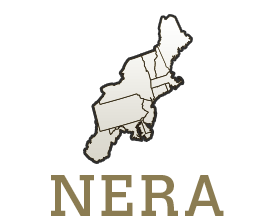
NE144: Forage Crop Genetics and Breeding to Improve Yield and Quality
(Multistate Research Project)
Status: Inactive/Terminating
NE144: Forage Crop Genetics and Breeding to Improve Yield and Quality
Duration: 10/01/1997 to 09/30/2002
Administrative Advisor(s):
NIFA Reps:
Non-Technical Summary
Statement of Issues and Justification
Forage crops constitute the foundation of livestock and dairy enterprises in the United States and Canada while also serving vital environmental functions such as soil conservation, nitrogen fixation and reduced need for tillage. Improving forage crop cultivars provide economic opportunities for integrating livestock/crop production systems that promote more stable, sustainable agriculture systems. Selective breeding and genetic engineering programs can improve pest resistance, annual persistence, and nutritional quality of forage crops without reduction in yields, as well as increase forage crop/livestock productivity without increasing seed costs or management input costs to the farmer.
Related, Current and Previous Work
Formal forage breeding efforts began just over 100 years ago (Hays, 1892; Smith, 1948; Beddows, 1953). Early cultivars were developed by intentional (Hays, 1892; Jenkin, 1943) or unintentional (Levy, 1932; Evans, 1937) selection for morphological or sexual reproductive traits. Despite rapid breeding progress and successful development of numerous improved cultivars of several species, these cultivars were limited in their breadth of adaptation. Cultivars exported from Europe to North America rapidly evolved in response to new environments and management systems (Levy, 1932; Evans, 1937). These types of changes have been shown to be genetically controlled in several species (Van Dijk, 1955; Cooper, 1959; Hayward, 1970). These changes can happen rapidly enough to cause significant, detrimental, and irreversible shifts in cultivar performance during the seed multiplication process (Evans et al., 1961; Crossley and Bradshaw, 1968). Numerous environmental factors (e.g., soil mineral contents or pH, ambient temperature, photoperiod, and moisture availability) may regulate these genetic shifts (see review by Snaydon, 1978).
Evaluation of breeding lines at multiple locations throughout a geographic region is the best means to develop cultivars with wide adaptation. Evaluation over a wide range of sites within or between regions is also useful in identifying the most appropriate sites for future tests (Barker et al., 1981). Additionally, some environments may be more conducive to selection progress than others (Snaydon; 1978; Casler and Walgenbach, 1990). However, many forage cultivars currently bred in Europe and North America are products of selection and breeding conducted at a narrow range of locations. The ultimate release and recommendation of a cultivar are often based on the results of a regional testing program, but are also frequently based on educated guesses and extrapolation on the part of the breeder. 'Saratoga' smooth bromegrass, perhaps the most broadly-adapted cultivar of this species based on high yield in many diverse environments (Caster and Ehlke, 1986) is a product of regional testing prior to synthesis and testing of the cultivar per se. Without regionwide interaction among breeders, the coordination necessary to develop broadly adapted cultivars cannot occur.
Germplasm collection and incorporation into breeding programs remains an important objective of most forage breeding programs (Breese and Tyler, 1986; Rumbaugh et al., 1988; Caster, 1991). Because forage breeding is a relatively young discipline, forage crops are generally not as domesticated as most cereal crops (Harlan, 1975). Thus, collections of naturalized populations in many species have similar phenotypic characteristics as cultivated germplasm (Breese and Tyler, 1986; Rumbaugh et al., 1988; Caster, 1991). Because introduced germplasm is adapted to some very specific environments, in the case of natural collections (Snaydon, 1978; Rumbaugh et al., 1988), or diverse production environments and management systems, in the case of cultivated germplasm from other countries, superior germplasm for use in breeding programs requires identification in a wide range of target environments. Germplasm evaluations in a wide range of environments can be extremely useful in identifying the breadth of adaptation of individual accessions as well as environmental factors responsible for differences in performance or adaptation (see review by Snaydon, 1978). This information is important for developing broadlyadapted cultivars and for identifying sites and environmental characteristics useful as targets for future collection. It can also be used to document agronomic improvements in forage crops due to breeding, information that is generally unavailable in most species.
Genetic improvement of forage yield is extremely difficult and slow, due to low heritability and high genotype x environment interaction (GEI) (Barker and Kalton, 1989). Thus, many breeders have developed new selection criteria for indirect forage yield improvement, e.g., leaf area expansion rate (LAER) in tall fescue (Sleper and Nelson, 1989), specific leaf weight in reed canarygrass (Carlson, 1990), and leaf blade length in perennial ryegrass (Rhodes, 1969). Low LAER tall fescue populations are best adapted to frequent cutting and perhaps a pasture management, while high LAER populations are best adapted to infrequent cutting (Sleper and Nelson, 1989). Long-leaf and short-leaf ryegrass populations behaved similarly to the high-LAER and low-LAER tall fescue populations, respectively (Rhodes, 1969).
