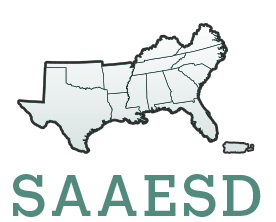
S1046: Improved management of plant-parasitic nematodes through modern diagnostic tools and increased use of host resistance
(Multistate Research Project)
Status: Inactive/Terminating
S1046: Improved management of plant-parasitic nematodes through modern diagnostic tools and increased use of host resistance
Duration: 12/01/2009 to 09/30/2014
Administrative Advisor(s):
NIFA Reps:
Non-Technical Summary
Statement of Issues and Justification
Plant-parasitic nematodes have been referred to as the 'Hidden Enemy' of crops because the losses resulting from these plant pests are often over-looked and under estimated. Yet nematodes are among the most wide-spread pests affecting crop production, especially in the southern United States where the warm climate and abundance of sandy soils provides an ideal environment for this group of pests. As a group, nematodes are estimated to cause 10-14% yield losses annually (Sasser & Freckman, 1987). They are especially important on cotton (Koenning et al., 2004), peanut (Dickson, 1998), soybean (Kinlock, 1998), turf (Crow, 2005), and numerous vegetable crops (Johnson, 1998). Although several species of nematodes are recognized as important pests of individual crops, the root-knot nematodes (Meloidogyne spp.) (Perry et al., 2009) are widely considered to be the most important nematode pests because of their extensive host range, with one or more species able to parasitize nearly every crop grown in the United States. The soybean cyst nematode (Heterodera glycines) is also a major pest because of its aggressive behavior and widespread distribution in soybean production areas of the South and the Midwest. Recently the reniform nematode (Rotylenchulus reniformis) has received increasing recognition and attention as a pest of several crops, especially cotton (Koenning et al., 2004).
The importance of plant-parasitic nematodes and the losses they cause is substantiated by the level of financial support given by stakeholders to the research efforts of many of the scientists that will be involved in this project. These stakeholders include Cotton Incorporated, the National Soybean Board, the National Peanut Board, and state level growers associations for each of these major crops. Much of the support for the proposed research will come directly from these stakeholder groups. Historically, nematodes were controlled (when growers were cognizant of their effects on crop yields) by the application of either a fumigant nematicide or one of a few granular insecticides that also had nematicidal activity. All of these products have been shown to present significant hazards to both environmental and human health. The recognition of these risks have resulted in either a loss of registration or greatly increased restrictions on the use of these pesticides. No new nematicide or nematicidal chemistry that has proven effectiveness has been developed in nearly 30 years to fill this void. A few new products have been developed, such as the use of abamections as a seed treatment but with limited success (Faske & Starr, 2007). Crop rotation has the potential to suppress nematode populations but is not widely practiced by growers. The wide host range of several important nematode pests limits the number of crops that a grower can use in rotation with their primarly crop of interest to suppress nematode populations because effective rotation crops are frequently of lesser economic value (ie, velvetbean for management of root-knot nematodes on soybean; Weaver et al., 1998). Because of the intensive crop production systems utilized by many growers, if a rotation crop is to be adopted it must have substantial benefit beyond just suppression of nematode pests.
Biological control has received much attention as an ecologically sound approach to the management of nematode pests. The limited mobility of nematodes and the relative stability of the soil environment suggest that biological control has significant potential. Unfortunately, this potential has been difficult to achieve. It has proved difficult to stimulate activity of fungal species that parasitize nematodes or to successfully introduce sufficient numbers of propagules of these agents into a large area to effect suppression of the desired nematode population. The bacteria Pasteuria spp. are obligate parasites of several nematode species and have effectively suppressed nematode populations in several tests (Dickson, 2004). However, large scale application is hampered by the inability to culture the bacteria in quantities needed for commercial purposes.
Several scientists from across the Southern and Midwestern United States are currently researching the use of host resistance as a corner stone for the sustainable management of plant parasitic nematodes. Several notable successes have been achieved as a result of the collaborative efforts of scientists participating in the S-1015 multistate project. These include introgression of resistance to M. arenaria and M. javanica into peanut cultivars adapted to both the southeast (Georgia) and western (Texas) production regions. These new peanut cultivars also have the highly desirable and new traits of improved resistance to other pathogens and a high ratio of oleic to linoleic fatty acids. Finally, scientists in Georgia have utilized the initial efforts from scientists in Texas to map the nematode resistance gene in peanut (research completed in Texas) to develop a high through put marker-assisted selection system. Similarly, groups of scientists from Alabama, Georgia, Mississippi and Texas have collaborated on efforts to identify, characterize, and develop sources of resistance to both the reniform and root-knot nematodes in cotton. Genetic relationships among several genes for resistance to the root-knot nematode are being characterized and several genes are being mapped. In other studies, new sources of resistance to root-knot nematodes are being identified. Similarly, several scientists have collaborated to screen different collections of Gossypium germplasm for resistance to reniform nematodes, have identified several sources of resistance, and are working to introgress these different sources of resistance into upland cotton. Because plant breeding efforts such as those being pursued by current project members are, by their very nature, long term projects it is important that our project be renewed to enable us to build on our past successes. The multistate effort has been successful because it has allowed scientists to share resources, especially germplasm, and avoided duplication of efforts.
Additionally, we seek to expand our efforts to include a renewed emphasis on the identification of nematode species, with special emphasis on Meloidogyne spp. As little as 25 yr ago there were approximately 30 recognized Meloidogyne spp. All but four species were considered to be of minor importance in the southern United States. It has long been accepted that M. arenaria, M. javanica, and especially M. incognita were the most wide spread and economically important root-knot nematodes. Meloidogne hapla was also important in the cooler climates, at higher elevations, and in the northern edge of the region. At present, however, there are nearly 100 valid species, many of which are becoming recognized as widely distributed in the southern United States (Hunt and Handoo, 2009). Some of these species are virulent on crops with resistance to the four species which have heretofore received the most attention from researchers. Because host resistance is typically a species, and sometimes a race-specific trait, it is increasing important that we continue to develop diagnostic tools for rapid identification of many of the newly described species known to exist in our region and considered to be of economic importance. Future successes in the development and deployment of resistant crop cultivars will be dependent on knowledge of the distribution of many more Meloidogyne spp. than were previously recognized.
The proposed research addresses important agricultural crop management issues as identified by the REE Advisory Board. In the Report on Agriculture Research Needs of Commodity Crops , the REE Advisory Board states that sustainable, efficient production of these staples (including cotton, soybean, and peanut) is critical to the US food supply and economy. They further list as top priorities, nematode resistance in cotton and improved varieties and disease resistance in peanut. (http://www.ree.usda.gov/nareeeab/reports/070609commoditycrops_rpt.pdf).
If this research is not conducted, growers will continue to see a shrinking profit margin due to greater production costs and yield losses due to nematodes. The deployment of nematode-resistant crops will lower production costs by reducing the need for nematicide applications and less profitable crop rotations while at the same time improving yield by reducing losses due to nematodes. Additionally, the reduction in use of nematicides such as the carbamate aldicarb and the fumigant 1,3-dichloropropene with reduce the potential for contamination of the environment and adverse effects to public health. An economic analysis of the implications of nematode-resistant crops will be evaluated through budgeting analysis. Crop enterprise budgets will be developed for traditional crop production and for nematode-resistant crops, comparing the gross returns and net returns (returns to land).
