodic, and/or saline. Surface water is even more vulnerable to contamination since it has no protective over-layer of soil. Surface water supplies are becoming use-restricted. In various parts of the SE, SW and NE U.S., recent droughts have reduced surface water levels to historic record lows. In addition, environmental agencies are claiming more surface water to protect endangered flora and fauna along waterways. In California, the Sacramento-San Joaquin River Delta, once a primary source of fresh water for agriculture, now has use restrictions to protect delta smelt populations. In Georgia, there is a mandatory release of water from Lake Lanier to support endangered mussels and sturgeon on the Chattahoochee River, to support a nuclear plant in Alabama, and supply water for southern Alabama, southern Georgia and Florida. Reclaimed water, which is wastewater or sewage that has been treated with conventional wastewater treatment processes or other processes, is being used in various parts of the country to irrigate specific agricultural crops. While reclaimed water offers a water source that can be available when other water sources are limited, there are drawbacks. Agriculture uses a significant portion of reclaimed water, using 48% in CA and 19% in FL in 2002. While not universally available, reclaimed water is available in many urban areas of the U.S. Benefits of using reclaimed water include lower costs and access to a water source that may be less affected by drought. However, the drawbacks of reclaimed water are maintenance cost of a separate water system, potential contamination with salts, heavy metals, and chlorine that may be harmful to sensitive crops. In many areas of the country, ornamental producers have begun recycling the tailwater and stormwater runoff from their facilities. This process can save money, especially for larger operations, since fertilizers in the runoff are re-utilized. However, runoff water may contain pesticide residues. Agricultural chemicals have been found in runoff ranging from less than 0.1 % to 20% recovery of active ingredient (Briggs et al., 2002; Riley et al., 1994; Willis, 1982). Pesticides and nitrates were detected in nursery runoff water and in nursery retention basins (Keese et al., 1994; Warsaw et al., 2009a; Wilson et al., 1996). Phytotoxicity problems may result when recycled water contains either pesticides with medium to high water solubilities or one pesticide is extensively used and the recycled water is applied to plants sensitive to that pesticide (Bhandary et al., 1997). Herbicide contamination levels of 1 to 10 ppm for short durations were found to be detrimental to growth and quality of several ornamental crops (Bhandary et al., 1997; Fernandez et al., 1999). Other drawbacks of recycling includes the need for adequate infrastructure to collect, capture and treat irrigation water runoff. However, in areas such as California, recycling of tailwater has been practiced since the 1970s (Skimina, 1986). 1.2 Irrigation Management Ideally when irrigating with good quality water, only the amount of water used through evapotranspiration is replenished. Applying water in excess of evapotranspiration can lead to reduced growth and nutrient loss (Tyler et al., 1996; Warsaw et al., 2009a, 2009b). However, irrigation system uniformity and efficiency are always less than 100% and result in wasted water. Manual versus automated irrigation systems and how those systems are managed also affects water use efficiency. Water quality and quantity issues affect irrigation management decisions: when water with high soluble salts is used for irrigation, a high leaching fraction may be required to prevent the buildup of excess salts in the substrate. Conversely, alkaline water should be applied at the lowest possible rates to minimize effects on substrate pH. Either of these scenarios can lead to nutrient management problems, a longer production cycle and more water used over the extended cycle (Beeson, 2006). Over-application of water is both an inefficient use of water and the main cause of fertilizer runoff (Bilderback, 2002; McAvoy, 1994; Warsaw et al., 2009a). New state laws have been passed that regulate the amount of runoff from all forms of agriculture (Lea-Cox and Ross, 2001). Excessive irrigation may also result in anaerobic conditions in the root zone possibly resulting in: 1) a root systems more susceptible to pathogens (Powell and Lindquist, 1997), 2) dissemination of pathogens from production areas, potentially leading to contamination of irrigation ponds, 3) nutritional problems due to denitrification and effects on root physiology and soil/substrate pH, 4) leaching of water, nutrients, pesticides, and herbicides from production areas posing a threat to water quality, and 5) excessive stem elongation, reduced plant quality and increased shipping costs. Excessive irrigation also has a direct impact on production costs: when a municipal water source is used, growers are charged on a per volume basis, while growers using well water incur energy costs associated with pumping water. Further, leaching nutrients from containers requires that greater quantities of fertilizer be used. Applying water efficiently and using more sustainable irrigation techniques will reduce production costs, conserve water, and produce higher quality crops. More precise control over irrigation will also allow growers to expose their crops to mild water stress, potentially hardening them to a drought stress that the plants may experience in the retail environment or landscape (Eakes et al., 1991). Reducing irrigation has been studied as a method to reduce stem elongation and/or improve quality of bedding plants (van Iersel and Nemali, 2004; Barrett and Nell, 1991; Latimer, 1992), perennial plants (Burnett and van Iersel, 2008) and woody plants (Warsaw et al., 2009a; 2009b). This has the potential to reduce the need for growth retardant applications or shorten the production cycle. Recent advances in irrigation technology have made it easier for growers to reduce water use during production and to harden plants by limiting water without risking plant damage or loss due to excessive drought stress. Such technology can assure an adequate water supply to the crop, even if environmental conditions change rapidly (Nemali and van Iersel, 2006). Because water traditionally has been available in abundant quantities in many parts of the country, irrigation recommendations for ornamental plant production have not received much attention. Currently, reliable tools and information to precisely schedule irrigations for diverse crop species in different environments is the missing link to reduce water use, optimize water use efficiency and minimize irrigation nutrient and chemical runoff from ornamental plant production. To reduce nutrient runoff and address issues related to irrigation water availability, it is crucial that more efficient approaches to irrigation, ground and surface water, and nutrient best management practices be developed (Lea-Cox et al., 2001). 1.3 Runoff Water Management and Quality. Container production creates the largest challenge to managing runoff water. The volume of water used and amount of runoff generated is much less for field production but issues are similar and still can have a major impact on water resources. Runoff of rain and irrigation water is an important avenue for the movement of agrichemicals from production sites into nearby receiving water bodies (Bjorneberg et al., 2002; Keese et al., 1994; Latimer et al., 1996; Meisinger and Delgado, 2002). If subsurface flow occurs and it is largely shallow and in a lateral direction, receiving bodies may be nearby surface waters located within or near the production site. If the site is underlain by porous soils or shallow aquifers, subsurface flow may directly enter groundwater resources. However, if properly managed surface runoff can be channeled and contained on site for reuse (Skimina, 1986; Hasek et al., 1986) or treated to remove sediment and/or agrichemical pollutants (Huett et al., 2005). Assessment and management of runoff therefore plays a critical role in minimizing environmental impacts of ornamental plant production operations. Fertilizer recommendations for ornamental plants often exceed recommendations for other similar agricultural crops (Rose, 1999). While controlled-release fertilizers (CRFs) are commonly used in container production, the nature of substrates and irrigation practices often leads to nutrient leaching. Pesticides are commonly applied every two to three weeks and up to 80% of an applied material can miss the application target (Gilliam et al., 1992). The current best management practice recommendation for overhead irrigation is that 10-20% of the applied water should leach out of containers. Overhead irrigation creates runoff water which transports pesticides and fertilizers to containment ponds and/or off-site before they can undergo degradation or be bound to soils/substrates. The plant production surface at container nurseries or greenhouses is commonly covered with plastic, fabric, gravel, concrete or other low permeable material. All ground covers result in greater surface runoff amounts than bare ground because of reduced infiltration and sheet flow. The combination of these factors leads to significant levels of agricultural chemicals in runoff water. The environmental fate of the pesticides is complex. Willis (1982) cites nine factors as having an effect on the concentration of pesticides in runoff water. The three most important are rainfall intensity and duration, amount of time between pesticide application and rainfall, and properties of the pesticide. In many production systems the daily use of overhead irrigation in effect increases rainfall frequency and decreases the time interval between pesticide application and rainfall. Concern for the protection of water resources, and the detrimental reintroduction of pollutants onto the crop through recycling, dictates that techniques to reduce the movement and enhance remediation of agricultural chemicals in runoff waters be developed. Unfortunately, little is known about the current state of runoff management in the ornamental plant industries throughout the United States (Yeager et al., 1993). Runoff can be substantially reduced by proper irrigation management (Warsaw et al., 2009b). Capturing and treating or recycling are other options. Many larger greenhouses have addressed the issue of runoff by using closed irrigation systems. Although subirrigation systems can virtually eliminate runoff from greenhouses, they are cost-prohibitive for many, especially for nurseries and small greenhouses. 1.4 Substrate and Nutrition Management Water runoff, fertilizer leaching and effects on recycling water systems are important considerations when selecting the components that formulate container substrates. Additionally, the use of inorganic and biological amendments including clay, pumice, mycorrhizae, and other biofungal amendments could be part of the formulations that could improve water and nutrient management of ornamental crops. With the increase in prices and availability of current substrate components, the interest within the ornamental industry to identify alternative materials that are suitable for formulating container substrates has also increased. Most of the alternative mixes can be used successfully to amend conventional substrates when used at proper ratios. However, an integrated approach to assess the physical and chemical properties of substrate mixes and their impact on plant health and growth and nutrient and water management is lacking. Pre-plant fertilization is achieved by incorporating water-soluble fertilizer, CRFs, and/or lime and microelements into the substrate (Nelson, 2008). CRFs are often applied when plants are initially potted. For established plants, top-dressing with CRFs during the spring or a combination of CRFs and water-soluble fertilizers is done. In some cases, particularly greenhouse and field production, water-soluble fertilizers serve as the primary fertilizer and are either applied each time the crop is irrigated or at regular intervals. Water-soluble fertilizers, by their nature, are more prone to leaching if mismanaged. Fertilizer concentrations are determined based on the N requirements of the crop. Many fertilizers are not well-balanced, containing considerable more P than required. Thus, by fertilizing based on N requirements, P is applied in excess. Substrate chemical and physical properties in combination with irrigation management and water quality affect leaching of nutrients regardless of management method and thus, the quality of runoff water. Excessive water applications result in substantial leaching of nutrients, and leaching of N and P can be of special concern. The susceptibility of N to leaching depends on the form of N that is applied. Due to the extremely low anion exchange capacity of many soilless substrates, nitrate is very susceptible to leaching, while ammonium (NH4+) leaches less readily due to the cation exchange capacity of the substrate. Although the use of NH4+ reduces the likelihood of N leaching, many greenhouse crops are susceptible to NH4+ toxicity, and a general guideline suggests not applying more that 40% of total N in the form of NH4+ or urea (Nelson, 2008). 1.5 Pathogens and Crop Health Management Plant pathogens in irrigation water were recognized early in the last century as a significant crop health issue (Bewley and Buddin, 1921). Plant pathogens threaten the sustainability and profitability of the ornamental plant industries as much as water shortages. Recycling irrigation conserves water but it may spread pathogens from a single point to an entire enterprise and from a single farm to other facilities sharing the same water resource (Hong et al., 2008c). This could result in severe losses of both crop and consumer confidence. At least 17 Phytophthora species, 26 of Pythium, 27 genera of fungi, 8 species of bacteria, 10 viruses, and 13 nematode species have been detected from water sources (Hong and Moorman, 2005). Among those pathogens are the sudden oak death (SOD) pathogen, and Ralstonia solanacearum, one of the USDA select agents under the Agricultural Bioterrorism Protection Act of 2002. Thus, there is an urgent need to assess the waterborne pathogen risk and develop mitigation strategies. Restrictions or timing of irrigation to conserve water may also decrease crop health due to coincidence of irrigation and ideal conditions for disease development. These issues have increased greatly in degree of impact and it will continue to be a problem with the increasing dependence on alternative irrigation water sources (Hong and Moorman, 2005).