For successful pasture production, cultivars must be tested for grazing tolerance. The best method to select and evaluate for grazing tolerance is by directly using animals, but the cost and difficulty of maintaining animals prohibits some researchers from using them. Mechanical mowers have been used by some researchers, but they "select" passively, i.e., they harvest everything above the height at which they are set and do not contribute damage from hooves and mouths. Animals on the other hand harvest selectively (Woledge et al., 1992). Cattle are less selective and less detrimental to white clover than are sheep (Evans et al., 1992) and rotational stocking is less harmful than continuous stocking (Sheath and Hodgson, 1989). Thus, it is possible to select the level of defoliation pressure by manipulating the animals and management used. Bouton et al. (1991) showed that grazing-tolerant alfalfa cultivars could be selected by intense grazing by cattle. Morphological and physiological traits are often radically different for high-performing pasture cultivars vs. high-performing hay-type cultivars (Stapledon, 1928; Jenkin, 1943; Breese and Tyler, 1986). Further studies showed that several morphological and physiological traits were associated with alfalfa grazing tolerance (Brummer and Bouton, 1991, 1992). Further research on traits and selection methodology is needed for other species. Regional collaboration will be an essential requirement for the development of cultivars adapted to relatively new management systems, such as intensive, rotational grazing.
There is conflicting evidence whether optimal grass-legume mixtures for long-term production of high-quality hay or pasture require breeding efforts under mixed-stand conditions. Some studies suggest that grass and legume cultivars may be matched according to vigor and maturity classifications (see review by Zannone et al., 1986). Others suggest that the mixtures with the highest forage yield are those that- have been selected under conditions that closely approximate the ultimate use and companion population (Evans et al., 1985; 1989). Persistence and/or productivity of perennial ryegrass, intermediate ryegrass, and smooth bromegrass in mixtures has been increased as a direct result of selection under mixture conditions (Van Dijk, 1983; Casler, 1988; Consigli, 1991). In white clover, stolon branching is an important component of vegetative persistence, especially under grazing, but stolon branching of white clover in monoculture is not predictive of stolon branching in a white-clover, grass mixture (Brink and Rowe, 1993). Predicted response to selection for plant spread in grass was 71 to 90% more effective than selection in monoculture (Rowe and Brink, 1993). Even forage yield in monoculture does not predict forage yield in mixtures, especially when measured over several years (Caradus et al., 1989). Continued selection and germplasm development under mixture conditions will be required to determine the relative merits of these two approaches of developing forage mixtures.
Little is known about the relationship between forage and seed traits of most forage species. Generally, seed is produced in dedicated environments which differ dramatically from those typical of most forage production. Thus, breeding for improved seed yield in forage environments, where most forage breeding programs are located, is often unsuccessful due to high GEI (Stratton et al., 1989). Overcoming this limitation requires either (1) collaborative research between scientists in both types of environments, (2) contract evaluations (such as those formerly provided by NC-83), or (3) an increase in our knowledge of the relationship between forage and seed yield and the genetic traits and environmental factors governing that relationship.
Persistence of forage stands for greater than 2 or 3 years is a major problem limiting use of several productive, high quality species, such as red clover (Smith and Kretschmer, 1989), birdsfoot trefoil (Miller et al., 1983), and several ryegrass species (Casler, 1988; Casler and Walgenbach, 1990). The ability of a plant or stand to persist for many years is governed by its resistance or tolerance to a multitude of stresses, both biotic and abiotic. Sometimes, improvements in resistance or tolerance to one stress factor can result in significant improvement in stand longevity (see review by Smith and Kretschmer, 1989). Persistence of some species has been improved by selection for survival in harsh environments (Caster, 1988; Consigli, 1991). However, more work needs to be conducted to determine the specific traits responsible for genetic improvement of persistence (Caster, 1988). For some species and for some stresses, research is still required to identify appropriate selection criteria and methodologies.
In the private forage breeding sector of the U.S., selection for increased disease resistance is a major component of alfalfa cultivar development programs, but very limited work on diseases is conducted on other forage crops (Brummer and Moutray, 1996). In birdsfoot trefoil, Fusarium wilt has been implicated in stand losses in NY and MN (Gotlieb and Dorski, 1983; Murphy et al., 1985; Tillapaugh, 1995; Ehlke and Samac, pers. comm). Although Hill and Zeiders (1987) improved resistance to Fusarium wilt in a birdsfoot trefoil population, no resistant cultivars have been released. Mycoleptodiscus terrestris, first identified by Gerdemann (1953), has been isolated from forage legumes in MO (Pettit et al., 1966, 1969), eastern USA (Carroll and Whittington, 1991), and WI (C.R. Grau and R R. Smith, pers. comm., 1994). The root-lesion nematode (Pratylenchus penetrans) causes serious damage to birdsfoot trefoil in Nova Scotia and other regions of North America (Willis et al., 1976). Papadopoulos et al. (1994) demonstrated significant variability among trefoil populations for resistance. A host of disease problems exist on the forage grasses as well (Braverman, 1986). In order to adequately develop widely adapted cultivars of forages that incorporate resistances to a range of pathogens, collaboration among public sector researchers is clearly needed.