Related, Current and Previous Work
There are currently four other multistate research projects that focus on plant parasitic nematodes, namely NE-171, NE-1019, NE-1040, NC-1035, and W-2186. All of these multistate projects are aimed at improved management of plant-parasistic nematodes but differ in specific objectives. This proposed research effort will have some overlap with the other projects but is unique in many aspects. The crops of primary interest for the proposed research include cotton, peanut, and soybean. Of these crops, cotton is of some importance to the W-2186 project and soybean to the NC-1035, with no overlap for any of these major crops in the NE projects, especially NE-1019 which focuses on horticultural crops. Additionally, our primary focus (objectives 1 and 2) is on host plant resistance, which is of less importance to the other projects except for resistance to the soybean cyst nematode in the NC-1035 project. Our Objective 3 (improved species identification) has some overlap with the W-2186 project but is necessitated by a larger array of Meloidogyne species of agriculture importance in the southern region of the US relative to the western states. Several Meloidogyne species of increasing importance in the southern region are not currently known to exist in the US outside of this region. These include M. floridensis, M. enterolobii (syn. M. mayaguensis), and M. marylandi. Additionally, M. partityla is limited to New Mexico in the western region but is widely distributed in the southern states.
Genetic resistance to nematodes and other plant pests and pathogens is generally acknowledged as the preferred method of pest management (Cook and Starr, 2006). However, because resistance is nematode species- (and frequently race-) specific, a single source of resistance will not control all nematode species. Thus resistance often must be integrated into a management system that incorporates other control tactics, such as crop rotation and the occasional use of nematicides. Although limited in scope at present, biological control of root-knot species especially with the obligate parasite Pasteuria penetrans holds much promise for being another option in such a management system (Chen and Dickson, 2004).
Soybean cultivars with resistance to H. glycines, M. incognita, and (or) R. reniformis, and resistance to M. incognita in tobacco have been available since the mid-1970's and the use of such resistance in various management systems that also use crop rotation and some application of nematicides is a well established practice. Rotation to various non-host crops or to a susceptible soybean cultivar is recommended to avoid or delay shifts in nematode virulence that can occur with frequent deployment of a single resistance gene (Riggs and Wrather, 1992). In some cases, such as with tobacco, nematicides are used with resistance to provide additional protection to the crop and to suppress other Meloidogyne spp. that may be present. Continuous use of resistance to M. incognita in tobacco has resulted in an increased incidence of damage cause by M. arenaria, for which the resistance is ineffective (Fortnum et al., 1984).
Unfortunately, there are few data that can be used to design optimal deployment strategies for recently released cultivars of peanut or for cotton cultivars currently being developed. No data are yet available on the potential of M. arenaria or M. javanica populations to become virulent on resistant peanut cultivars. It is known that other Meloidogyne spp. (M. hapla and M. haplanaria) are present in parts of the peanut production and are parasitic on the peanut cultivars with resistance to M. arenaria and M. javanica. Additionally, new Meloidogyne species are being described which may be parasitic on one or more of the resistant cultivars. Currently there are over 90 recognized Meloidogyne spp., several of which have been in found in the southern and southwestern regions of the United States (Hunt and Handoo, 2009).
Resistance to M. incognita and R. reniformis in the known cotton germplasm resources varies from moderate to near immunity, but few cultivars have been developed from these sources of resistance (Starr et al., 2007). As additional cultivars are developed and used by growers it will be important to use the resistance in such as manner so as to prolong the durability of resistance. Available data suggest that virulence to some sources of resistance being used to develop M. incognita-resistant cotton exists in some nematode populations (Zhou et al., 2000). No data are yet available on the potential for virulent races within R. reniformis populations. Because resistance suppresses nematode reproduction, even moderate levels of resistance can be useful in managing nematode population densities (Davis and May, 2003). Although the first consideration with the use of resistance is to improve yields in nematode-infested fields, the suppression of resident nematode populations with the use of resistance is a significant benefit also. Ogallo et al. (1999) demonstrated the benefit to yield of lima beans from growing resistant cotton in nematode-infested fields before planting the susceptible lima beans.
Virulence against the Mi resistance gene in tomato is known to exist in some M. incognita populations and has compromised the use of this resistance in some fields in California (Kaloshian et al., 1996). One report suggests that virulence can be detected after only five seasons of use of cultivars containing the Mi gene (Noling, 2000). The Mi source of resistance has not been widely used in the southeastern United States because growers relied primarily on the use of methyl bromide for control of nematodes and other soilborne pests. With increased restrictions on the use of methyl bromide and its eventual banning based on the Montreal Protocols, growers in the southeast will place greater reliance on resistant tomato cultivars as part of the crop management system. Because of the temperature sensitivity of Mi-mediated resistance, care will have to be exercised in the use of such resistance to obtain optimal benefits. It may be necessary to link use of resistance with other management systems to effectively manage of root-knot nematodes on tomato.
Reliance on a single gene for resistance can lead to selection of virulent nematode populations (Roberts et al. 1998). Additionally, many crops are attacked by multiple nematode parasites, which can coexist in the same field site (Koenning et al. 1999). Resistance genes typically confer resistance to only one or two species of plant-parasitic nematodes. In fields with mixed populations of economically important nematodes, frequent planting of a cultivar with resistance to only one species will lead to a population increase of nematodes not affected by resistance. Such a situation occurred in Britain where frequent planting of potato possessing the H1 gene for resistance to one potato cyst nematode, Globodera rhostochiensis, provided a selective advantage to another species, G. pallida, which was not affected by H1 (Cook and Evans, 1987). This resulted in an increase in damage to potato caused by G. pallida.
The most significant development in resistance to M. incognita in cotton was the release of Auburn 623 RNR germplasm (Shepherd 1974b). Auburn 623 RNR had far more nematode resistance than either of its parents (Shepherd 1974a) and no upland cotton germplasm with higher levels of resistance has been developed since (Robinson et al., 2001). Possible additional sources of M. incognita resistance have been identified in primitive race stocks of Gossypium hirsutum (Shepherd, 1983; Robinson and Percil, 1997; Robinson et al., 2001), but no cultivars or improved germplasm have been developed from these sources. At least some of the previously un-characterized sources of resistance to M. incognita appear to represent unique genes for resistance and different from those present in genotypes derived from Auburn 623 RNR (Starr et al.,2009). The recent development of DNA markers linked to major genes for resistance to root-knot nematodes in cotton will be a useful tool to identify new sources of resistance (Shen et al. 2006, Wang et al., 2006). For example, the lack of the marker in resistant lines may indicate the presence of a different resistance gene.
Currently, no cotton cultivar with resistance to R. reniformis is available and only low to moderate levels of resistance have been identified in any G. hirsutum genotype (Starr et al., 2007). Several members of this proposed projected are working to introgress resistance from other Gossypium species into G. hirsutum, using resistant accessions from the tetraploid G. barbadense and G. longicalyx (Robinson et al., 2007). Two introgression germplasm lines with resistance derived from the diploid G. longicalyx have been released as breeding lines (Bell et al., 2009), but these genotypes are not yet suitable from release as cultivars.
There are currently two peanut cultivars (NemaTAM and Tifguard) with resistance to M. arenaria, the dominant root-knot nematode parasitizing peanut in the Southeast and Texas. However, resistance in both cultivars is conferred by the same gene (Rma). The durability of this resistance will be compromised if virulent pathotypes of M. arenaria develop. Another concern regarding the durability of resistance is that it is only effective against M. arenaria and M. javanica, but not M. hapla (Timper et al., 2003). Although M. arenaria is the dominant species parasitizing peanut in the Southeast and Texas, populations of M. hapla have been found in all the peanut-producing states except Florida and it is the dominant species in Virginia and the Carolinas (Dickson, 1998). Planting cultivars with resistance only to M. arenaria would provide a competitive advantage to M. hapla in fields where they coexist. Potential new genes for resistance to M. hapla have been identified in Chinese accessions of domesticated peanut (Arachis hypogaea) and some interspecific hybrids with A. hypogaea (Dong et al., 2008). Moreover, some interspecific hybrids with resistance to M. arenaria do not contain the marker 197/909 for the Rma gene indicating that they have other resistance genes.