Related, Current and Previous Work
2.1 Source Water Management and Quality It has only been during the last few decades that the detrimental aspects of agricultural chemicals in runoff have been considered in ornamental production. In 1994, Niemiera (1994) linked irrigation management with nutrient leaching fractions and gave recommendations for monitoring production areas to reduce the environmental impact of management practices. Ornamental producers can now employ numerous best management practices (BMPs) to increase the efficiency of water and nutrient management (Yeager et al., 1997; Yeager et al., 2007). Ornamental producers have taken the forefront in initiating more efficient use of water resources (Skimina, 1986). Research has also been conducted to optimize water and fertilizer use as a result of limited water availability, increased fertilizer cost, and environmental concerns (Albano et al., 2006; Blythe et al., 2006; Merhaut et al., 2006; Newman et al., 2006; Ristvey, 2004; Ristvey et al. 2004; 2007; Warsaw et al., 2009a, 2009b). Yet, due to the enormous diversity of crops grown, many relationships are not well understood. Little information is available regarding the impact of lower quality water sources on ornamental production. Also, the presence and consequences of pesticides and plant and human pathogens in water sources must be investigated. 2.2 Runoff Water Management and Quality Runoff is unavoidable in container production since irrigation often hits semi-permeable to impermeable surfaces. Runoff often results in nutrients, herbicides and pesticides being transported to non-target sites such as nearby ground and surface waters. Yeager et al. (1993) reported elevated concentrations of N in nursery runoff and receiving water bodies in six southeastern states. Briggs et al. (1998, 2002) showed that pesticides are transported away from production beds in irrigation runoff. Because irrigation efficiencies are often low and rainfall is uncontrollable without overhead structures, managing runoff even with good irrigation management poses a problem for nurseries that do not contain and recycle water. Runoff management has two primary goals: 1) interception and containment 2) reuse/treatment. Containment entails channeling and collecting runoff water in a non-erosive fashion to a water conservation structure, allowing the water to be stored for reuse or treatment prior to discharge. Technologies developed and tested for removing agrichemicals from runoff water include both extensive natural systems (Headley et al., 2001; Ullah and Zinati, 2006) and intensive bioreactor systems (Modzden, 2007). Some technical guidelines for runoff management have been described (Yeager et al., 2007). More recently, Yeager (2008) has assessed capture and recycling of irrigation water and Ross (2008a) has provided guidelines for containment basin design. Several coordinated research projects are involved with runoff issues in ornamental production. The USDA-ARS Floral and Nursery Initiative (FNI) has a multi-pronged approach of 1) assessing runoff quantity and nutrient content, 2) developing a simulation model for predicting runoff quantity and quality, and 3) testing wetland and bioreactor systems for remediating runoff water. The FNI research group is focused on the Eastern U.S. but the information learned from this project could be tested for applicability to other locations nationwide. For example, wetland and bioreactor systems found to work in eastern nurseries could be tested in western locations. Another multi-state outreach project also in the Eastern U.S. is the on-line Green Industry Knowledge Center for Water, Nutrient and Plant Health Management (http://www.waternut.org/moodle). Twenty five in-depth learning modules have been developed by faculty from 6 land-grant institutions. This Knowledge Center provides research-based best management practices for substrate, irrigation, surface water, nutrient and crop health management for nursery and greenhouse environments (Lea-Cox et al., 2008b). Revising and adding modules to this knowledge center would provide a wider range of water management information, tools and advanced technology to ornamental producers nationwide. 2.3 Irrigation Management Different approaches have been used in the past to develop more efficient irrigation practices. Irrigation systems are primarily dependent upon good design and continued maintenance (Ross, 2008b, c). However, the critical issue for most growers is scheduling irrigation, which is a complex daily decision often based upon subjective techniques (Lea-Cox et al., 2009). When controlling irrigation based on real-time measurements, the first decision is whether to use a measure of plant water status or the water status of the substrate/soil to measure plant water availability. Both of these approaches have advantages and disadvantages. In principle, controlling the water status of the plant may allow for more direct control of the physiology and growth of the plants. Leaf temperature, leaf reflectance, leaf/stem water potential, and stem diameter have all been used as indicators of plant water status. Leaf temperature measurements can be used to determine the crop water stress index (CWSI), which can then be used to control irrigation. Prenger et al. (2005) found that irrigation was reduced by approximately 50% when based on CWSI as compared to using a timer. The difference between leaf and air temperature also can be used as an indicator of leaf water status (Blom-Zandstra and Metselaar, 2006). Measuring leaf reflectance, potentially by remote sensing techniques, is another approach that may be used to determine the crop water status. Ritchie et al. (2005) used remote sensing to determine the normalized difference vegetation index (NDVI) to trigger irrigation of cotton when the crop showed signs of drought stress. However, it is not clear how this and other canopy stress measurement techniques could be adapted for use in intensive ornamental plant production, which have mixed blocks of species, different ages of plants and varying water availability at any one time. Additional drawbacks to using plant water status for irrigation scheduling include that plant-based measurements may be able to detect the onset of stress but are not well suited for the prevention of stress, they can change rapidly making them difficult to use for irrigation control (Jones, 2004; 2008) and they are not necessarily an indication of drought but can also be caused by root disease or poor root/vascular functioning. Jones (2004; 2008) argues that measurements of soil water status are better suited for irrigation control than plant water status. In this case, the first decision to be made is whether to measure volumetric water content or matric potential. Tensiometers have long been used to measure the matric potential of soils and soilless substrates (Lieth and Burger, 1989). Although tensiometers have proven to be valuable research tools, they are difficult to use in substrates and some soils. Tensiometers rely on close contact between their ceramic tip and the soil or substrate. If the soil or substrate shrinks or the tensiometer is moved accidentally this contact may be disrupted and the tensiometer will not work properly (Zazueta et al., 1994). These problems, and the need for routine maintenance, have limited the use of tensiometers in commercial ornamental production. Matric potential is a direct measure of how easily available soil or substrate water is for plant uptake. However, matric potential does not indicate the quantity of water present. Volumetric moisture sensors can be used to determine how much water is present in the soil or substrate but do not indicate how easily plants can absorb water. Soil/substrate moisture release curves can be developed to convert matric potential to volumetric water content, but such relationships are soil/substrate specific (Arguedas et al., 2007). Ristvey et al. (2004) used time domain reflectometry (TDR) to measure substrate water content and control irrigation of Ilex cornuta. They found that using TDR sensors for monitoring and control of irrigation reduced water use by 60 85%, with similar reductions in N and P leaching, compared to cyclic irrigation, a currently recommended best management practice. However, TDR systems are expensive to install, limited by non-wireless signal transmission and the data interpretation can be complicated. As such, these systems are not well-suited for large-scale commercial ornamental production either. In recent years, several new soil moisture sensors have become available. These new sensors determine the volumetric moisture content by measuring the apparent dielectric constant of the substrate. This technology is simple and highly reproducible. Nemali and van Iersel (2006) used such capacitance sensors to build an automated system for irrigation monitoring and control, and this system has since been used successfully for quantifying water requirement of Gaura (Burnett and van Iersel, 2008) and to determine plant physiological responses to drought stress (Nemali and van Iersel, 2008). Although it is currently possible for growers to control irrigation using soil moisture sensors, it is important to develop systems that allow growers to easily collect, store, and analyze the data from these sensors. Lea-Cox et al. (2007; 2008b) have developed/adapted wireless sensor networks that can be used to gather data throughout a greenhouse or nursery. Data is transmitted to a central computer, from where data streams can be monitored and irrigation set points can be adjusted as needed. Using wide area sensor networks, they were able to go beyond the measurement of a single variable, such as soil/substrate moisture, by integrating them with other environmental variables including water inputs (rainfall, irrigation volumes), electrical conductivity (salt accumulation), light, soil and air temperature, relative humidity and wind speed/direction. By integrating these variables into predictive models (Bauerle et al., 2002; Bowden et al., 2005; Kim and van Iersel, 2009), it should be possible to move towards a more robust capability to predict plant water use. In order to do this however, more sophisticated software and interfaces that are user-friendly and convenient need to be developed for growers. 