Lack of winterhardiness is a particular problem in the north central United States and Canada. If the cultivated germplasm of a given species does not have sufficient cold tolerance or winterhardiness, wild or unimproved germplasm often does. Medicago sativa ssp. falcata, yellowflowered alfalfa from Siberia, and Russian collections of orchardgrass are sources of winterhardiness for these species (Barnes et al., 1972; Kalton, pers. comm.). Because selection for cold hardiness requires harsh winters, the intensity of selection will vary year to year at any location. For material from areas where cold winters are not common, testing in more northerly areas may result in greater gains in winterhardiness.
Ideas relating to improved forage quality have changed as animal nutritionists have learned more about the complexities of nutrition. Protein is considered one of the major contributions of most legumes to animal nutrition if the forage is managed and stored correctly. Protein concentration is highly heritable and can be improved readily by breeding (Clements, 1969; Phillips et al., 1982; Coors et al., 1986). Perhaps a more important objective is to alter the proteins in forage legumes to make them more resistant to rumenal degradation (i.e., increasing `bypass protein') so that nitrogen is less likely to be lost as ammonia (Broderick and Buxton, 1991). Skinner et al. (1994) found variability among alfalfa germplasm sources for protein degradability. Alternatively, plant protein utilization could by improved by increasing the amount of rapidly degraded carbohydrate in the forage so that energy would not be limiting to rumen microbes (Stokes et al., 1991a, b). Pectic polysaccharides represent a potential source of rapidly degraded carbohydrates (Gradel and Dehority, 1972; Van Soest, 1982, 1995), and a significant portion of the total structural polysaccharides in forage legume cell walls are pectic materials (Titgemeyer et al., 1991; Hatfield, 1992). Hatfield and Smith (1995) measured the concentration of uronosyls, pectic sugars and pectin in leaf and stem walls of alfalfa, red clover, and birdsfoot trefoil and found significant differences among and within species. Another means to increase available carbohydrates is to increase digestibility of fiber by breeding for lower lignin:NDF ratio. Lowering lignin:NDF ratio could maintain total fiber concentration while increasing the availability of carbohydrates in forage.
Breeding for improved forage quality (small changes in digestibility) in three grass species had a significant positive impact on animal performance and consequently economic returns (Eichhorn et al., 1986; Anderson et al., 1988, Moore et al., 1995). Jung (1996) reported that just small shifts toward more digestible fiber in alfalfa resulted in significant increases in milk production by dairy cows. Continued research on divergent selection for forage quality traits in other species, followed by laboratory evaluation and animal performance trials, will be necessary to confirm the animal
response to increased forage quality. Buxton and Caster (1993) and Rhodes (1985) concluded there is evidence that lignin and associated phenolics are involved in providing plant resistance to diseases, insects, cold temperatures, and other environmental stresses in various plant species. However, in smooth bromegrass and switchgrass, increased digestibility did not result in changes in forage yield or lodging potential (Vogel et al., 1981; Casler and Ehlke, 1986), despite large reductions in lignin concentration for smooth bromegrass. Further research into the structural and nonstructural roles of lignin is warranted. Although increased disease resistance increases forage quality in the presence of the pathogen (Catherall, 1987; Kam et al., 1989), the effects of genetically improved forage quality on pest resistance and stress tolerance are not clearly delineated.
The concept of progeny testing as a selection tool in forage crop breeding has been in existence since the 1890s (Frandsen, 1991). It was refined and put to use by considerable research at numerous breeding stations during the 1950s (see review by Hanson and Carnahan, 1956). Both polycross and testcross procedures have been proposed and compared, on a theoretical basis, with phenotypic selection which is selection based on individual plant performance per se (Empig et al., 1972; Hill and Haag, 1974). Although empirical comparisons have been made among selection methods for disease resistance in alfalfa (Haag and Hill, 1974; Hill and Leath, 1979), they have not been made for other traits of other species. Contrary to expectations for traits controlled by some dominant genes, high-performance testers did not differ from low-performance in empirical evaluations of the testcross procedure (Voigt, 1968; Frandsen, 1989). Further research is needed to define the limitations and practical uses of both phenotypic and progeny test selection methods. We also need to use both existing theory and empirical selection studies to improve the efficiency of breeding systems, thereby increasing the rate of genetic progress.