The soybean cyst nematode, H. glycines (SCN), causes the greatest yield loss in soybean of any single pathogen (Wrather and Koenning 2009). Resistant soybean varieties derived from PI88788 and Peking were very effective in managing SCN. Unfortunately, the genetic variability of this nematode results in race shifts when resistant varieties are used, such that resistant varieties become susceptible. Resistance derived from Peking was effective in only 2% of North Carolina fields from 1994-1996 (Koenning and Barker, 1998). An additional problem associated with the use of resistant varieties is that the race of SCN must be known in most instances in order to select a resistant variety. The varieties Hartwig and Delsoy 5710 derived from PI 437654 are virtually immune to all races of SCN and thus avoid the necessity of using a race determination, but have limited yield potential. Two varieties derived from Hartwig, Fowler and Anand, have high yield potential and are resistant to most races; however, the use of these varieties is limited because they lack resistance to Roundup herbicide, although efforts are under way to incorporate herbicide resistance into these varieties. The southern root-knot nematode (M. incognita) and the reniform nematode (Rotylenchulus reniformis) complicate effective deployment of resistance because these nematodes are also important pathogens of soybean, particularly in the southern region. Cultivars with resistance to SCN are often not resistant to root-knot or reniform nematodes.
RNA interference (RNAi) of parasitism genes in root-knot and cyst nematodes has tremendous potential as a new tool for engineering crop plants with resistance to these important plant parasites. Several studies have demonstrated the expression of double stranded RNA (dsRNA) in planta, uptake by either Meloidogyne spp. or Heterodera spp., and subsequent down-regulation of parasitism genes leading to a reduction in nematode infection of roots (Huang et al. 2006, Steeves et al. 2006, Yadav et al. 2006, Sindhu et al. 2009).
Root-knot nematodes. The success of resistant cultivars for the management of root-knot nematodes depends on the knowledge of the species, race, and virulence characteristics of the locally occurring population. Identification of species of Meloidogyne is normally difficult due to the relatively small inter-specific morphological variation within the genus (Jepson, 1987) and the relatively high intra-specific variation in the most widely distributed species.
A number of diagnostic techniques can be used to supplement or verify traditional identification by morphology, including isozyme patterns, DNA-based methods, and host differential tests. Several DNA-based diagnostic methods have been developed in the last few years for the most common species (Powers, 2004; Adam et al. 2007), but no single marker that can be used for all the species in the genus has been identified yet. At the intra-specific level, the use of nuclear and mitochondrial markers has produced valuable information about the phylogenetic relationships and the biogeography of populations, but much is still unknown about virulence variants.
For several decades, the North Carolina differential host test (Hartman and Sasser, 1995), which is based on the ability of a species or race to reproduce on a series of selected hosts, has been used to separate the four main Meloidogyne species, M. arenaria, M. incognita, M. hapla, and M. javanica, as well as host races of M. incognita and M. arenaria. The difficulties in characterizing Meloidogyne populations by this method have been highlighted in several publications (Carneiro et al., 2003; Fargette, 1987; Robertson et al., 2009; Stanton and O'Donnell, 1998) in which numerous atypical populations have been described. Moens et al. (2009) have suggested that since the North Carolina differential host test is not relevant to most of the more than 90 recognized Meloidogyne species, that it no longer be used to characterize root-knot populations. A revised and updated method to characterize host races or relevant physiological intra-specific variants is needed.
Numerous taxonomic studies comparing groups of species in the genus Meloidogyne have been published over the last 150 years, but only two comprehensive monographs of the genus exist (Jepson, 1987; Whitehead, 1968). The first, by Whitehead (1968), included 23 species, while the second, by Jepson (1987), included 51 species. Considering that, at present, there are nearly 100 described species (Hunt and Handoo, 2009) an updated detailed monograph of the genus is needed.
Reniform nematode. In recent years, research efforts to develop commercial cultivars with resistance to reniform nematode (Rotylenchulus reniformis) have increased. However, information on the variability among and within populations of this nematode present in the United Statesdoes does not exist. Genetic variability of reniform nematode could impact the effectiveness and longevity of management strategies based on host plant resistance, as has been the case with several cyst and root-knot nematode species in various crops (Anwar et al., 2000; Kaplan et al., 1999; Niblack et al., 2002; Noe, 1992; Riggs et al., 1981; Van der Beek et al., 1999; Zhou et al., 2000). Additionally, the potential existence of infra-specific variants with differential reproduction abilities should be investigated in order to verify the reliability of a single isolate or population for resistance-screening purposes.
Several aspects of the biology and ecology of R. reniformis have been identified as contributing to its establishment as a major pathogen of several crops in the U.S. and the world (Gaur and Perry, 1991; Robinson et al., 1997). This parasite has a broad host range, including dicotyledonous and monocotyledonous plants, making control by crop rotation difficult. It also has the ability to enter an anhydrobiotic state to remain viable for long periods of time in dry soil and to facilitate survival in the absence of a host. Additionally, it possesses a high reproductive rate, a short life cycle, and the ability to colonize the plow layer from depths below the effective range of nematicide treatment. Robinson (2007) discusses why these and other biological attributes explain how this species is expected to colonize new fields or certain fields faster than other nematodes would, but do not fully explain the temporal changes in distribution observed over broad geographical regions.
Research on the population biology and ecology of reniform nematode in the United States is lacking. Only one published study addresses the question of intraspecific variability of R. reniformis in the United States (Agudelo et al., 2005). Multivariate analysis of variance and discriminant analysis were used to determine the variability of morphology in males and immature females of populations from cotton-growing locations. Additionally, reproduction indices of populations were measured on selected soybean and cotton genotypes in the greenhouse. High variability in morphometrics and reproduction was observed within all the populations, and several differences were found among populations. DNA sequences of the nuclear ribosomal first internal transcribed spacer region (ITS1) were compared among United States populations and to sequences of populations from Brazil, Colombia, Honduras and Japan. No polymorphic nucleotide sites were observed among the amphimictic populations, with this marker. Only a parthenogenic population from Japan was distinct. The conclusions of this work were that phenotypic polymorphisms of the species in the United States could impact the effectiveness of management strategies based on host plant resistance, and that further phylogenetic analyses including other markers informative at the population level (e.g. microsatellites) needed to be undertaken.
Several authors have reported morphometric variability among populations of reniform nematode and among individuals of a single population (Dasgupta et al., 1968; Germani, 1978; Lehman and Inserra, 1989; Linford and Oliveira, 1940; Nakasono, 1983; Robbins, 1994; Sivakumar and Seshadri, 1971; Soares et al., 2003; Van der Berg, 1978). Dasgupta et al. (1968) examined specimens from diverse geographic origins and characterized the species as "polymorphic", reporting the occurrence of populations without males, and of individuals ranging from small to large in size. In Japan, Nakasono (1983) identified three morphologically distinguishable groups based on size (small, medium and large), and three biological types based on frequency of male occurrence (male-numerous, male-rare and male-absent). This naturally occurring intraspecific variability has not been correlated to host species, geographic origin, population density, or any environmental factor. Neither has any phenotypic variation been correlated to molecular markers.
Genetic diversity refers to the variation at the individual genomic level and supports evolution and adaptation of nematodes to their changing environment. In agroecosystems, the pattern and amount of population genetic diversity in obligate plant-parasitic nematodes is mainly shaped by plant host factors. The assessment of genetic variation and related features of populations is very relevant to the design of sustainable management strategies for reniform nematode on cotton.
Literature on phenotypic variation in reniform nematode related to host preferences is scarce, and no standardized tests exist to identify variants within this species. Dasgupta and Seshadri (1971) proposed the existence of two races in India, based on a study of reproduction on cowpea, castor and cotton, where 9 of 10 morphologically similar populations of reniform nematode were able to reproduce on the three hosts, and one would reproduce only on cowpea. Vadhera et al. (1999) subsequently confirmed this observation. Nakasono (1983) used a system analogous to the race determination scheme for soybean cyst nematode (Golden et al., 1970) to characterize differences among populations of reniform nematode in Japan, by comparing reproduction on nine plant species. His results suggested variations in host preferences within populations, and implied that the genetic structure of the populations could be altered over successive generations. McGawley and Overstreet (1995), in a study including reniform nematode populations from Arkansas, Hawaii, Louisiana, Mississippi, and Texas, reported variations among populations, in reproduction and damage to cotton and soybean. No genetic markers correlated to differential responses on plant hosts, or to any other type of infraspecific variation in R. reniformis, have been reported.