2.4 Substrate and Nutrition Management Since there is no ideal substrate with universal availability, substrate mixtures are often a compromise to achieve optimal growth for a relatively broad range of species at a feasible cost. Soilless substrates primarily consist of organic material, with higher air-filled porosity and lower ion exchange capacities than soils (Bilderback et al., 2007). The lower ion exchange capacity results in a high leaching potential. The addition of clay, humus, peat, composted pine bark, composted sawdust, or vermiculite as a substrate amendment can greatly increase the cation exchange capacity (Handreck and Black, 2002). Typical amendments to increase anion exchange capacity are calcined clays, attapulgite, and shale, but in general, most soilless substrates have very low anion exchange capacity (Handreck and Black, 2002). There is a need to rigorously quantify nutrient application, use, leaching and runoff from ornamental production systems. An effective nutrient management strategy is dependent on the interactions between soils/substrates, fertilization and irrigation practices, and runoff-water management (Lea-Cox and Ross, 2007). Very few quantitative system approaches exist for ornamental production situations, especially container production which constitutes the highest-risk for water and nutrient runoff (Lea-Cox et al., 2001). The first step in reducing nutrient use in ornamental production is obtaining a better understanding of plant nutrient requirements. Even though there are exhaustive numbers of journal articles on maximizing plant growth with increasing nutrient addition, there are very few studies in the ornamental literature that quantify all the above-mentioned variables and understanding the interactions with the environment. While more research is needed to quantify the water and nutrient requirements for many plant species, information from a few well-studied species may be used as a basis for irrigation and nutrient application recommendations (Cabrera, 2003; Ku and Hershey, 1997a; 1997b; Ristvey, 2004; Ristvey et al., 2007; Rose, 1999). Ku and Hershey (1997a, b) were among the first to publish a complete N and P budget study for poinsettia and they concluded that with constant leaching fractions of 0.2, there was still a potential for at least 50% of the applied N to be lost. This is notable, since a leaching fraction of 15% is considered a best management practice (Yeager et al., 2007). The additional practice of leaching only when EC levels rise to 1.52.0 dS·m-1 could further reduce nutrient runoff. Rose (1999) suggested that current fertilizer recommendations for many field-grown ornamental plants far exceed recommendations for other crops, and are based on a goal of maximum growth rate rather than best management practice goals. Ristvey et al. (2007) concluded from studies on azalea that N loss could be reduced from 1100 to 326 kg·ha1 by reducing N application rates from 250 mg N·L-1 to 100 mg N·L-1, a nearly threefold decrease in potential N loss to the environment. Ristvey concluded that even greater reductions in P loading could be achieved by decreasing P application rates to levels more attuned to actual plant requirements (100 vs. 22 kg·ha1). When nutrient budgets are reported in the literature, there is often a moderate to high percent of N that cannot be accounted for in the total budget, even with 15N studies (Lea-Cox et al., 2001). Cabrera (2003) reported 23% - 41% of the N could not be accounted for with fertilizer N concentrations from 15 - 300 mg·L-1. This N loss is typically attributed to denitrification. Denitrification has been identified as a major N loss mechanism in agriculture. Denitrification within the root zone can decrease the available N for plants, requiring additional N applications. Control of denitrification rates could increase N availability and reduce over-application and cost. Nieder et al. (1989) reported on a number of in situ denitrification experiments and found a range of 5 kg·ha1 to 60 kg·ha1 N losses using 15N labeled compounds, and N loss rates of 0.7 to 233 kg·ha1 using the acetylene inhibition method over a variety of conditions. Ristvey (2004) concluded that N loss could be exacerbated by high (daily) irrigation applications in container-production. Aulakh et al. (1992) report that denitrification is negligible below 60% of water holding capacity, at least for mineral soils. Therefore, denitrification could reasonably be presumed to be a major loss mechanism of N from ornamental production systems, and yet there is very little information on this subject in the ornamental literature. Denitrification inhibitors, which can be mixed with fertilizers or container substrates, have shown limited ability to control denitrification in soilless substrates yet are a relatively low cost way of reducing denitrification rates (Goh and Haynes, 1977). It is likely that the best reductions in denitrification rates will be seen with a combination of denitrification inhibitors, appropriate rates of CRFs, and better irrigation practices. 2.5 Pathogens and Crop Health Management Plant pathogens in irrigation water were recognized early in the last century as a significant crop health issue (Bewley and Buddin, 1921). This issue has increased greatly in scope and degree of impact since that time and it will continue to be a problem as horticulture increasingly depends on the use of alternative water sources (Hong and Moorman, 2005). Existing studies are directed largely towards identification of water molds and adaptation of water treatments developed and used for drinking water. Water molds are a group of oomycetes including species of Phytophthora and Pythium as well as downy mildew pathogens. A number of Phytophthora species were detected from nursery irrigation systems previously in California (MacDonald et al., 1994), North Carolina (Lauderdale and Jones, 1997), Oklahoma (Von Broembsen and Wilson, 1998) and Virginia (Bush et al., 2006). Similarly, numerous species of Pythium have been recovered from recycling irrigation systems (Bush et al., 2003; Hong and Moorman, 2005). As monitoring of waterborne pathogens intensified, more species including several new species of Phytophthora have been identified (Hong et al., 2008a, b). We are at the beginning of a learning curve about the diversity of plant pathogens in irrigation water. New records and species of water molds will continue to emerge. Diversity of plant pathogenic bacteria, fungi, nematodes, viruses and other pests in irrigation water is largely unknown. But it is clear that irrigation water is a major, if not the sole source of inoculum for water molds in ornamental production (Hong et al., 2002a,b; Stanghellini, et al.,1996a,b; Stanghellini and Miller, 1997; Stanghellini and Rasmussen, 1994). An array of treatments has been developed for effectively treating drinking water but few have been evaluated for use in agricultural settings. Chlorination remains economically the most viable option for irrigation water decontamination (Hong and Richardson, 2004; Hong et al., 2003b). However, the efficacy of this technology is subject to water pH, the highest at pH 5.5 to 6.5 and dropping dramatically with increasing pH, by 80% at pH 8 (White, 1999). Water in most irrigation reservoirs is alkaline (Hong et al., 2009a; Kong et al., 2009). Thus, acidification often is required prior to use of chlorination for the most effective water treatment. Liquid chlorine is corrosive and gaseous chlorine can be explosive, presenting significant health risks to laborers and costs to producers. Promising alternatives include use of chlorine dioxide (Copes et al., 2004), surfactants (Stanghellini and Miller, 1997; Stanghellini et al., 1996a, b) and heat pasteurization (McPherson et al., 1995). These have been used in commercial greenhouse production to treat recirculating water. A major challenge is applying them to nursery settings where much larger quantities of water are used. Water treatment certainly is a critical strategy to dissociate crop health risk from recycling practices but it is equally important to reduce the amount of pathogens returning to irrigation reservoirs (Hong, 2009). Many growers have seen the advantages and economics of switching from overhead to drip and/or pulse irrigation for water resource conservation and protection but few realize its potential benefits in water and crop health improvement. The smaller the amount of runoff water returning to tanks or reservoirs, the fewer pathogens will be recycled. Irrigation scheduling also could be a powerful tool for crop health management. Some pathogens have a daily cycle of production of sporangia and zoospores in response to light. Production of sporangia is mainly diurnal, whereas production of zoospores is primarily nocturnal (Nielsen et al., 2006), therefore, phytophthora diseases spread several times faster on plants irrigated at night. Irrigation scheduling also may shorten the length of the wet period that is required for many important plant pathogens to germinate and initiate infection processes. In addition, proper watering enhances plant vigor and immunity. Meeting plant water needs may be accomplished by watering several times a day in small amounts each time or watering less frequently but thoroughly every time. Apparently the former would prolong the wet period on the plant surface, promoting pathogen germination and subsequent infection compared to the latter.