In the past decade, molecular markers have become widely used for genetic mapping and breeding methodology studies of agronomic crops (Phillips and Vasil, 1994). Molecular marker work on forages has been scattered among numerous species with little in-depth work on any species other than alfalfa and tall fescue (Phillips and Vasil, 1994; Casler et al. 1996). Molecular markers could be used to augment breeding efforts in two ways: (1) through genetic mapping and markerassisted selection or (2) through diversity analyses for parental selection or varietal typing. Genetic linkage maps provide a framework for gene localization and open the potential of marker-assisted breeding of both qualitative and quantitative traits. Linkage maps have been constructed in alfalfa (Brummer et al., 1993; Kiss et al., 1993; Echt et al., 1994; Tavoletti et al. 1995), tall fescue (Xu et al., 1995), and eastern gamagrass (Blakey, 1993). Marker facilitated selection, which could be practiced in the seedling year, could greatly speed the forage breeding process. Other molecular marker work has focussed on assessing variability within and among populations (Brummer et al., 1991; Yu and Pauls, 1993; Huff et al., 1993; Kidwell et al., 1994; and Xu et al., 1994) and on varietal identification (Huff, 1996; Barker, 1996). Markers may potentially be useful for the selection of parental plants having alleles that maximize heterosis (Brummer et al., 1994), similar to work on heterotic groups in maize (e.g., Lee et al., 1989) and oilseed rape (biers et al., 1996). Obviously more work remains to be done to assess the utility of molecular markers to forage breeding projects. Collaborative agreements are essential to enable the wide area testing required to associate markers with phenotypes across several environments. Region-wide cooperation also allows breeders without access to laboratory facilities to interact with others who have molecular marker capabilities.
Objectives
-
Identify plant characters appropriate for use as selection criteria for genetically improving stability, quality, and productivity of hay, silage, and pasture crops.
-
Develop and modify breeding methods for use in improving broadly-adapted germplasm of forage crops.
-
Develop and evaluate experimental populations and cultivars for use in sustainable, integrated, crop-livestock production systems.
-
Methods
Objective No. 1: Identify plant characters appropriate for use as selection criteria for genetically improving stability- quality. and productivity of hay, silage, and pasture crops.1.1 Birdsfoot Trefoil
1.1.1. Identification of pathogens useful for developing birdsfoot trefoil germplasm with multiple pest resistance.
Cooperating locations: NY`, USDFRC, AFCN, MN.
During the 1993 technical committee meeting of the NE-144 Regional Cooperative Research Project, breeders indicated that specific pathogens that reduced productivity and stand life of birdsfoot trefoil recently had been identified in different areas of North America. Because of limited resources for breeding birdsfoot trefoil, each breeder cannot embark on a new breeding program for every disease resistance. Plans were developed to cooperate in breeding birdsfoot trefoil with multiple disease resistance.
The table below lists the cooperators and the pathogens) isolated from each location.
Location | Breeder | Pathologist | Pathogen |
NY | D.R. Viands | G.C. Bergstrom | Fusarium oxysporum |
USDFRC | R.R. Smith | C.R. Grau | Mycoleptodiscus terrestris |
AFCN | Y.A. Papadopoulos | J. Kimpinski | Pratylenchus penetrans |
Each cooperator will conduct recurrent phenotypic selection for resistance to the disease identified at his/her location. Selection will be done within a source population from each of the four locations. Source populations will be kept separate until three to four cycles of recurrent selection have been completed. Most cooperators currently are in the second cycle of selection. At the completion of recurrent selection, the progress from selection for resistance to each of the diseases will be determined. Any correlated responses from selection for resistance to these diseases will also be evaluated.
1.1.2. Introgression of rhizomes into improved populations. Cooperating locations: PGRU, AFCN, MN, NY, USDFRC.
Rhizomes have recently been identified in wild germplasm from Morocco. The trait is dominant and simply inherited, making it easy to transfer from wild to domesticated (improved) populations and cultivars. Further study is needed to determine whether rhizomes will provide any advantages to domesticated birdsfoot trefoil. The PGRU (lead) location maintains the wild Moroccan germplasm and will either cross them to birdsfoot trefoil populations developed by the cooperating stations or send vegetative propagules of the wild germplasm to allow cooperating stations to produce their own crosses.
The rhizomatous populations will be further improved by selection and will be tested regionally to determine their agronomic value to forage production and persistence. If rhizomes are a valuable
` See list of project leaders for key to abbreviations. The lead location is listed first. For all projects, the lead location will be responsible for coordinating annual progress reports, data summarization, and scientific publications. asset to domesticated birdsfoot trefoil, this research will enhance their distribution among genetically diverse populations with potential for commercialization.
1.2 White Clover
Identification of white clover traits important for grass compatibility and broad adaptation. Cooperating locations: ASWCRL, IA, PSWMRL, AFCC.
White clover, Trifolium repens L., is the primary pasture legume in northeastern (NE) and north central (NC) USA, where it grows in mixtures with grasses and other plants. Despite its importance, little white clover breeding has been conducted in the NC and NE regions in the past several decades. As a result, a collaborative effort has been developed to evaluate and select germplasm with the long-term objective of developing and releasing a cultivar with broad geographic adaptation.