Under the previous project much progress was made with respect to developing resistance to root-knot nematodes M. arenaria and M. javanica in peanut (Holbrook et al., 2008; Simpson et al., 2003), including the development of maker assisted selection protocols (Chu et al., 2007; Church et al., 2000). Nematode resistance has even been combined with the highly desirable traits of resistance to tomato spotted wilt virus and a high ratio of oleic to linoleic fatty acids. In the new project peanut emphasis will be placed on the developed of peanuts with all three of these highly desirable traits along with inproved resistance to other diseases, especially early and late leafspots and Sclerotina blight. Additionally, renewed effort will be placed on the development of resistance to M. hapla (Dong et al., 2008), which is expected to become a more important pathogen with the wide spread deployment of resistance to the current sources of resistance. Research is also needed on how to most effectively deploy the newly developed resistance to reduce the probability of development of virulent nematode populations that can overcome this resistance.
In cotton, several sources of resistance to the reniform nematode have been identified introgressed into G. hirsutum (Bell et al., 2009; Starr, unpublished data). However, all of these genotypes lack the agronomically important traits of high yield and high fiber quality. Additionally, high through-put marker-assisted selection protocols need to be developed before private sector seed and breeding companies will begin using this germplasm to produce high quality crop cultivars. Additionally, because current available resistance M. incognita in cotton is narrowly based and evidence of virulence in nematode populations has been reported (Zhou et al., 2000), it is important that other cotton accessions reported to be resistant to root-k not nematodes be investigated for allelic relationships with the mostly widely used sources of resistance(Starr et al., 2009). As with nematode resistance in peanut, the has been little research on how best to integrated nematode resistance in cotton into various cropping systems to maximize its potential for increasing sustainable crop production with fewer pesticide inputs.
Economic analytical procedures used for Integrated Pest Management (IPM) will be the basis for this project. Budgeting analysis will be used for economic evaluation of nematode resistance. Budgeting analysis has a rich history ranging from evaluation of new varieties (comparing the new to the traditional), to comparison of technologies. For example, releases of cotton cultivars were compared to gain insight on the implications to returns to land (profit) as presented in Masud and Lacewell(1990)and Masud et al. (1981, 1985).
Objectives
-
Integrate resistant cultivars with other nematode management tactics to reduce selection pressure on nematode populations and communities.
-
Identify and incorporate new sources of resistance into elite germplams lines and cultivars ) and evaluate economic implications.
-
Facilitate and improve identification of nematode species and races .
Methods
Obj. 1 Integrate resistant cultivars with other nematode management tactics to reduce selection pressure on nematode populations and communities. Soybean. One means to prevent or delay race shifts is the use of blends of resistant and susceptible varieties (Anand et. al., 1995; Tinius et. al., 1998). Blends of varieties may yield as well or better than the parent lines in some instances and may provide more durable disease resistance. The proposed research will evaluate the use of blends of soybean varieties resistant and susceptible to soybean cyst nematode for yield, their ability to minimize reproduction of this nematode, prevent race shifts of SCN, and provide additional tactics for management of this important soybean pathogen. These objectives will be accomplished through a three-year (possibly longer) study evaluating various blends of resistant and susceptible soybean cultivars. Cotton. Resistant cultivars often have lower yield potential than susceptible cultivars in fields not infested with damaging nematode population densities (Starr and Mercer, 2009). A recent study showed that, compared to continuous nematode-susceptible cotton, rotating resistant and susceptible genotypes provides a yield benefit to the susceptible cotton even when nematicides are used (Davis, unpubl.). Moreover, rotating resistant and susceptible cotton also will reduce selection for virulent nematode pathotypes thereby prolonging the effectiveness of the resistance genes. A collaborative study with an agricultural economist will be conducted to determine whether rotating high-yielding resistant and susceptible cotton increases net economic return compared to either continuous susceptible or resistant cotton. Because nematode penetration into the roots of resistant cotton may cause enough damage to the roots to reduce yield even if very few of the nematodes can complete their life cycle, another study will be conducted to determine whether nematode penetration into roots of resistant cotton causes measurable yield loss. If yield loss occurs with moderate or high initial nematode population densities, then it may be necessary in some instances to combine use of a resistant cultivar with nematicide application, with crop rotation, or another control tactic to obtain maximum benefit from genetic resistance. Peanut. As with soybean and cotton, there is concern that continuous use of newly developed root-knot resistant cultivars will compromise that resistance through the development of virulence populations. Thus several experiments will be initiated to determine the impact of occasional use of a nematode resistant cultivar on nematode population densities. These studies will compare rotating resistant and susceptible peanut cultivars along with other crops in a long term cropping systems. Because only M. incognita parasitizes cotton whereas M. arenaria and M. javanica are the most common species parasitizing peanut, a cotton peanut rotation is helpful in suppressing root-knot nematode population densities on both crops. It will be useful to determine if the occasional use of resistance in either or both hosts will provide further benefits. Additionally, as cropping systems shift based on the economic climate such as a recent increase in corn acreage with a corresponding decline in cotton, and because many corn hybrids are susceptible to all of these Meloidogyne spp. (Windham, 1998), it will be important to determine how the resistant peanut cultivars fit in a cropping system with corn or other grain crops. Obj. 2 Identify and incorporate new sources of resistance into elite cultivars. Cotton. Most of the resistance to M. incognita currently used in cotton is based on one of three related sources (Starr et al., 2007); resistance derived from the old cultivar Clevewilt-6, resistance derived from Auburn 623 RNR (which was derived in part from Clevewilt-6) and the recently developed Acala NemX, which may have the same major genes for resistance as Clevewilt-6 (Zhou et al., 1999). A previously identified DNA marker (CIR316) (Wang and Roberts, 2006) linked to a major gene for resistance to root-knot nematodes will be used as a tool to identify new sources of resistance in 12 primitive race stocks of Gossypium hirsutum. A germplasm line previously proposed as a new source of root-knot resistance has been confirmed to have a different resistance gene. QTLs for nematode resistance genes in this germplasm are being identified through bulk segregate analysis. Additionally, five primitive G. hirsutum accessions identified as resistant to M. incognita by Robinson and Percival (1997) have recently been shown to have genes for resistance that are different from those in Clevewilt-6 or Wild Mexico Jack Jones (the second source of resistance in the Auburn 623 RNR and its derivatives)(Starr, unpublished data). Several cotton breeding programs are beginning to use these newly identified sources of resistance. Recently, near immunity to the reniform nematode has been introgressed from G. longicalyx into G. hirsutum (Robinson et al., 2007). Unfortunately, this newly developed germplasm is not suitable for cultivar development and additional breeding efforts are needed to break linkages between reniform-resistance and undesirable agronomic traits. This will likely require several backcross generations and the screening of a large number of progeny in each generation to achieve the objective of a high level of resistance and high yield potential. Other more moderate sources of resistance to R. reniformis were identified in the previous multi-state project (Robinson et al.,2006; Weaver et al., 2007) and breeding efforts are ongoing to develop cotton germplasm with moderate levels of resistance to this nematode. These efforts are using both backcross and pedigree breeding strategies to achieve their objective. Peanut. Genes for resistance to M. hapla and additional resistance genes for M. arenaria will be identified and characterized so we can begin incorporating them into lines with good agronomic characteristics. The genetics of resistance to M. hapla in Chinese accessions of A. hypogaea will be determined by crossing resistant lines with two different susceptible cultivars. Accessions with potential new resistance genes for M. arenaria will be crossed with a susceptible parent and Tifguard and segregation patterns for resistant progeny will be evaluated in the F2. The progeny and the parents will be assessed for the 197/909 marker linked to the Rma gene (Garcia et al., 1996) and for resistance to M. arenaria in the greenhouse. Additionally, preliminary data suggests that the interspecific Arachis hybrid TXAG-6 that was used as the primary source of resistance to M. arenaria is also resistant to M. hapla, but that the resistance to M. hapla was lost during the development of the M. arenaria resistant germplasm (Nelson et al., 1989). This potentially useful source of resistance to