Objectives
-
Develop an effective multistate group to identify knowledge gaps in water management and quality for ornamental production; organize transdisciplinary teams to address these gaps, and leverage existing national programs to maximize impact.
-
Develop effective outreach programs which a) change behavior and implement best management practices; b) increase resource use-efficiency and minimize environmental impacts of practices; c) increase production efficiency and profitability and d) allow regulatory agency and public sectors to access baseline information which can be used for policy and other decision-making.
-
Disseminate research results to the academic community through traditional means (e.g. peer reviewed journals, and extension programs) and also more novel web-based methods (knowledge centers, eXtension and social networks)
-
Integrate projects, programs and results according to the following specific project objectives.
-
Gather information regarding the quantity and quality of primary water sources currently available in various regions of the United States.
-
Determine what water quality parameters limit ornamental plant production and how secondary water sources differ throughout different regions of the U.S.
-
Determine national research priorities regarding water quantity and quality of primary and secondary water sources.
-
Obtain funding and conduct research to address the physical and chemical limitations of primary and secondary water sources.
-
Gather comprehensive runoff-related information from the following sectors: a) growers, b) regulatory agencies, c) university research and extension, and d) public.
-
Assess the relative impacts of nursery runoff on surface and groundwater resources through detailed on-site investigations
-
Determine priority areas for improving runoff management and obtain funding to address these specific research and extension needs.
-
Determine the water requirements of a variety of ornamental plants and how these water requirements are affected by plant size and environmental conditions.
-
Compare different irrigation methods (overhead, spray stakes, drip irrigation, subirrigation) to determine how they affect total water use, plant growth and quality, and runoff water quality.
-
Quantify reductions in water use, leaching, and runoff that result from more efficient irrigation techniques
-
Develop new methods to provide growers with real time information regarding the water requirements of their crops, including crop water use models and sensor networks that can be easily deployed in greenhouses and nurseries.
-
Determine the economic impact of more efficient irrigation practices (cost/benefit analyses).
-
Gather information on what has been published in the area of substrate media and on commonly used components and formulated media per state per region.
-
Gather information on available recycled organic components per region that have potential use for cost effective media mixes.
-
Gather information on production quantity and cost of the components used across the regions.
-
Assess physical and chemical properties of formulated media mixes and their impact on plant health and nutrient levels in leachates for a variety of plants considered of importance to these states/regions.
-
Develop BMP guidelines for substrate/amendment management practices based on the above objectives.
-
Characterize pests in irrigation reservoirs and other water sources
-
Elucidate the aquatic ecology of water molds and develop biologically-based water decontamination technology
-
Expand evaluation of and improve existing water treatments
-
Develop best management practices to improve crop health and reduce the amount of pests returning to runoff irrigation reservoirs
Methods
The methods are described in the context of the objectives in the previous section, but it is important to realize that these are not clearly delineated areas; rather there is much overlap among these areas, which is necessary to assure that this project will integrate the most promising approaches. Each subsection of the methods will be coordinated by one of the project members identified at the annual meeting. Subsection leaders and the project executive committee will accomplish coordination of methods to attain overall project objectives. 4.1 Source Water Management and Quality Methods for 3.1.a. and b. The workgroup will gather information through surveys to ornamental nursery growers. The workgroup will also gather data from state water boards to determine the quantity and quality of primary and secondary water sources. Methods for 3.1.c. The workgroup will meet with other scientists at professional meetings and with representatives from State Water Boards to determine the course of action feasible to make primary and secondary water sources economically and responsibly utilized by the ornamental nursery industry. Methods for 3.1.d. Conduct multistate projects with other scientists to determine how to overcome limitations in water quantity and quality. These projects may be conducted onsite at participating nurseries, onsite and/or in more controlled conditions like land grant experiment stations located throughout the U.S. Methods for 3.1.e. Research results will be published in peer-reviewed journals so that new technologies and research information can be improved upon. Extension programs will be conducted in a manner to efficiently and effectively disseminate information to nursery growers. Methods of outreach will include publications in common trade magazines, websites, and onsite programs 4.2 Runoff Water Management and Quality Methods for 3.2.a. This would be a major effort of the runoff subgroup. A coordinated survey would be taken of important stakeholders including: nursery growers, regulatory agencies, research and extension groups, and the public including environmental groups. Some critical areas for the survey would include determining the: i) current state of water use and runoff quantity and quality in nurseries ii) extent that containment and water reuse practices are being employed iii) extent that monitoring and remediation of runoff are being practiced iv) financial or technological roadblocks to effectively managing runoff. Runoff subgroup leaders will work together with representatives of the stakeholders to be surveyed to develop a survey that will provide the needed information in these critical areas. Growers in the survey will be asked if they would be willing to participate in detailed evaluations as described in Objective 2 below. Methods for 3.2.b. Several nurseries will be selected in each subgroup leaders region to participate in on-site detailed runoff investigations. For participating nurseries, investigators will conduct an extensive monitoring of water use, runoff, and runoff impacts in the nursery. Emphasis of this investigation will be on the movement of water and agrichemicals within and out of the participating nurseries. Monitoring will entail measuring flow and water quality in both well water and surface water within the nursery and at strategic outlets. Important questions to be answered include: i) What is the quantity and quality of runoff generated at the production bed level? ii) Where is the runoff going and what impact does it have on ground and surface water resources? iii) Are containment and treatment practices effective in reducing these impacts? By conducting these detailed investigations with uniform monitoring procedures, an assessment of actual runoff dynamics in nurseries in a wide range of locations can be made. In combination with the survey information obtained in Objective 1, a baseline of valuable information will be available for developing strategies in Objectives 3 and 4. Methods for 3.2.c. and d. Runoff subgroup leaders will use the results generated in Objectives 1 and 2 to formulate research and extension strategies for improving runoff management in ornamental nurseries. Where research needs are indicated, the baseline information will be invaluable in the ability of the subgroup leaders to garner the necessary funding to carry out the required research. Subgroup leaders will also be charged with the task of developing extension strategies which promote adoption of best management practices for managing runoff in the nursery. Among other factors, these strategies will take into consideration the financial and technological roadblocks that might become evident in the survey. For example, results from survey and the detailed, on-site runoff investigations will provide data which can be used by the runoff subgroup leaders to help in garnering or developing cost-sharing programs to help growers implement best management practices. This same information will also help in garnering additional funding for improving technological outreach activities such as expanding the runoff-related information available on the previously described web-based Green Industry Knowledge Center. 4.3 Irrigation Management Methods for 3.3.a. The efficiency of water applications depends greatly on how the water is delivered. A wide variety of irrigation systems are used in greenhouses and nurseries, ranging from recirculating subirrigation systems, to drip irrigation, to spray stakes, and to overhead sprinklers. In general, the more efficient irrigation systems are more expensive to install and maintain. There is little comprehensive information available on differences in water use among these different irrigation approaches, and even less information on their economics. We will determine how the irrigation method affects water use in greenhouses and nurseries. This information will be valuable for growers when determining the costs and benefits of different irrigation approaches. Methods for 3.3.b. i) Use soil moisture sensors to measure substrate water status and control irrigation. We will test a variety of soil moisture sensors for their usefulness in soilless substrates, and then select the most appropriate sensors for use in studies where substrate water content will be monitored and controlled ii) Measure plant responses to drying substrates and detect the early onset of drought stress to determine when irrigation is needed. Plant parameters to be measured could include changes in stem caliper, leaf temperature, leaf reflectance, stomatal conductance, and photosynthesis. iii) Quantify the effects of mild drought stress and plant anatomical, morphological, and physiological responses to determine how plant quality is affected iv) Use weather station data to determine the reference evapotranspiration and combine this information with crop coefficients to determine crop-specific irrigation models v) Develop crop water use models to estimate daily water use of plants. Such models can be based on estimates of plant water use and substrate/soil water content to predict plant physiological responses. Methods for 3.3.c. The benefits of efficient irrigation methods go beyond reductions in water use. Equally important are reduced leaching and runoff. Reduction in leaching and runoff of nutrients (and N and P in particular) need to be quantified. Since substrates differ in water holding capacity and ion exchange capacity, leaching and runoff is likely to be dependent on the physical and chemical properties of the substrate. Interactions between substrate properties and irrigation practices are therefore likely to affect leaching and runoff, and optimal irrigation practices may be substrate dependent. Thus, different irrigation methods will be tested in a variety of substrates. Methods for 3.3.d. Growers will need simple, effective methods to monitor and control the water status of their crop. The University of Maryland and Carnegie Mellon have jointly developed wireless sensor networks that can get a large amount of information, but simple methods to organize and analyze this information are lacking. We will develop database tools that make it feasible for growers to collect data from sensors distributed throughout their greenhouse or nursery, and to effectively use that information for more efficient irrigation management. An alternative approach is to minimize data that need to be handled by growers: stand-alone irrigation controllers may be able to make autonomous decisions about when and for how long to irrigate, based on weather or substrate conditions detected by the controller. Developing and testing such controllers will provide an alternative to growers who want to use simple system, without extensive data management. Methods for 3.3.e. The benefits of any technology or practice depends upon how quickly and extensively they are adopted by industry, but follow two general pathways: i) Private Benefits will include those that accrue to nursery and horticultural businesses because of the higher prices they may be able to receive because of higher product quality and because of cost savings associated with increased resource use efficiency and other related reductions in input requirements. ii) Public Benefits will include those associated with reduced demand for water and reduced water discharges and related pollutant discharges and reduced energy use and air emissions. The value of some environmental improvements and related ecosystem services may be measurable directly using non-market valuation methods. The value of others may be imputed by examining the level of spending that is taking place elsewhere to achieve the same reductions in water use and water and air emissions. 