We will study five experimental populations of white clover derived from pasture collections in Iowa, Pennsylvania, West Virginia, Wisconsin, and Prince Edward Island and compare them with Brown Loam, a germplasm from Mississippi. Seedlings of all six populations will be grown in the greenhouse and plants transplanted into an established or establishing sod of orchardgrass in central Iowa, central Pennsylvania, and southern West Virginia. The experiment will be initiated in 1997, assuming adequate seed of all germplasm are available by February 1, 1997. If adequate seed are not available at that time, establishment of the study will be delayed until 1998. The plot areas will be limed and fertilized with P and K to meet soil test recommendations. Plots will be single rows of 25 plants spaced 1 m apart with plants spaced 0.5 m apart within rows. Two separate, but identical plantings will be established: one will be managed by grazing, sheep or cattle as available, and the other by mowing. Four replications will be included within each planting.
Establishment year management: Before transplanting the plot areas will be examined for the presence of wild clovers. These will be removed by spraying prior to transplanting. Following transplanting, cooperators will manage the plots as appropriate for weed control, survival of the clover transplants, and survival of the companion grass. Data as described below for clover plant height, spread, and density will be collected prior to a late summer mowing (late August to early September). If time is available, leaf size measurements will also be made. Stolon density counts will be obtained following the late summer mowing.
Harvest years management: Mowed plots will be clipped to a height of 8 cm each time canopy height reaches 20 cm. Grazed plots will be grazed each time canopy height reaches 20 cm. If uniform defoliation has not been achieved by the end of a grazing period, the grazed plot will be mowed to a uniform height of 6 cm. Individual white clover plants will be measured for plant height, and visually rated for spread and density prior to the first spring harvest, before a late June or early July (early summer) harvest, and before the final fall harvest. Stolon density will be estimated following the final fall harvest by counting the number of stolons transecting a 15 cm arc
on each side of the row (900 from the direction of the row) and 15 cm from the point where each plant was transplanted. Leaf size will be measured once each year, preferably prior to the early summer harvest. Leaf size of each plant can be obtained by measuring the leaf area of 10 fully expanded leaves of each plant. Dry matter yields of each plot in the mowed study will be measured. Clover percentage will be estimated visually.
Data will also be collected on other traits that appear useful for selection. Additionally, RAPD marker data will be supplied for these plants (PSWMRL). Cooperators are urged to remain in contact via e-mail so that changes in procedures and changes to data collection can be handled as uniformly as possible across locations. The study will last for at least two harvest-years. At the end of the study, a cooperative experiment will be initiated to select plants based on the results of this experiment, with the goal of developing a cultivar with broad adaptation.
1.3 Forage Quality
1.3.1. Selection for improved cell wall pectin concentration in alfalfa. Cooperating locations: NY, USDFRC.
New York and USDFRC are cooperating to develop breeding methods for improving nutritive quality of alfalfa for livestock. Different techniques for assaying forage pectin concentration recently were developed at these two locations. New York just initiated research to determine heritability and to conduct recurrent phenotypic selection for increasing concentration of forage pectin. At USDFRC, cell wall pectin concentration of alfalfa, red clover, and birdsfoot trefoil leaf and stem tissue was determined using laboratory procedures. Using this chemical information and spectral data from near infrared reflectance spectroscopy (NIBS), equations have been developed to predict cell wall pectin concentration of leaves and stems of these three species. The equations generated for alfalfa were used to predict the pectin concentration of leaf and stem cell walls of 64 alfalfa plants previously selected for winter survival. From these predictions, five groups were formed: high and low leaf pectin, high and low stem pectin, and high leaf and stem pectin. Three to four plants representing each group were intercrossed. The subsequent polycross progeny from these crosses and the respective parents have been established in the field and will be evaluated for cell wall pectin concentration. The objective is to develop populations with high and low leaf pectin and high and low stem pectin to validate the effect of altered cell wall pectin concentration on animal nutrition. These polycross progeny will also be evaluated for ADF, NDF, ADL, protein concentration, digestibility, leaf-to-stem ratio, etc. to determine correlated responses from selection for cell wall pectin concentration.
1.3.2. Selection for altered fiber constituent ratios. Cooperating locations: NY, AFCSF.
Recurrent phenotypic selection for fiber ratios (higher hemicellulose:ADF and lower lignin:[cellulose + hemicellulose]) is being conducted in alfalfa (NY) and timothy (AFCSF) in an attempt to increase energy availability to dairy animals. After each cycle of selection, seed quantities will be increased and planted in plot trials at multiple locations to determine selection progress. After two years in the field, plants will be selected for lower ADL:(hemicellulose + cellulose), lower ADL:cellulose, and higher hemicellulose:cellulose. Populations developed by these selection criteria will be evaluated for selection progress and ultimately for effect on dairy cow performance. Comparisons of the gain from selection and the significance of fiber alterations will be made between alfalfa and timothy.
1.3.3. Interactions of nonstructural carbohydrates, seed yield, forage quality and yield
components.
Cooperating locations: WV, KY, NFSPRC.