M. hapla will be further evaluated and, if the preliminary report is confirmed, cross between TxAg-6 and M. hapla-susceptible cultivars will be made. An effort will also be made to map the M. hapla-resistance locus and to develop high-throughput markers linked to the resistance locus. This effort will use both SSR and AFLP systems to map the resistance gene in populations segregating for the resistance phenotype. Newly identified sources of resistance to M. hapla will subsequently be screned against a number of geographically diverse populations of M. hapla to provide an estimate of the durability and stability of this resistance. Soybean. Soybean breeding lines and occasionally PIs will be tested in greenhouse pot assays for resistance to the reniform nematode. Additional work will involve greenhouse screening of transgenic soybean lines with RNAi constructs inserted to provide resistance to soybean cyst nematode. Soybean lines carrying SCN-resistance that is different from the sources (PI 88788, Peking, and PI 437654) currently used in commercial cultivars will be identified. The new resistance will be incorporated into elite SCN-susceptible soybean cultivars to develop soybean germplasms and cultivars against SCN populations that are virulent to current commercial cultivars. New molecular markers will be developed for identification of the resistance. Economic implications of resistance will be estimated through development of crop enterprise budgets, one where the crop has resistance and one without the resistance. The crop enterprise budget is comprised of expected yield, variable inputs, and fixed costs. Incorporating the price for the product as well for each of the variable inputs gives the economic components for analysis or the base information to compare gross returns, returns above fixed costs and returns to land between resistant plants and non resistant plants in a production system. Risks will be handled with sensitivity analysis. Objective 3 Identification of Meloidogyne species. Efforts will be made to discover new root-knot nematode species, new host associations, and new geographic occurrences. It is important to characterize new agriculturally important nematodes with expanded or novel morphological and molecular diagnostic characters for the development of improved diagnostic keys and phylogenetic trees. New species of root-knot nematodes from field crops, grasses, and natural areas adjacent to croplands will be described. This work will incorporate traditional morphological studies, SEM, and molecular approaches to better define species limits so that crop pests are identified more accurately (Eisenback and Hunt, 2009; Hunt and Handoo, 2009). These species will be established in culture, when possible, and experimental host ranges will be determined. Results can be used to predict the future pathogenic potential of new and/or obscure species, which may become more important as land uses change. Identification of intraspecific virulence in root-knot, reniform, and soybean cyst nematodes. The development of effective management strategies is directly related to the ecological significance of the phenotypic variation in the nematode pest and the correlation of such variation with genetic diversity in the nematode. The identification of intraspecific variation will have practical significance to control nematodes or reduce damage to crops through the use of resistant varieties and non-host plants. More precise damage thresholds and functions will be developed for reniform and root-knot nematodes on cotton and soybean. Selected reniform and root-knot nematode-resistant cotton and soybean cultivars will be employed in microplot and field environments to evaluate their resistance more precisely. These plants will be exposed to a range of each of the nematodes, and yield and nematode disease reactions will be determined. Additional cotton germplasm and breeding lines will be screened under greenhouse conditions to evaluate their suitability as hosts of the reniform nematode. To determine variability of reniform nematodes, populations will be increased in the greenhouse and will be used to assess host range, reproduction, and pathogenicity. Host range studies involve inoculation of host differentials used to separate species and races of root-knot nematode as well as several other leguminous and non-leguminous plant species. Selected populations will be advanced to a sheltered microplot environment for further evaluation. At harvest, plant dry weights, cotton yield, and nematode reproduction will be determined. Greenhouse studies will be conducted to determine seasonal changes of soybean cyst nematode (SCN) dormancy and infectivity, in order to optimize the procedures of SCN virulence phenotype tests. SCN virulence phenotypes (HG Types) are determined by greenhouse bioassays. The results of these assays are affected by numerous factors, including the nematodes (source, stage, viability, diapause, inoculation level, etc.), the soybean plants, and the environment (temperature, soil texture, moisture, etc.). In more northern regions of soybean production (i.e., Minnesota) SCN appears to be highly dormant and its infectivity is very low during the winter. This study will improve the efficiency and accuracy of analysis of SCN virulence phenotypes. There is a big need for testing for virulence phenotypes of SCN populations in commercial fields. This study will increase the capacity to analyze SCN virulence phenotypes for commercial samples. With our commercial service, growers can select appropriate SCN-resistant cultivars for effective SCN management. Another activity under this objective will be to characterize the genetic diversity of populations of R. reniformis associated with cotton in the United States, and to study the selection that host plants exert on R. reniformis populations. We propose to use microsatellites to characterize the genetic variability present among and within the collected populations. Microsatellite loci will be isolated by enriched library screening. Populations will be scored for the microsatellite loci generated, and the genetic diversity for each population estimated by standard genetic parameters: average allelic richness across loci standardized to the smallest sample size, observed heterozygosity and expected heterozygosity. We will use amplified fragment length polymorphisms (AFLP) to assess the host-induced selection on populations of reniform nematode. We will measure the effect of two rotation crops recommended for managing reniform nematode on cotton: corn and resistant soybean. Crop rotation studies generally indicate that population densities of reniform nematode rebound fully during the first year back into cotton (Davis et al., 2003). No population genetic studies have been published for this nematode, so it is unclear if any selection occurs during these rotations. Rotation combinations of cotton-corn-soybean will be simulated in the greenhouse, allowing three months for each host. Part of this activity will be to screen numerous populations of R. reniformis for variation in virulence against sources of resistance currently being developed. Ten reniform nematode populations will be collected from infested fields from numerous locations using a line-transect method and used to inoculate a standardized set of differential hosts with resistance derived from G. longicaylx and G. barbadense, along with a standard susceptible cultivar. Such data will allow estimation of variability on both a fine (within a field) and coarse (across states) scale of distribution. Preliminary data suggests that measuring variation in virulence based on eggs/g roots will be more reliable than data based on vermiform stages extracted from infested soil for short time greenhouse assays. Survival of inoculums in short term tests can confound interpretation of counts made after a 6-8 wk incubation period. In the long term, populations that are found to vary in virulence on a specific source of resistance can for studies on inheritance of virulence. Since R. reniformis reproduces by cross-fertilization it is amenable to standard genetic analyzes of the virulence phenotype. A key component of the project will be the use of common protocols, rapid exchange of data and germplasm, and coordination of effort to reduce unnecessary redundancy while ensuring key discoveries are vigorously tested in multiple location. Each project participant will select one or more key areas of activity (eg. Under Obj. 1 P. Agudelo will emphasis identification of species and race specific primers for nematode identification whereas J. Starr will focus on development of standard protocols and differential host genotypes for characterization of virulence in reniform populations). Although several participants will be working on resistance to root-knot and reniform nematodes in cotton (Obj. 2), each will be working with unique germplasm resources to ensure broad coverage of available germplasm. New sources of resistance, either directly from germplasm collections or following introgression into advanced generation breeding lines with improved agronomic traits will be rapidly shared with other project participants for additional testing. This will allow for evaluation of gene x environmental interactions and determination of how broadly the resistance can be deployed. During the first year of the project a coordinator will be selected for each of three objectives to facilitate the reporting of project accomplishments.Measurement of Progress and Results
Outputs
- Development of crop breeding lines and cultivars with improved, novel sources of resistance to plant parasitic nematodes.
- Development of new cropping systems based on cultivars with resistance to plant parasitic nematodes with economic implications as reflected in crop enterprise budgets.
- Descriptions of new species and virulence races, especially of Meloidogyne spp, and R. reniformis.
- Development of a data base on the distribution of newly described nematode species and virulence races.