4.4 Substrate and Nutrient Management Methods for 3.4.a.-c. Gather published information and survey the stakeholders on commonly used components and mixes used per state/region. The survey will include sections that address quantities of substrate components and amendments and their costs. Methods for 3.4.d. and e. Identify the gaps in research and extension areas and team up with other researchers across the nation to seek funding to address the multifaceted and multidisciplinary projects that address the impact of using major substrates and amendments on plant health, leachate quality, and water use on the basis of the physical and chemical properties. The subgroup along with other researchers will set up research studies that address the above objectives. Methods for 3.4.f. and g. Use the results generated from the 3.4.a.-e. to develop guidelines for suitable substrates and amendments that provide optimum physical and chemical properties as well as sustainable crop production. The subgroup will develop effective outreach program to develop demonstration sites and educate nursery stakeholders on integration of management practices. The subgroup will continue to seek funding to support the outreach program and expand the information necessary that help growers adopt the management practices. 4.5 Pathogen and Crop Health Management Methods for 3.5.a. and b. We have recently described several new species of Phytophthora including P. irrigata and P. hydropathica by morphology, DNA fingerprints and sequence analysis (Hong et al., 2008a, b). We will use the same methodology to characterize other new species found in irrigation water. Phytophthora species declined in irrigation reservoirs along water path from irrigation runoff entrance to outlet/pump inlet (Bush et al., 2003; Ghimire et al., 2006; Hong et al., 2003a). We will continue investigation of spatial distribution of pathogens in irrigation reservoirs and how they may be related to water quality. We also will expand the studies on pathogen aquatic ecology and consequently develop biologically-based pathogen avoidance and mitigation strategy. Methods for 3.5.c. and d. In addition to improving chlorination protocols, we have evaluated ultraviolet irradiance for irrigation water decontamination (Cohn and Hong, 2003). Our next step is to revamp the heat pasteurization procedure. Current procedure recommends treating water at 95C for 30 second. A major limitation with this procedure is high energy consumption and cost. Thus, we will look into whether and how water temperature can be lowered without sacrificing efficacy. Specifically, we will determine the effect of water temperature on survival of different pathogen structures including zoospores, chlamydospores, oospores and mycelium and how treatment temperature may be compensated by time. Best management practices will be developed based on results. Methods for 3.5d. Use the results generated from 3.5.a.-c. to develop guidelines that reduce the transmission of pathogens in water and back to plants by using physical, chemical, and biological mitigation techniques. An outreach program to demonstrate for ornamental growers the effectiveness and feasibility of adopting these strategies in reducing pest pressure returning to runoff-irrigation reservoirs, improving crop quality, reducing use of pesticides, and ultimately improving the quality of water used for irrigating ornamental crops.Measurement of Progress and Results
Outputs
- Develop multi-state and multidisciplinary proposals for granting agencies with a goal of at least one proposal submission annually.
- Serve as a clearinghouse for articles and speakers from members to national and member state trade journals, conferences, workshops, and other presentations. The goal will be to have at least 5 articles or presentations related to project activities annually. The internet and other novel methods for delivering information to stakeholders will also be used and developed.
- Present project results at national and international scientific conferences as symposia, colloquia, workshops, presentations or posters. The goal will be to have at least one presentation from the project annually.
- Refereed journal articles will be developed based on activities generated by the project. In the first few years during the planning or establishment phase, refereed publications probably will not be generated but the goal is to have at least 3 refereed publications during the first 5 years of the project related to the groups activities.
- 5.1 Source Water Management and Quality a. The results of surveys to nursery growers and information collected from State Water Boards regarding primary and secondary water sources will be published in refereed journals and trade magazines. b. Develop best management guidelines for water quality from secondary sources. c. Use national research priorities to develop research proposals on water quantity and quality of secondary water sources. 5.2 Runoff Water Management and Quality a. Results of the multi-state survey will be compiled into a report and made available to all stakeholders. The report will include both survey response data, summary, and conclusions. Highlights of the survey will be published in trade and research journals. b. Monitoring results detailing the movement of water and associated agrichemicals from the production area to adjacent water bodies in a wide range of nurseries will be published in trade and research journals. The case studies will be useful in documenting outreach programs that promote effective runoff management. c. Results of the survey along with case study investigations will be used to develop and augment existing on-line educational resources that will be accessible by all stakeholders. These educational materials will include on-line publishing of survey results, case studies, techniques for monitoring runoff, and common means for containment, reuse, and mitigation of runoff water on-site. 5.3 Irrigation Management a. Improved strategies for irrigation management, which reduce water and fertilizer use, while maintaining or improving plant quality. b. Guidelines for growers on the optimal irrigation approach for their operation c. Scientific papers will be written to share the results of the research with other researchers around the world d. Extension publications and articles in trade magazines will make this information readily accessible for growers e. Guidelines for the use of sensors and wireless sensor networks for irrigation control will be developed. 5.4 Substrate and Nutrition Management a. Gathered information and survey results on various components and media mixes used by state and region will be put into a report and become available to ornamental growers to use through fact sheets, workshops and demonstration sites, and online publications. b. Scientific research results will be shared at meetings, trade shows, and published in scientific papers. c. Develop guidelines for ornamental growers that can be used to formulate optimum substrate mixes that match plant needs, reduce nutrient loss through leachates. 5.5 Pathogens and Crop Health Management a. Name five new taxa of Phytophthora that we have recovered and will emerge within the next 4 years b. An improved heat pasteurization protocol by 2013. c. Biologically-based pathogen avoidance and mitigation practice manual by 2015.
Outcomes or Projected Impacts
- Assist the ornamental nursery industry efficiently and successfully utilize available primary and secondary water sources.
- Mitigate nutrient and pesticide runoff into the environment from nursery production facilities.
- Better utilization of limited water resources.
- Through increased use of secondary water sources instead of primary potable water sources, this project will increase the available of potable water for other consumer uses.
- 6.2 Runoff Water Management and Quality a. The survey and detailed investigations will provide much needed baseline information for all stakeholders to make informed assessments of current runoff issues and what solutions are needed to minimize the impacts of nursery runoff. b. Baseline data and case studies will be essential for obtaining additional funding that can be used to develop and test new technologies for controlling and treating runoff. The information should be invaluable for soliciting cost-share or other financial aid that may allow growers to more easily adopt some of the more costly runoff management technologies such as containment basins and monitoring wells as well as to obtain funding for outreach activities such as workshops, publications, and on-line educational programs. c. Outreach activities based upon baseline information developed from surveys and case study investigations will help educate all stakeholders on the rewards for managing runoff to maximize grower profitability and minimize environmental impacts. 6.3 Irrigation Management The impact of this project can be divided into two areas. Those impacts that mainly benefit the greenhouse and nursery industry, and those impacts that benefit society at large. a. Producer (private) benefits: increased profitability by reducing costs associated with irrigation (direct costs of water), reduced power use, savings on fertilizer cost, and labor savings. i. Reduced water and fertilizer use, energy and labor (increased resource use efficiency) ii. Increased production (plant growth benefits) iii. Large reductions in leaching and runoff of water and nutrients (environmental benefits) b. Societal benefits: i. Conservation of water resources. Decreasing the amount of water used by ornamental growers will leave more water available for other uses. ii. Improved water quality. Reducing runoff will help protect the water quality of ground and surface water throughout the US. iii. Decreased carbon footprint of operations 6.4 Substrate and Nutrient Management a. Increasing our knowledge on the currently used substrates and amendments. b. Identify the gaps that require further work, and coordinate with multidisciplinary researchers on funded projects to address the stakeholders needs and finding proper substrate media that produce sustainable nursery crops. c. Identify cost-effective management practices, provide information to the stakeholders on benefits of using suitable amendments and substrate media to reduce water and nutrient runoff and stressing plants. 6.5 Pathogen and Crop Health Management a. Increasing our knowledge on plant pathogens in irrigation water and their significance to the health of ornamental crops and the sustainability of the horticultural industry. b. Provision of novel and improved water decontamination technologies that will help growers mitigate crop health risk, increasing the nations horticultural industrys competitiveness in a global market and long-term sustainability. c. Identifying major knowledge gaps and future research directions. d. Reduce the dependency on using pesticides for controlling diseases caused by phytophthora and pythium (environmental benefit). e. Improve the quality of returning runoff-irrigation water (environmental benefit). f. Outreach activities on managing pathogens in recycled water and plants will help educate all stakeholders on the rewards to maximize profitability and minimize environmental impacts.