Tall fescue populations and clones with a range of total nonstructural carbohydrate (TNC) concentrations will be identified. Correlations among TNC, seed and forage yields, and forage quality components will be investigated. The studies may include entries of related species and derivatives such as perennial ryegrass, meadow fescue, and festulolium.
Four replications of selected populations and/or clones will be planted in swards and/or space plant nurseries and analyzed for TNC, seed yield, forage yield, and forage quality components. Inflorescences will be harvested at the late milk to soft dough stage of maturity and analyzed for TNC. The plots used for inflorescence sampling will be harvested for seed yield. The purpose of these studies is to determine whether TNC in the inflorescences during seed filling can predict seed yield at three locations (WV, KY, and OR). Data are available which show differing levels of TNC in inflorescences of cultivars at the milk to soft dough stage. Cultivars with varying seed yield potential (as demonstrated in Oregon seed production environments) will be analyzed for differences in TNC in inflorescences.
Near infrared reflectance spectroscopy will be used to estimate crude protein and other forage characters such as ADF, NDF, lignin, and IVDMD of forage harvested from plots of these populations. The information obtained from these studies will be used to build synthetic populations with divergent levels of TNC concentrations. These new populations can be used to test the role of TNC concentration in palatability, winterhardiness, and persistence.
1.4 Alfalfa
Identification of traits useful for improving M. saliva ssp. falcata germplasm. Cooperating locations: IA, AFCSF, NGPRL, WP, SD, KS.
Several studies in recent years using isozymes and molecular markers identify M. saliva ssp. falcata as a likely source of new, or at least underutilized, genetic variation for alfalfa breeding. M. saliva ssp. falcata has several traits that make its improvement appealing in addition to the possible addition of new heterotic blocks, e.g., persistence under adverse conditions of cold winter and overgrazing. M. saliva ssp. falcata has long been recognized as an ideal legume for rangeland conditions. Alfalfa cultivars different from the traditional ones used for high quality dairy hay could be developed for beef cow-calf producers to use in permanent pastures. The winterhardiness of M. saliva ssp. falcata also could be useful in improving the winter survival of alfalfa cultivars. However, very little work has been conducted to improve M. saliva ssp. falcata for agronomic traits, and unless such work is initiated, the germplasm will likely be of little use in the future.
We plan to evaluate tetraploid M. saliva ssp. falcata accessions. Of the approximately 185 accessions currently available from the USDA PI station, about 120 are tetraploid. However, some of these accessions are purple flowered indicating that some contamination occurred during seed increase or that they were misclassified when collected. In addition to the PI material, several germplasms of falcata material have been developed and will be included: WISFAL (E.T. Bingham), C-25 (C. Townsend), SD, IA. A space planted nursery including 96 of the tetraploid, yellow-flowered accessions with 10 plants in each of two replicates will be established at all locations in 1997.
Transplants will be spaced one foot apart in 30" rows. The following visual ratings will be made on each accession row: (1) vigor several times during 1997 and 1998, (2) flower color, (3) growth habit (upright vs. prostrate), (4) leafiness, (5) disease incidence, (6) stem number, (7) crown diameter, (8) winter survival, (9) recovery after cutting and after winter, and (10) fall growth. In 1999, superior plants will be selected as parents for improved populations. Stem cuttings will be made of the selected plants and the seed increase will be conducted in isolation in Washington or Idaho. Each cooperator will maintain his own population. The initial work will concentrate on yellow flowered accessions to maintain as many falcata alleles as possible. This material will need to be introgressed into purple flowered saliva for adequate levels of commercial seed production and disease resistance.
1.5 Molecular Markers
Identifying molecular markers useful for forage improvement. Cooperating locations: IA, KY, NFSPRC, MN, PSWMRL.
Molecular markers, RFLP, RAPD, AFLP, SSR, and isozymes, can provide estimates of genetic variation within and among plant populations in order to deduce genetic relatedness. Individually, markers can be useful as diagnostic or selection tools. When combined into a genetic map, molecular markers can be useful for monitoring introgression. The utility of molecular markers for breeding and selection in many forages is not clearly defined.
Populations of eastern gamagrass from OK, KS, IA, KY, and NC will be evaluated with molecular markers to determine the extent of variation present in native populations of this nutritious, high
yielding prairie grass. Plants will be maintained in either Ames, IA or Lexington, KY. RAPD and RFLP analysis will be conducted in IA, and isozymes in KY. Approximately 20 RAPD markers and 10 RFLP markers will be analyzed in addition to several isozymes. Data analysis will be done with assistance from Reed Barker at NFSPRC using several methods, including UPGMA, PAUP, and AMOVA, that will be compared for their usefulness. Results from this study will be used to develop populations based on marker diversity to determine if there is any benefit from using markers over conventional selection. Similar analyses will be conducted for the five white clover populations in objective 1.2 at PSWMRL.