Outcomes or Projected Impacts
- Identification novel sources of resistance and introgression into suitable crop genotypes will result increased durability of the resistance phenotype.
- Release of new sources of genetic resistance will enable private sector seed companies to develop new resistant cultivars.
- Increased availability of crop cultivars with resistance to plant parasitic nematodes will decreased crop yield losses and reduce the reliance on pesticides.
Milestones
(2010): Development and adoption of standardized protocols for screening populations of Meloidogyne spp. and Rotylenchulus reniformis for virulence on different sources of host resistance. Initiate field testing of yield potential and level of nematode resistance of breeding lines and cultivars currently being developed. Initiate field tests of the efficacy of host resistance in different cropping systems.(2011): Complete region-wide testing of molecular methods for identification of Meloidogyne species and development of a plan for testing large numbers of field populations. Complete ongoing efforts at screening cotton and peanut germplasm collections for new sources of resistance to Meliodogyne incognita and Rotylenchulus reniformis.
(2012): Complete the identification of molecular markers linked to newly identified sources of resistance in cotton and peanut. Develop and initiate breeding strategies for introgression of newly identified sources of resistance into agronomically superior crop genotypes.
(2013): Increase seed inventories of various breeding lines that were identified in the previous project in preparation for release of germplasm to private sector seed companies. Make advance generation selections and crosses among more recently identified sources of resistance. Complete screening of Meloigdogyne populations for presence of new species. Complete screening of Rotylenchulus reniformis populations for virulence to multiple sources of resistance in cotton.
(2014): Complete data analysis and publication of results of research in refereed journals. Dissemination of results to the public as outlined in the Outreach plan.
Projected Participation
View Appendix E: ParticipationOutreach Plan
More basic aspects of this research (i.e., resistance/parasitism gene discovery and characterization) will be published in refereed journals such as the Journal of Nematology, Phytopathology, and Molecular Plant-Microbe Interactions. Initial reports on efficacy of various cropping systems based on host resistance and biological control will also be published in more applied, peer reviewed journals such Plant Disease, Peanut Science, and the Journal of Cotton Science. Such publications are typically preceded by presentation of preliminary data at meetings of various learned societies (American Phytopathological Society, Society of Nematologists) and at commodity group meetings (American Peanut Research & Education Society, Beltwide Cotton Conferences, Southern Soybean Disease Workers). Additionally, project personnel will make regular reports on research findings to commodity groups within each state (i.e., North Carolina State Support Program - Cotton Incorporated, South Carolina Soybean Board, Texas Peanut Producers Board). Crop germplasm lines, advanced generation breeding lines and cultivars developed by project personnel will be released in accordance with policies of cooperating institutions and the various state foundation seed organizations. Cropping systems developed based on the results of this research will be demonstrated via linkage with cooperative extension personnel in each state and made available to the public via traditional print media and electronic venues. It will be especially important to establish a website for rapid dissemination project accomplishments and to have links to the Society of Nematologists website (www.nematologists.org) and other relevant websites such as the soybean cyst nematode home page (http://nematode.unl.edu/scn.htm), and the nematode control recommendations for vegetables (www.aces.edu/pubs/docs/A/ANR-0030/), regional IPM websites and state ag extension websites (www.ces.ncsu.edu/depts/pp/notes/Soybean/soy001/soy001.htm) that provide plant disease control recommendations.
Organization/Governance
Activities of the project will be coordinated by elected officers serving as Chair, Vice-Chair, and Secretary. Each officer will serve a two year term, with the Vice-Chair automatically moving up to the position of Chair after two years. Additionally, an Objective Coordinator will be appointed for each of the three principle objectives of the project, they will be responsible for summarizing research results for the objective for which they are responsible. Administrative guidance will be provided by an assigned Administrative Advisor and a CSREES Representative. Project members will meet annually to review research progress and to plan and coordinate future efforts. Additionally, annual meetings will be used to review and revise as needed common protocols for screening plant germplasm and nematode populations for virulence. The use of such common protocols will be essential for eventual publication of these data. Many decisions on exchange of germplasm will also be made at the annual meetings.
Literature Cited
Adam, M.A.M., Phillips, M.S. and Blok, V.C. (2007) Molecular diagnostic key for identification of single juveniles of seven common and important species of Meloidogyne. Plant Pathology 56, 190-197.
Anand, S. C., Koenning, S. R., and Sharma, S. B. 1995. Performance of blends of soybean cyst nematode resistant and susceptible soybean cultivars. Crop Science 35:524-528.
Anwar, S. A., M. V. McKenry, and J. Faddoul. 2000. Reproductive variability of field populations of Meloidogyne spp. on grape rootstocks. Journal of Nematology 32:265-270.
Agudelo, P., R.T. Robbins, J.McD. Stewart, and A.L. Szalanski. 2005. Intraspecific variability of Rotylenchulus reniformis from cotton-growing regions in the United States. Journal of Nematology 37: 105-114.
Bell, A.L., Starr, J.L., Jones, J. E., Lemon, R.G., Nichols, R. L., Overstreet, C., and Stelly, D.M. 2009. Nematode resistance and agronomic performance of LONREN and NEMASTACK lines. Proc Cotton Disease Council, Belt Cotton Conferences.
Carneiro, R.M., R.G. Carneiro, D.I. Das Neves, M.A. Almeida. 2003. New race of Meloidogyne javanica on Arachis pintoi in the state of Parana. Nematol. Bras. 27:219-221.
Chu, Y., C. Holbrook, P. Timper, and P. Ozias-Akins. 2007. Development of a PCR-based molecular marker to select for nematode resistance in peanut. Crop Sci. 47:841-847.
Church, G. T., C. E. Simpson, M. D. Burow, A. H. Paterson, and J. L. Starr. 2000. Use of RFLP markers for identification of individuals homozygous for resistance to Meloidogyne arenaria in peanut. Nematology 2:575-580
Cook, R., and K. Evans. 1987. Resistance and tolerance, Pp. 179-231 in R. H. Brown and B. R. Kerry, eds. Principles and Practice of Nematode Control in Crops, New York: Academic Press.
Dasgupta, D. R., D. J. Raski, and S. A. Sher. 1968. A revision of the genus Rotylenchulus Linford and Oliveira, 1940 (Nematoda: Tylenchidae). Proceedings of the Helminthological Society of Washington 35:169-172.
Dasgupta, D. R., and A. R. Seshadri. 1971. Races of the reniform nematode, Rotylenchulus reniformis Linford and Oliveira, 1940. Indian Journal of Nematology 1:21-24.
Davis, R.F., S.R. Koenning, R.C. Kemerait, T.D. Cummings, W.D. Shurley. 2003. Rotylenchulus reniformis management in cotton with crop rotation. Journal of Nematology 35:58-64.
Davis, R. F., and O. L. May. 2003. Relationships between tolerance and resistance to Meloidogyne incognita in cotton. Journal of Nematology 35:411-416.
Dickson, D.W. 1998. Peanut, Pp. 523-566 in K. R. Barker, G. A. Pederson and G. L. Windham, eds. Plant and nematode interactions, Madison, WI: American Society of Agronomy, Crop Science Society of America, Soil Science Society of America.
Dong, W.B., C.C. Holbrook, P. Timper, T.B. Brenneman, Y. Chu, and P. Ozias-Akins. 2008. Resistance in peanut cultivars and breeding lines to three root-knot nematode species. Plant Disease 92:631-638.
Fargette, M. 1987. Use of the esterase phenotype in the taxonomy of the genus Meloidogyne. Rev. Nematol. 10;45-56.
Fortnum, B. A., Krauzs, J. P., and Conrad, N. G. 1984. Increasing incidence of Meloidogyne arenaria in flue-cured tobacco in South Carolina. Plant Disease 68:244-245.
Garcia, G. M., Stalker, H. T., Shroedeer, E., and Kochert, G. 1996. Identification of RAPD, SCAR, and RFLP markers tightly linked to nematode resistance genes introgressed from Arachis cardenasii into A. hypogaea. Genome 39:836-845.