Milestones
(2011): Develop and send out survey of water resource use and management to growers. Prepare and submit first group research proposal, proposals will be developed in all years and research will be based on funding success.(2012): Complete survey of water resource use and management by growers, use survey results to refine research proposals and develop outreach information.
(2013): Present survey results to scientific and lay audiences through presentations at scientific and grower meetings and peer reviewed and trade publications.
(2014): Prepare presentations and publications based on previous years' activities and research.
(2015): Prepare presentations and publications based on previous years' activities and research.
Projected Participation
View Appendix E: ParticipationOutreach Plan
Peer-reviewed publications will be generated as well as shorter, trade articles. Presentations will be made at international (International Society for Horticultural Science), national (American Society for Horticultural Science, Southern Nursery Association Researchers Conference, Ohio Florists Short Course, Land Grant and Sea Grant National Water Conference), regional (Southeast Greenhouse Conference), and state association meetings. Nearly every study will include a web component, published on the Committees Website (http://nc216group.ning.com/). Collective and individual manuscripts and presentations are planned. Researchers with extension appointments will take the lead on disseminating information through regional and state trade meetings.
Organization/Governance
The Committee shall be organized as a Multistate Research Project in accordance with the Guidelines for Multistate Research Activities (USDA/CSREES/SAESD/ESCOP, Updated October 16, 2006) and shall operate under the regulations specified therein. 10.1 Members Membership will be open to SAES scientists, other public and private sector scientists, extension professionals, an administrative advisor, a CSREES representative, and others who are in a position to contribute to the proposed activities. Voting members will consist of one representative from each SAES. Voting membership may be extended to other members not affiliated with a SAES upon a majority vote of the voting members. In addition to carrying out the agreed research collaboration, project members are responsible for reporting progress, contributing to the ongoing progress of the activity, and communicating their accomplishments to the committees members and their respective employing institutions. 10.2 Officers All voting members of the committee are eligible for office, regardless of sponsoring agency affiliation. The executive committee consists of the officers: Past chair: The past chair will prepare the annual report for the year in which he/she served as chair. The past chair will assist the chair to insure a smooth transition. The past chair will serve as the chair in the absence of both the elected chair and vice-chair. Chair: In consultation with the administrative adviser, notifies the committee members of the time and place of meetings, prepares the agenda, presides at meetings of the committee and the executive committee. The chair is responsible for preparing or supervising the preparation of the annual report of the project. The chair will serve a one-year term. Vice-chair: Succeeds the chair and is expected to carry out duties assigned by the chair. The chair-elect serves as the chair in the absence of the elected chair. The chair-elect will serve a one-year term and will succeed to the chair the following year. Secretary: records the minutes and performs other duties assigned to him/her by the committee or the administrative advisor. The secretary shall prepare and e-mail the minutes of any official meeting to committee members within four weeks after the end of the meeting. The secretary shall be responsible for assisting the chair prepare official communications to the administrative advisor, NIMSS, and other external parties. The secretary will serve a one-year term and succeed to the Vice-chair the following year. 10.3 Subcommittees Subcommittees will be named by the chair as needed for specific assignments. This may include subcommittees to develop procedures, manuals, and phases of the regional project; to review work assignments; to develop research methods; and to prepare publications.
Literature Cited
Albano, J.P., D.J. Merhaut, E.K. Blythe, and J.P. Newman. 2006. Nutrient release from controlled-release fertilizers in a neutral-pH substrate in an outdoor environment: II. Leachate calcium, magnesium, iron, manganese, zinc, copper, and molybdenum concentrations. HortScience 41(7):1683-1689. Arguedas, F.R., Lea-Cox J.D., and Ristvey A.G. 2007a. Revisiting the measurement of plant available water in soilless substrates. Proc. Southern Nursery Assoc. Res. Conf. 52:111-115. Atkinson, S.F., G.D. Miller, D.S. Curry and S.B. Lee. 1986. Salt water intrusion: Status and potential in the contiguous United States. Chelsea, Michigan: Lewis Publishers. Aulakh, M.S., Doran, J.W. and Mosier, A.R. 1992. Soil denitrification-significance, measurement, and effects of management. Advances in Soil Science. 18: 1-57 Barlow, P.M. 2005. Ground Water in Freshwater-Saltwater Environments of the Atlantic Coast. USGS Circular 1262. U.S. Geological Survey, Information Services, Box 25286, Denver Federal Center, Denver, CO 80225 or http://pubs.water.usgs.gov/cir1262. Barrett, J.E. and T.A. Nell. 1991. Mechanical stress and drought stress effects on height control of greenhouse crops. In: 1991 Proc. Plant Growth Regulat. Soc. Amer., p. 50-56. Bauerle W. L., Post, C.J., McLeod, M.F., Dudley, J.B. and Toler, J.E. 2002. Measurement and modeling of the transpiration of a temperate red maple container nursery. Agr. Forest Meteorology. 114: 45-57. Bewley, W. F., and Buddin, W. 1921. On the fungus flora of glasshouse water supplies in relation to plant diseases. Annals of Applied Biology 8:10-19. Beeson, R.C., Jr. 2006. Relationship of plant growth and actual evapotranspiration to irrigation frequency based on management allowed deficits for container nursery stock. J. Amer. Soc. Hort. Sci. 131:140-148. Bhandary, R., T. Whitwell, J. Briggs, and R.T. Fernandez. 1997. Influence of Surflan (oryzalin)) concentrations in irrigation water on growth and physiological processes of Gardenia jasminoides radicans and Pennisetum rupelli. J. Environ. Hort. 15:169-172. Bilderback, T.E., J.S. Owen, Jr., S.L. Warren, and J.P. Albano. 2007. Non-organic amendments can extend media life: with limited breakdown, they're good additions to bark and peat. Nursery Management Prod. April:37-42. Bjorneberg, D.L., Westermann, D.T., and J.K Aase. 2002. Nutrient losses in surface irrigation runoff. J. Soil Water Conserv. 57:524-529. Blom-Zandstra, M. and Metselaar, K. 2006. Infrared thermometry for early detection of drought stress in Chrysanthemum. HortScience 41: 136-142. Blythe, E.K., D.J. Merhaut, J.P. Newman, and J.P. Albano. 2006. Nutrient release from controlled-release fertilizers in acid substrate in a greenhouse environment: II. Leachate calcium, magnesium, iron, manganese, zinc, copper, and molybdenum concentrations. HortScience 41(3):788-793. Bowden, J.D., Bauerle, W.L., Lea-Cox, J.D. and Kantor, G.F. 2005. Irrigation scheduling: An overview of the potential to integrate modeling and sensing techniques in a Windows-based environment. Proc. Southern Nurs. Assoc. Research Conf. 50: 577-579. Briggs J, T. Whitwell, R.T. Fernandez, M.B. Riley. 2002. Effect of integrated pest management strategies on chlorothalonil, metalaxyl, and thiophanate-methyl runoff at a container nursery. J. Amer. Soc. Hort. Sci. 127:1018-1024. Briggs, J., T. Whitwell, M.B. Riley, and T. Lee. 1998 . Cyclic irrigation and grass waterways combine to reduce isoxaben losses from container plant nurseries. J. Environ. Hort. 16:235-238. Burnett, S.E. and M.W. van Iersel. 2008. Morphology and irrigation efficiency of Gaura lindheimeri grown with capacitance-sensor controlled irrigation. HortScience 43:15551560. Bush, E. A., Stromberg, E. L., Hong, C. X., Richardson, P. A., and Kong, P. 2006. Illustration of key morphological characteristics of Phytophthora species identified in Virginia nursery irrigation water. Plant Health Progress doi:10.1094/PHP-2006-0621-01-RS. Bush, E. A., Hong, C. X., and Stromberg, E. L. 2003. Fluctuations of Phytophthora and Pythium spp. in components of a recycling irrigation system. Plant Disease 87:1500-1506. Cabrera, R.I. 2003. Nitrogen balance for two container-grown woody ornamental plants. Sci. Hort. 97: 297-308. Camper, N. D., T. Whitwell, R. J. Keese and M. B. Riley. 