Some reports have suggested a complete lack of genetic variation for winter hardiness in perennial ryegrass. Our program (MN) was interested in transferring winter hardiness from Festuca species into perennial ryegrass. Reciprocal crosses were made between perennial ryegrass, red fescue (F. rubra) and a fertile putative hybrid (between annual ryegrass (L. multiflorum) and red fescue). Seedling were evaluated for a number of traits, and 138 of the putative hybrids were selected for RFLP analysis to verify hybridization. Standard DNA extraction and RFLP techniques are being used. RFLP probes were obtained from a tall fescue genomic library developed by Dr. D. Sleper (Univ. Missouri). Putative hybrids including the annual ryegrass X red fescue cross are being screened. Currently all putative hybrids have been screened with at least one restriction enzyme and probe. To date, over 30 true hybrids have been identified.
Objective No. 2. Develop and modify breeding methods for use in improving broadly-adapted germplasm of forage crops.
2.1 Alfalfa
2. 1. 1. Phenotypic and genotypic selection methods for improving alfalfa forage yield. Cooperating locations: AFCC, AFCSF; NY, AFCN.
A selection experiment to compare the effectiveness of phenotypic selection, half-sib family selection, and testcross selection for forage yield was initiated in 1987 at AFCC (leader), NY and AFCSF. Each location conducted selection in their most elite alfalfa germplasm. All plant populations are being evaluated for progress from selection for three productions years at AFCC, NY, AFCSF, and AFCN. One more year of data collection is required at some locations. During 1998-99, data will be analyzed and a publication written.
2.1.2. Comparison of hill, row, and sward plots for alfalfa breeding. Cooperating locations: AFCSF, NY.
Another study was established at AFCSF and NY to compare hill and row plots to sward plots for forage yield. Data from three production years will be analyzed and published in 1997-98. Future cooperative research will be planned after the current data are analyzed.
2.1.3. Comparison of mass, S 1, and S2 selection in alfalfa. Cooperating locations: AFCSF, IA, NY, KS.
Phenotypic mass selection is commonly used for selection in alfalfa; however, it may not be the most efficient manner to increase yields. Early work in the US and Italy indicated that one or two generations of selfing prior to selection improved gain. However, more rigorous research needs to be conducted, particularly with current cultivars containing high pest resistance profiles. Deterioration in pest resistance may occur during the selfing generations. In this experiment, three populations will be selected using one (S1) or two (S2) generations of selfing prior to selection or using phenotypic mass selection to improve yield. The correlated responses of other traits, particularly disease resistances, will be measured in addition to yield after several cycles of selection. Each cooperating program will select within a different population adapted to their area.
2.2 Perennial Ryegrass and Orchardgrass
Ear-to-row pedigree selection to improve the efficiency of forage yield selection.
Cooperating locations: MN, PSWMRL (Dr. Clyde Berg, retired).
Modified ear to row selection was completed at the PSWMRL for forage yield in orchardgrass and perennial ryegrass. Evaluation of four cycles of MEER selection is currently underway at MN. Evaluation of the trials for yield will be completed in 1997 and the data written for publication.
Objective No. 3. Develop and evaluate experimental populations and cultivars for use in sustainable, integrated, crop-livestock production s, stems.
3.1 Kura Clover
Regional Evaluation of Kura Clover Cooperating locations: USDFRC, IA, SD, MN, KY, AFCSF, AFCN, WV, NY, KS.
Evaluation trials of coated and non-coated seed of four kura clover cultivars (Rhizo, Cossack, Endura, and KZ2), Alfagraze alfalfa, and Ladino white clover were established in 1995 in 15 locations (IL, IA, KS, KY, MN, NY, ND, AFCN, PA, PEI, AFCSF (2 sites), SD, WV and USDFRC). The objectives of the project are to: (i) determine the effectiveness of coating kura clover seed with Rhizobium and calcium on the establishment of the species, (ii) to evaluate the performance of the cultivars under a wide range of environmental conditions, and (iii) to provide information to the respective cooperators relative to the merits for conducting further breeding research. Initial stand establishment was determined at all locations and forage yield obtained in 6 locations during this establishment year. Trials were discontinued in 1996 in ND (winter killed), AFCC and PSWMRL (poor stand and weed pressures), and Beckley, WV (excessive weed pressure from white clover).
Procedures for 1997 and 1998: Forage yield data will be collected on all active trials in both 1997 and 1998. After the 1998 harvests, all data will be summarized and reported collectively to the scientific community. Data from this project will provide information on the adaptiveness of kura clover to a wide range of environments; the yield and longevity potential over a large geographic area; and the effect of initial coating kura seed with calcium and Rhizobium relative to stand establishment and production in subsequent years of growth.
3.2 Orchardgrass
Evaluation of convergent-divergent populations. Cooperating locations: NFSPRC, IA, KY.