Gaur, H.S. and R.N. Perry. 1991. The biology and control of the plant-parasitic nematode Rotylenchulus reniformis. Agricultural Zoology Reviews 4:177-212.
Germani, G. 1978. Caractères morpho-biométriques de trois espèces ouest-africaines de Rotylenchulus Linford & Oliveira 1940 (Nematoda:Tylenchida). Revue de Nématologie 1:241-250.
Golden, A. M., J. M. Epps, R. D. Riggs, L. A. Duclos, J. A. Fox, and R. L. Bernard. 1970. Terminology and identity of infraspecific forms of the soybean cyst nematode (Heterodera glycines). Plant Disease Reporter 54:544-546.
Hartman, K.M. and J.N. Sasser. 1995. Identification of Meloidogyne species on the basis of differential host test and perineal pattern morphology. Pages 69-77 in: An Advanced Treatise on Meloidogyne, Vol. II. K.R. Barker, C.C.
Carter, and J.N. Sasser, eds. North Carolina State University Graphics, Raleigh.
Holbrook Jr, C.C., Timper, P., Culbreath, A.K., Kvien, C.K. 2008. Registration of 'Tifguard' Peanut. Journal of Plant Registrations. 2:92-94.
Huang, G. Z., R. Allen, E. L. Davis, T. J. Baum, and R. S. Hussey. 2006. Engineering broad root-knot resistance in transgenic plants by RNAi silencing of a conserved and essential root-knot nematode parasitism gene. Proceedings of the National Academy of Sciences of the United States of America 103: 14302-14306.
Hunt, D. J., abnd Handoo, Z. A. 2009. Taxonomy, identification, and principal species. IN: R. N. Perry, M. Moens, and J. L. Starr (eds.). Root-knot Nematodes. Wallingford, UK: CABI Press (In Press)
Jepson, S. B. 1987. Identification of root-knot nematodes (Meloidogyne species). Wallingford, UK, C.A.B. International.
Kaplan, M., E. P. Caswell-Chen, and V. M. Williamson. 1999. Assessment of host- induced selection on three geographic isolates of Heterodera schachtii using RAPD and AFLP markers. hytopathology 89:68-73.
Kaloshian, I., Williamson, V. M., Miyao, G., Lawn, D. A., and Westerdahl, B. B. 1996. Resistance-breaking nematodes identified in California tomatoes. California Agriculture 50:18-19.
Koenning, S. R., 2002. Southern United States Soybean Disease Loss Estimate for 2001. Proceedings of the Southern Soybean Disease Workers 29th Annual Meeting.
Koenning, S. R. 2004. Resistance of soybean cultivars to field populations of Heterodera glycines in North Carolina. Plant Disease 88:942-950.
Koenning, S. R., and Barker, K. R. 1998. Survey of Heterodera glycines races and other plant-parasitic nematodes on soybean in North Carolina. J. Nematol. (suppl.) 30:569-576.
Koenning, S. R., Barker, K. R., and Bowman, D. T. 2001. Resistance as a tactic for management of Meloidogyne incognita on cotton in North Carolina. Journal of Nematology 33:126-131.
Koenning S. R., Overstreet C., Noling J. W., Donald P. A., Becker J. O. and Fortnum B. A. 1999. Survey of crop losses in response to phytoparasitic nematodes in the United States. Journal of Applied Nematology 31: 587-618.
Lehman, P. S., and R. N. Inserra. 1989. Morphometric variation of Rotylenchulus parvus and Rotylenchulus reniformis populations in the southern United States. Proceedings of the Soil and Crop Science Society of Florida 49:220-226.
Linford, M. B., and J. M. Oliveira. 1940. Rotylenchulus reniformis, nov.gen., n.sp., nematode parasite of roots. Proceedings of the Helminthological Society of Washington 7:35-42.
Masud, Sharif M. and Ronald D. Lacewell. 1990. Energy, Water and Economic Savings of Improved Production Systems: Texas High Plains, American Journal of Alternative Agriculture 2, 69-75.
Masud, Sharif M., Ronald D. Lacewell, Emery Boring III and Thomas Fuchs. 1985. Economic Implication of a Regional Uniform Planting Date Cotton Production System: Texas Rolling Plains. Journal of Economic Entomology 78, 535-41.
Masud, Sharif M., Ronald D. Lacewell, C. Robert Taylor, John H. Benedict and Lawrence Lippke. 1981. Economic Impact of Integrated Pest Management Strategies for Cotton Production in the Coastal Bend Region of Texas. Southern Journal of Agricultural Economics 13(2) December, 47-52.
McGawley, E. C., and C. Overstreet. 1995. Reproduction and pathological variation in populations of Rotylenchulus reniformis. Journal of Nematology 27:508 (Abstr.).
Moens, M., Perry, R. N., and Starr, J. L. 2009. Meloidogyne species A diverse group of novel and important plant parasites. IN: R. N. Perry, M. Moens, and J. L. Starr (eds.). Root-knot Nematodes. Wallingford, UK: CABI Press (In Press)
Nakasono, K. and M. Ichinohe. 1967. A Japanese reniform nematode, Rotylenchulus nicotiana (Yokoo and Tanaka, 1954) n. comb. (Tylenchida: Hoplolaimidae). Japanese Journal of Applied Entomology and Zoology 4:169-176.
Nakasono, K. 1983. Studies on morphological and physio-ecological variation of the reniform nematode, Rotylenchulus reniformis Linford and Oliveira, 1940 with an emphasis on differential geographical distribution of amphimictic and parthenogenetic populations in Japan. Bulletin of the National Institute of Agricultural Sciences, Japan 38:4-67.
Nelson, S. C., C. E. Simpson, and J. L. Starr. 1989. Resistance to Meloidogyne arenaria in Arachis spp. germplasm. Supplement to Journal of Nematology (Annals Applied Nematology) 21:654-660.
Niblack, T. L., P. R. Arelli, G. R. Noel, C. H. Opperman, J. H. Orf, D. P. Schmitt, J. G. Shannon, and G. L. Tylka. 2002. A revised classification scheme for genetically diverse populations of Heterodera glycines. Journal of Nematology 34:279-288.
Noling, J. W. 2000. Effects of continuous culute of a resistant tomato cultivar on Meloidogyne incognita soil population densities and pathogenicity. Journal of Nematology 32:452 (Abstr).
Ogallo, J. L., Goodell, P. B., Eckert, J. W., and Roberts, P. A. 1999. Management of root-knot nematodes with resistant cotton cultivar NemaX. Crop Science 39:418-421.
Powers, T. 2004. Nematode molecular diagnostics: from bands to barcodes. Annual Review of hytopathology, 42: 367-383.
Riggs, R. D., M. L. Hamblen, and L. Rakes. 1981. Infra-species variation in reactions to hosts in populations. Journal of Nematology 13:171-179.
Riggs, R. D., and Wrather, J. A. 1992. Biology and management of the soybean cyst nematode. St. Paul, MN: APS Press.
Robbins, R. T. 1994. Variation in the size of Rotylenchulus reniformis eggs, J2, males, and young females from resistant soybean. Journal of Nematology 26:109-110 (Abstr.).
Roberts, P. A., W. C. Matthews, and J. C. Veremis. 1998. Genetic mechanisms of host-plant resistance to nematodes, pp. 209-238, Plant and Nematode interactions. American Society of Agronomy, Madison, Wisconsin.
Robertson, L., M.A. Diez-Rojo, J.A. Lopez-Perez, A. Piedra Buena, M. Escuer, . Lopez-Cepero, C. Martinez, and A. Bello. 2009. New host races of Meloidogyne arenaria, M. incognita, and M. javanica from horticultural regions of Spain. Plant Disease 93:180-184.
Robinson, A.F. 2007. Reniform in U.S. cotton: when, where, why, and some remedies. Annual Review of Phytopathology 45:263-88.