1994. Herbicide levels in nursery containment pond water and sediments. J. Environ. Hort. 12:8-12. Cohn, D., and Hong, C. X. 2003. Efficacy of ultraviolet irradiance for disinfesting recycled irrigation water. Phytopathology 93:S123. Copes, W. E., Chastaganer, G. A., and Hummel, R. L. 2004. activities of chlorine dioxide in a solution of ions and pH against Thielaviopsis basicola and Fusarium oxysporum. Plant Dis. 88:188-194. Eakes, D.J., R.D. Wright, and J.R. Seiler. 1991. Moisture stress conditioning effects on Salvia splendens Bonfire. J. Amer. Soc. Hort. Sci. 116:716-719. Fernandez, R.T., T. Whitwell, M.B. Riley and C.R. Bernard. 1999. Evaluating semiaquatic perennials for use in herbicide phytoremediation. J. Amer. Soc. Hort. Sci. 124:539-544. Garber, M.P., J.M. Ruter, J.T. Midcap and K. Bondari. 2002. Survey of container nursery irrigation practices in Georgia. HortTechnology 12(4):727-731. Ghimire, S. R., Richardson, P. A., Moorman, G. W., Lea-Cox, J., Ross, D. S., and Hong, C. X. 2006. Detection of Phytophthora species in a run-off water retention basin at a commercial nursery in plant hardiness zone 7b of Virginia in winter. Phytopathology 96:S40. Gilliam, C. H., D. C. Fare and A. Beasley. 1992. Nontarget herbicide losses from application of granular ronstar to container nurseries. J. Environ. Hort. 10:175-176. Goh, K.M. and Haynes, R.J. 1977. Evaluation of potting media for commercial nursery production of container grown plants. New Zealand J. of Agricultural Research. 20: 383-393. Handreck, K. and Black, N. 2002. Growing Media for Ornamental Plants and Turf (3rd ed.). University of New South Wales Press, Sydney, Australia. Hasek, R.F., R.H.Sciaroni, and R.L Branson. 1986. Water conservation and recycling in ornamentals production. HortScience 21:35-38. Headley, T.R., D.O. Huett, and L. Davison. 2001. The removal of nutrients from plant nursery irrigation runoff in subsurface horizontal-flow wetlands. Water Sci. Tech. 44:77-84. Hong, C. X. 2009. Re-circulating irrigation without sacrificing crop health. Pages 1-6 in: The 25th Pest Management Conference The American Society of Florists and Greenhouse Grower, San Jose. Hong, C. X., Lea-Cox, J. D., Ross, D. R., Moorman, G. W., Richardson, P. A., Ghimire, S. R., and Kong, P. 2009a. Containment basin water quality fluctuation and implications for crop health management. Irrigation Science 27:485-496 Hong, C. X., Richardson, P. A., Ghimire, S. R., Hao, W., Moorman, G. W., Lea-Cox, J. D., Ross, D. S., Kong, P., and Xu, Z. H. 2009b. The first records of four Phytophthora species from nursery irrigation water in Virginia (Abstr.). Phytopathology 99:S54. Hong, C. X., Gallegly, M. E., Richardson, P. A., Kong, P., and Moorman, G. W. 2008a. Phytophthora irrigata, a new species isolated from irrigation reservoirs and rivers in eastern United States of America. FEMS Microbiology Letters. 285:203-211. Hong, C. X., Gallegly, M. E., Richardson, P. A., Kong, P., Moorman, G. W., Lea-Cox, J. D., and Ross, D. S. 2008b. Phytophthora irrigata and Phytophthora hydropathica, two new species from irrigation water at ornamental plant nurseries. Phytopathology 100:S68. Hong, C. X., Richardson, P. A., and Kong, P. 2008c. Pathogenicity to ornamental plants of some existing species and new taxa of Phytophthora from irrigation water. Plant Disease 92 (8):1201-1207. Hong, C. X., and Moorman, G. W. 2005. Plant pathogens in irrigation water: challenges and opportunities. Critical Reviews in Plant Sciences 24:189-208. Hong, C. X., and Richardson, P. A. 2004. Efficacy of chlorine on Pythium pathogens in irrigation water. Proceedings of the Southern Nursery Association Research Conference 49:265-267. Hong, C. X., Richardson, P. A., and Kong, P. 2003a. Decline in Phytophthora population with increasing distance from runoff water entrance in a retention pond. Phytopathology 93:S36. Hong, C. X., Richardson, P. A., Kong, P., and Bush, E. A. 2003b. Efficacy of chlorine on multiple species of Phytophthora in recycled nursery irrigation water. Plant Disease 87:1183-1189. Hong, C. X., Cohn, D., Kong, P., and Richardson, P. A. 2002. Economic significance to nursery production of Phytophthora species present in irrigation water. Proceedings of Southern Nursery Association Research Conference 47:237-240. Hong, C. X., Kong, P., and Richardson, P. A. 2002. Epidemiological significance of Phytophthora species present in recycled irrigation water to ornamental production. Phytopathology 92:S144. Huett, D.O, S.G. Morris, G. Smith, N. Hunt. 2005. Nitrogen and phosphorus removal from plant nursery runoff in vegetated and unvegetated subsurface flow wetlands. Water Research 39:3259-3272. Jones, H.G. 2008. Irrigation Scheduling Comparison of Soil, Plant and Atmosphere Monitoring Approaches. Acta Hort. 792: 391-403. Jones, H.G. 2004. Irrigation scheduling: advantages and pitfalls of plant-based methods. J. Exp. Bot. 55:2427-2436. Keese, R.J., N.D. Camper, T. Whitwell, M.B. Riley and P.C. Wilson. 1994. Herbicide runoff from ornamental container nurseries. J. Environ. Qual. 23:3320-324. Kim, J. and M.W. van Iersel. 2009. Daily water use of abutilon and lantana at various substrate water contents. Proc. Southern Nursery Assoc. Res. Conf., 54: (In Press) Kong, P., Moorman, G. W., Lea-Cox, J. D., Ross, D. S., Richardson, P. A., and Hong, C. X. 2009. Zoosporic tolerance to pH stress and its implications for Phytophthora species in aquatic ecosystems. Applied and Environmental Microbiology doi:10.1128/AEM.00119-09. Ku, C.S.M. and Hershey, D.R. 1997a. Growth response, nutrient leaching and mass balance for potted poinsettia I. Nitrogen. J. of the Amer. Society of Horticultural Sci. 122: 452-458. Ku, C.S.M. and Hershey, D.R. 1997b. Growth response, nutrient leaching, and mass balance for potted poinsettia II. Phosphorus. J. of the Amer. Society of Horticultural Sci. 122: 459-464. Latimer, J.G. 1992. Drought, paclobutrazol, abscisic acid, and gibberellic acid as alternatives to daminozide in tomato transplant production. J. Amer. Soc. Hort. Sci. 117:243-247. Latimer J.G., R.D. Oetting, P.A. Thomas, D.L. Olson, J.R Allson, S.K. Braman, J.M. Ruter, R.B. Beverly, W. Florkowski, C.D. Robacker, J.T. Walker, M.P. Garber, O.M. Lindstorm, and W. G. Hudson. 1996. Reducing pollution of pesticides and fertilizers in the environmental horticulture industry: I. Greenhouse, nursery and sod production. HortTechnology 6:115-124. Lauderdale, C. C., and Jones, R. K. 1997. Monitoring irrigation ponds for Phytophthora sp. Proceedings of the Southern Nursery Association Research Conference 42:225-226. Lea-Cox, J.D., Ristvey, A.G. and Kantor, G.F. 2009. Wireless water management. American Nurseryman. 44-47. Lea-Cox, J. D., A. G. Ristvey, F. R. Arguedas Rodriguez, D. S. Ross, J. Anhalt and G. Kantor. 2008a. A Low-cost Multihop Wireless Sensor Network, Enabling Real-Time Management of Environmental Data for the Greenhouse and Nursery Industry. Acta Hort 801: 523-529. Lea-Cox, J.D., Zhao, C., Ross, D.S., Bilderback, T.E., Harris, J.R., Hong, C., Yeager, T.H., Bauerle, W.L., Day, S.D., Ristvey, A.G., Beeson, R.C., Jr. and Ruter, J.M. 2008b. An on-line knowledge center for water and nutrient management for the nursery and greenhouse industry. Acta Hort. 801: 693-700. Lea-Cox, J.D. and Ross, D.S. 2007. Water and nutrient management planning. In: Green Industry Knowledge Center for Water and Nutrient Management Learning Modules. J.D Lea-Cox, D.S. Ross and C. Zhao (Eds.). University of Maryland, College Park, Maryland. 43pp. Published online at http://www.waternut.org/moodle/course/view.php?id=6 Lea-Cox, J. D., G. Kantor, J. Anhalt, A. G. Ristvey and D. S. Ross. 2007. A Wireless Sensor Network for the Nursery and Greenhouse Industry. Proc. Southern Nursery Assoc. Res. Conf. 52: 454-458. Lea-Cox, J. and D.S. Ross. 2001. A review of the federal clean water act and the Maryland water quality improvement act: the rational for developing a water and nutrient management planning process for container nursery and greenhouse operations. J. Environ. Hort. 19: 226-229. Lea-Cox, J.D., Ross, D.S. and Teffeau, K.M. 2001. A water and nutrient management planning process for container nursery and greenhouse production systems in Maryland. J. of Environmental Horticulture. 