Two cycles of selection for seed yield per panicle was done in OR similar to local selection done in the convergent-divergent (C/D) study of the last project. The same four populations, developed in WI, PA, IA, and MO, were used as the original GD study and the selection protocol was similar. Eight hundred plants of each population were established in the field, with selection before anthesis based on forage traits; i.e., vigor, freedom from disease, leafiness, agronomic appearance, etc. to reduce the population to 200 individuals, then a final selection based on seed yield per panicle to reduce the final population to 50 plants. Seed was increased of both CID selection cycles for each population so that the selection progress test could be done using first generation seed from the selection cycle.
Twenty populations are available for testing: four cycle 0 populations, eight C/D populations (cycle 1 and cycle 2), and eight OR selected populations (cycle l and cycle 2). Selection progress will be measured for seed production by planting the ?0 populations from the selection cycles along with six check cultivars (Ambassador, Benchmark. Dawn, Justus, Pennlate, and Potomac) at two locations in OR. Forage traits will be examined in trials using the same seed source and planted in WI, PA, IA, and MO, the sites of the original selection, and also in KY. Trials will be planted in spring 1997 with two years of harvest planned. Collaborators will prepare a research publication detailing the results of the experiment after the second harvest season.
3.3 Birdsfoot Trefoil
Evaluation of birdsfoot trefoil populations selected for resistance to crown- and root-rot complex using convergent/divergent selection.
Cooperating locations: PGRU, IA, NY, USDFRC.
Selection of birdsfoot trefoil for crown- and root-rot complex using convergent/divergent selection was initiated among eight locations in 1985 (PGRU, CA, IA, IL, MN, NY, SD and USDFRC). The base population for selection was MU-81 germplasm. Each space-planted field nursery was grown for two years, then surviving plants were dug and scored for extent and severity of necrosis. Selected individuals from the same location were intercrossed to produce seed for the next cycle of selection. The third and final cycle of selection was completed in summer 1996 at five locations (PGRU, IL, MN, NY, and USDFRC). The selections from each location have been propagated and planted in isolated seed production blocks at Columbia, MO and are also maintained in the greenhouse. Plans are to produce in isolation seed of 1.) populations from each location, and 2.) a composite population containing selections from all locations. An additional population will be synthesized as a composite population derived by bulking equal amounts of seed from each of the five isolated populations. Thus, by fall 1997 there should be seven populations for evaluating progress from selection.
The size and type of evaluation conducted will depend upon the amount of seed produced for each population. Minimally, the seven populations will be planted at four locations (PGRU, IA, NY, and USDFRC) in side-by-side space-planted replicated nurseries for comparisons against each other and the base population MU-81. Side-by-side plantings of lm wide swards will be established by direct seeding if sufficient seed is available. Planting will commence in spring 1998. Nurseries will be grown for two years and surviving plants dug and scored in spring 2000. Data collected will include: 1.) attrition recorded in spring and fall, 2.) vigor, 3.) crown- and rootrot score based on degree of necrosis, and possibly yield. Upon completion of the evaluation the cooperators will prepare a research manuscript describing the entire experiment.
3.4 Alfalfa
Evaluation of alfalfa populations developed for resistance to the alfalfa snout beetle. Cooperating locations: NY, AFCSF, SD, IA.
The alfalfa snout beetle (Otiorhychus ligustica) has caused severe productivity and stand losses in isolated areas of NY for several decades. The insect now is spreading rapidly across northern NY and threatens to spread into neighboring states. Resistance has not been found in current cultivars. Therefore, NY will develop a screening procedure and identify resistance or tolerance in plant introductions, beginning with the core collection. Data from previous experiments indicated that alfalfa with a fibrous root may tolerate alfalfa snout beetle feeding longer than plants with primarily a taproot. Therefore, populations are being developed with more fibrous and branched roots and for capability to regenerate roots after taproot severing. New York will cooperate with the other institutions by screening their breeding populations for resistance or tolerance. Improved populations with resistance or tolerance will be evaluated for yield and adaptation at cooperating locations throughout the northern U.S. and Canada.
Measurement of Progress and Results
Outputs
- Developed improved experimental populations of birdsfoot trefoil, orchardgrass and alfalfa expressing broad geographic adaptation, disease resistance and seed/forage yield.
- Evaluated alternate breeding methods for alfalfa, orchardgrass and perennial rye grass in order to identify the most effective methods for producing improved cultivars.
- Evaluated germplasm of alfalfa for agronomic qualities.
- Evaluated kura clover quality for adaptation as forage crop.
- More rapid selective breeding programs assisted by use of genetic markers.
- Develop genetic markers for desirable traits using mapping methods unique to polyploid plants.
- Determine if Klason lignin concentration is as accurate as acid detergent lignin concentration for predicting forage digestibility.
Outcomes or Projected Impacts
- Improved cultivars of forage crops. Improved digestibility of some forage crops leading to increases in nutrient conversion. Improved pest and disease resistance of forage crops.
- Improved winter-hardiness of cool season forage crops.
- Increased geographic adaptability of forage crops.
- More rapid adoption by farmers of integrated crop/livestock systems.
- More holistic, sustainable agricultural systems.