Robinson, A. F., Akridge, J. R., Bradford, J. B., Cook, C. C., Gazaway, W. S., McGawley, E. C., Starr, J. L., and Young, L. D. 2006. Suppression of Rotylenchulus reniformis 122 cm deep endorses resistance introgression in Gossypium. Journal of Nematology 38:195-209.
Robinson, A. F., Bell, A. A, Dighe, N. D., Menz, M. A., Nichols, R. L., and Stelly, D. M. 2007. Introgression of resistance to nematode Rotylenchulus reniformis into upland cotton (Gossypium hirsutum) from G. longicalyx. Crop Science 47:1865-1877.
Robinson, A. F., D. T. Bowman, C. G. Cook, J. N. Jenkins, J. E. Jones, L. O. May, S. R. Oakley, M. J. Oliver, P. A. Roberts, M. Robinson, C. W. Smith, J. L. Starr, and J. M. Stewart. 2001. Nematode Resistance, pp. Pp. 68-72. In T. L. Kirkpatrick and C. S. Rothrock (eds.), Compendium of Cotton Diseases, second edition ed. APS Press, St. Paul, MN.
Robinson, A. F., and Percival, A. E. 1997. Resistance to Meloidogyne incognita race 3 and Rotylenchulus reniformis in wild accessions of Gossypium hirsutum and G. barbadense from Mexico. Journal of Nematology 29:746-755.
Shen, X. L., G. Van Becelaere, P. Kumar, R. F. Davis, O. L. May, and P. Chee. 2006. QTL mapping for resistance to root-knot nematodes in the M-120 RNR Upland cotton line (Gossypium hirsutum L.) of the Auburn 623 RNR source. Theoretical and Applied Genetics 113: 1539-1549.
Shepherd, R. L. 1974a. Transgressive segregation for root-knot nematode resistance in cotton. Crop Science 14:872-875.
Shepherd, R. L. 1974b. Registration of Auburn 623-RNR cotton germplasm. Crop Science 14: 911-911.
Shepherd, R. L. 1983. New sources of resistance to root-knot nematodes among primitive cottons. Crop Science 223:999-1002.
Shepherd, R. L., J. C. McCarty, J. N. Jenkins, and W. L. Parrott. 1996. Registration of nine cotton germplasm lines with resistance to root-knot nematodes. Crop Science 36:820.
Simpson, C. E., J. L. Starr, G. T. Church, M. D. Burow, and A. H. Paterson. 2003. Registration of NemaTAM peanut. Crop Science 43:1561.
Sindhu, A. S., T. R. Maier, M. G. Mitchum, R. S. Hussey, E. L. Davis, and T. J. Baum. 2009. Effective and specific in planta RNAi in cyst nematodes: expression interference of four parasitism genes reduces parasitic success. Journal of Experimental Botany 60: 315-324.
Sivakumar, C. V., and A. R. Seshadri. 1971. Life history of the reniform nematode, Rotylenchulus reniformis Linford and Oliveira, 1940. Indian Journal of Nematology 1:7- 20.
Soares, P. L. M., J. M. dos Santos, and P. S. Lehman. 2003. Estudo morfométrico comparativo de populações de Rotylenchulus reniformis (Nemata: Rotylenchulinae) do Brasil. Fitopatologia Brasileira 28:292-297.
Stanton, J. and W.E. ODonnell. 1998. Assessment of the North Carolina differential host test for the identification of Australian populations of root-knot nematodes (Meloidogyne spp.). Australas. Plant pathol. 27:104-111.
Starr, J. L., Koenning, S. R., Kirkpatrick, T. L., Robinson, A. R., Roberts, P. A., and Nichols, R. L. 2007. The future of nematode management in cotton. Journal of Nematology 39:283-294.
Starr, J. L., and Mercer, C. F. 2009. Development of resistant varieties. PP 326-337 IN: R. N. Perry, M. Moens, and J. L. Starr (eds.). Root-knot Nematodes. Wallingford, UK: CABI Press
Starr, J. L., R. L. Nichols, E. Moresco, and C. W. Smith. 2009 Inheritance of resistance to Meloidogyne incognita in primitive cotton accessions. Journal of Nematology 41:IN Press
Steeves, R. M., T. C. Todd, J. S. Essig, and H. N. Trick. 2006. Transgenic soybeans expressing siRNAs specific to a major sperm protein gene suppress Heterodera glycines reproduction. Functional Plant Biology 33: 991-999.
Timper, P., C.C. Holbrook, and W.F. Anderson. 2003. Reproduction of Meloidogyne spp. on resistant peanut genotypes from three breeding programs. Journal of Nematology 35:417-421.
Tinius, C.N., Schmitt, D. P., and Carter, T. E. Jr. 1988. Response of a resistant/susceptible soybean blend to Heterodera glycines. Abstr. J. Nematol. 20:661.
Vadhera, I., J. Bhatt, and B. Shukla. 1999. Prevalence of a new race of Rotylenchulus reniformis in Madhya Pradesh. Indian Journal of Mycology and Plant Pathology 29:132- 134.
Van der Beek, J.G., J.A. Los, and L.P. Pijnacker. 1998. Cytology of arthenogenesis of five Meloidogyne species. Fundamental and Applied Nematology 21:393-399.
Van der Beek, J. G., P. W. Maas, G. J. W. Janssen, C. Zijlstra, and C. H. Van Silfhout. 1999. A pathotype system to describe intraspecific variation in pathogenicity of Meloidogyne chitwoodi. Journal of Nematology 31:386-392.
Van der Berg, E. 1978. The genus Rotylenchulus Linford & Oliveira 1940 (Rotylenchulinae: Nematoda) in South Africa. Phytophylactica 10:57-64.
Wang, C., and Roberts, P. A. 2006. Development of AFLP and derived CAPS markers for root-knot nematode resistance in cotton. Euphytica 152:185-186.
Wang, C., Ulloa, M., and Roberts, P. A. 2006c. Identification and mapping of microsatellite markers linked to a root-knot nematode resistance gene (rkn1) in Acala NemX cotton (Gossypium hirsutum L.). Theoretical and Applied Genetics 112:770-777.
Weaver, D. B., Lawrence, K. S., and Van Santen, E. 2007. Reniform nematode resistance in upland cotton germplasm. Crop Science 47:19-24.
Whitehead, A.G. 1968. Taxonomy of Meloidogyne (Nematoda: Heteroderidae) with descriptions of four new species. Trans. Zool. Soc. London 31:263-401.
Windham, G.L. 1998. Corn. Pp 335-358 IN: K. R. Barker, G. A. Pederson, and G. L. Windham (Eds). Plant nematode interactions. Madison, WI:Crop Science Society of America
Wrather, J. A., and S. R. Koenning. 2006. Estimates of disease effects on soybean yields in the United States 2003-2005. Journal of Nematology 38:173-180.
Wrather, A., and S. Koenning. 2009. Effects of Diseases on Soybean Yields in the United States 1996 to 2007. Plant Health Progress.
Xinlian Shen, Guillermo Van Becelacre, Pawan Kumar, Richard F. Davis, O. Lloyd May, Peng Chee. 2006.QTL mapping for resistance to root-knot nematodes in the M-120 RNR Upland cotton line (Gossypium hirsutum L.) of the Auburn 623 RNR source. Theoretical Applied Genetics DOI 10.1007/s00122-006-0401-4.
Yadav, B. C., K. Veluthambi, and K. Subramaniam. 2006. Host-generated double stranded RNA induces RNAi in plant-parasitic nematodes and protects the host from infection. Molecular and Biochemical Parasitology 148: 219-222.
Zhou, E., J. L. Starr, and C. W. Smith. 1999. Inheritance of resistance to Meloidogyne incognita in the cotton cultivar Acala NemaX. Journal of Nematology 31:584-585
Zhou, E., T. A. Wheeler, and J. L. Starr. 2000. Root galling and reproduction of Meloidogyne incognita populations from Texas on resistant cotton genotypes. Supplement to Journal of Nematology 32:513-518.