19: 230-236. Leith, J.H. and D.W. Burger. 1989. Growth of Chrysanthemum using an irrigation system controlled by soil moisture tension. J. Amer. Soc. Hort. Sci. 114: 387-397. MacDonald, J. D., Ali-Shtayeh, M. S., Kabashima, J., and Stites, J. 1994. Occurrence of Phytophthora species in recirculated nursery irrigation effluents. Plant Disease 78:607-611. McPherson, G. M., Harriman, M. R., and Pattison, D. 1995. The potential for spread of root diseases in recirculating hydroponic systems and their control with disinfection. Med. Fac. Landbouww. Univ. Gent. 60 (2b):371-379. Meisinger, J.J. and J.A. Delgado. 2002. Principles for managing nitrogen leaching. J. Soil Water Conserv. 57:485-498. Merhaut, D.J., E.K. Blythe, J.P. Newman, and J.P. Albano. 2006. Nutrient Release From Controlled-release Fertilizers in Acid Substrate in a Greenhouse Environment: I. Leachate Electrical Conductivity, pH, and Nitrogen, Phosphorus, and Potassium Concentrations. HortScience 41(3):780-787. Nelson, P.V. 2008. Greenhouse operation and management (7th ed.). Prentice Hall, NJ. Nemali, K.S. and M.W. van Iersel. 2008. Physiological responses to different substrate water contents: screening for high water-use efficiency in bedding plants. Journal of the American Society for Horticultural Science 133:333-340. Nemali, K.S. and M.W. van Iersel. 2006. An automated system for controlling drought stress and irrigation in potted plants. Scientia Horticulturae 110:292297. Newman, J.P., J.P. Albano, D.J. Merhaut and E.K. Blythe. 2006. Nutrient release from controlled-release fertilizers in a neutral-pH substrate in an outdoor environment: I. Leachate electrical conductivity, pH, and nitrogen, phosphorus, and potassium concentrations. HortScience 41(7):1674-1682. Nieder, R., Schollmayer, G. and Richter, J. 1989. Denitrification in the rooting zone of cropped soils with regard to methodology and climate: A review. Biology and Fertility of Soils. 8: 219-226. Nielsen, C. J., Ferrin, D. M., and Stanghellini, M. E. 2006. Cyclic production of sporangia and zoospores by Phytophthora capsici on pepper roots in hydroponic culture. Can. J. Plant Pathol. 28 (3):461-466. Niemiera, A.X., 1994, Fertilizers: Minimizing use and maximizing growth of container and field-grown plants, p.105-115. In: C. Heuser and P. Heuser (eds.) Recycling and resource conservation: A reference guide for nursery and landscape industries, Pennsylvania Nurserymens Association, Inc. Harrisburg, Pennsylvania. Ogg, C.W, and G.A. Keith. 2002. New federal support for priority watershed management needs. J. Amer. Water Resources Assoc. 38:577-586. Powell, C.C. and R.K. Lindquist. 1997. Ball pest & disease manual: Disease, insect, and mite control on flower and foliage crops. 2nd ed. Ball Publishing, Batavia, Ill. Prenger, J.J., P.P. Ling, H.M. Keener, R.C. Hansen. 2005. Plant response-based irrigation control system in a greenhouse: system evaluation. Transactions of the ASAE 48(3):1175-1184. Riley, M. B., R. J. Keese, N. D. Camper, T. Whitwell and P. C. Wilson. 1994. Pendimethalin and oxyfluorfen residues in pond water and sediment from container plant nurseries. Weed Tech. 8:299-303. Ristvey, A.G. 2004. Water and Nutrient Dynamics in Container-Nursery Production Systems. Natural Resource Sciences and Landscape Architecture. University of Maryland. College Park, MD. Ph. D. 254 pp. Ristvey, A.G., Lea-Cox, J.D. and Ross, D.S. 2007. Nitrogen and phosphorus uptake efficiency and partitioning of container-grown azalea during spring growth. J. of the Amer. Society of Horticultural Sci. 132: 563-572. Ristvey, A. G., J. D. Lea-Cox and D.S. Ross. 2004. Nutrient Uptake, Partitioning and Leaching Losses from Container-Nursery Production Systems. Acta Hort. 630:321-328. Ritchie, G.L., C.W. Bednarz, J. Whitaker, and C. Mills. 2005. Cotton irrigation timing using remote sensing. Proc. 2005 Beltwide Cotton Conf. 89-94. Rose, M.A. 1999. Nutrient use patterns in woody perennials: Implications for increasing fertilizer efficiency in field-grown and landscape ornamentals. HortTechnology. 9: 613-619. Ross, D.S. 2008a. Containment basin design. In: Green Industry Knowledge Center for Water and Nutrient Management Learning Modules. J. D. Lea-Cox, D. S. Ross. and C. Zhao. (Eds.). University of Maryland, College Park, Maryland. Published online at http://www.waternut.org/moodle/course/view.php?id=29. Ross, D.S. 2008b. Irrigation system audits. In: Water and Nutrient Management Learning Modules J.D. Lea-Cox, D.S. Ross and C. Zhao (Eds) University of Maryalnd, College Park, Maryland. Published online at http://www.waternut.org/moodle/course/view.php?id=26. Ross, D.S. 2008c. Irrigation system design and components In: Green Industry Knowledge Center for Water and Nutrient Management Learning Modules. J. D. Lea-Cox, D. S. Ross, and C. Zhao. (Eds.). University of Maryland, College Park, Maryland. Published online at http://www.waternut.org/moodle/course/view.php?id=19. Ross, D.S., J.D. Lea-Cox, and K.M. Teffeau. 2002. The importance of water in the nutrient management process. Proc. S. Nursery Assoc. Res. Conf. 46:574-577. Skimina, C.A. 1986. Recycling irrigation runoff on container ornamentals. HortScience 21:32-34. Stanghellini, M. E., and Miller, R. M. 1997. Biosurfactants: their identity and potential in the biological control of zoosporic plant pathogens. Plant Disease 81:4-12. Stanghellini, M. E., Kim, D. H., Rasmussen, S. L., and Rorabaugh, P. A. 1996a. Control of root rot of peppers caused by Phytophthora capsici with a nonionic surfactant. Plant Disease 80:1113-1116. Stanghellini, M. E., Rasmussen, S. L., Kim, D. H., and Rorabaugh, P. A. 1996b. Efficacy of nonionic surfactants in the control of zoospore spread of Pythium aphanidermatum in a recirculating hydroponic system. Plant Disease 80:422-428. Stanghellini, M. E., and Rasmussen, S. L. 1994. Hydroponics: A solution for zoosporic pathogens. Plant Disease 78:1129-1138. Tyler, H.H., S.L. Warren and T.E. Bilderback. 1996. Reduced leaching fractions improve irrigation use efficiency and nutrient efficacy. J. Envrion. Hort. 14:199-204. Ullah, S. and G. M. Zinati. 2006. Denitrification and nitrous oxide emissions from riparian forests soils exposed to prolonged nitrogen runoff. Biogeochemistry 81:253-267. USDA. 1994. 1992 Census of agriculture. USDA, Washington, D.C. USDA. 2004. 2002 Census of agriculture. USDA NASS, Washington, D.C. USDA. 2007. Nursery crops 2006 summary. USDA NASS, Washington, D.C. von Broembsen, S. L., and Wilson, S. K. 1998. Occurrence of Phytophthora spp. in nursery runoff and recycled irrigation water. Phytopathology 90:S92. Warsaw, A.L., R.T. Fernandez, B.M. Cregg and J.A. Andresen. 2009a. Container-grown ornamental plant growth and water runoff nutrient content and volume under four irrigation treatments. HortScience 44:1308-1318. Warsaw, A.L., R.T. Fernandez, B.M. Cregg and J.A. Andresen. 2009b. Water conservation, growth, and water use efficiency of container-grown woody ornamentals irrigated based on daily water use. HortScience 44:1573-1580. White, G. C. 1999. Handbook of chlorination and alternative disinfectants. J. Wiley-Interscience, New York. Willis, G.H. 1982. Review: pesticides in agricultural runoff and their effects on downstream water quality. Environ. Tox. Chem. 1:267-279. Wilson, C., T. Whitwell and M.B. Riley. 1996. Detection and dissipation of isoxaben and trifluralin in containerized plant nursery runoff water. Weed Science. 44:683-688. Yeager, T.H. 2008. Capture and recycling of irrigation water. In: Green Industry Knowledge Center for Water and Nutrient Management Learning Modules. J. D. Lea-Cox, D. S. Ross. and C. Zhao. (Eds.). University of Maryland, College Park, Maryland. Published online at http://www.waternut.org/moodle/course/view.php?id=21. Yeager, T., T. Bilderback, D. Fare, C. Gilliam, J. Lea-Cox, A. Niemiera, J. Ruter, K. Tilt, S. Warren, T. Whitwell, and R. Wright. 2007. Best management practices: Guide for producing nursery crops. 2nd ed. Southern Nursery Assn., Atlanta. Yeager, T.H., Gilliam, C.H., Bilderback, T.E., Fare, D.C., Niemiera, A.X. and Tilt, K.M. 1997. Best management practices guide for producing container-grown plants. Southern Nursery Association, Marietta, GA. Yeager T., R. Wright, D. Fare, C. Gilliam, J. Johnson, T. Bilderback, and R. Zondag. 1993. Six state survey of container nursery nitrate nitrogen runoff. J. Environ. Hort. 11:206-208.