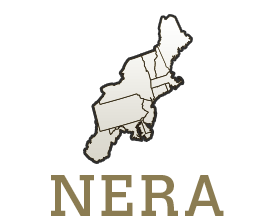
NE1038: Hydropedology: Genesis, Properties, and Distribution of Hydromorphic Soils
(Multistate Research Project)
Status: Inactive/Terminating
NE1038: Hydropedology: Genesis, Properties, and Distribution of Hydromorphic Soils
Duration: 10/01/2009 to 09/30/2014
Administrative Advisor(s):
NIFA Reps:
Non-Technical Summary
Statement of Issues and Justification
Because of the environmental and economic importance of riverine, coastal, and subaqueous resources as well as wetlands in the northeast US, pedologists working together under NE-1021 have been developing expertise in the identification, characterization, classification, and land use interpretations for hydromorphic soils (soils that have formed in the presence of excess water) within these areas. The key linkage among these soils and resources across the landscape is the hydrology. Understanding the integrated effects of the hydrologically-driven processes associated with saturation, inundation, and transport across the landscape is critical for the development of numerous soil based land use interpretations. For example, understanding soil morphology/water table relationships is critical in developing interpretations for the construction of buildings and roads, surface application of waste, the use of on-site waste disposal systems, and the function of various wetlands in storing carbon (C) and providing beneficial ecological services. Studies were initiated in the current NE-1021 project to address some of these problem areas. For example, data collected in the project indicated that hydric soil morphology in the Piedmont can be used to characterize soil hydroperiods with respect to the frequency of water table fluctuations and the vertical range in fluctuations. These hydroperiod characteristics have relevance not only to water table interpretations but also to wetland functions pertaining to water quality. The original work was done in the Piedmont. We propose to test this relationship in other physiographic regions in the northeast. Wetland delineation is a multi-million dollar industry. New techniques in landscape modeling are allowing investigators to identify wetlands using geospatial analysis and remote sensing (Pantaleoni et al, 2009a and 2009b). The improved mapping of wetlands is intended to improve the comprehensive National Wetland Inventory maps used as a basis for legislative decisions and wetland monitoring. A step in this goal is the recent formation of the Wetland mapping Consortium, sponsored by the Assn. of State Wetland Managers (http://www.aswm.org/swp/mapping/index.htm). Identification of hydric soils is essential in the on-site delineation process. Field indicators of hydric soils were developed to facilitate jurisdictional determinations of wetlands by identifying hydric soils (USDA-NRCS, 2006). Several field indicators rely on the quantity of soil C and may prove useful in predicting the amount of soil C in the surface of wetland soils. Some hydric soils, however, fail to develop the typical morphology found in most wetland soils. These so called "problem hydric soils" occur across the entire region and need to be addressed from a regional perspective (Vepraskas and Sprecher, 1997; Castenson, 2004; Zurheide, 2009). Across the United States efforts have been ongoing to develop regional supplements to the ACOE Wetland Delineation Manual (Environmental Laboratory, 1987). These supplements would provide guidelines for identifying hydric soils using regional hydric soil indicators. Based on our previous observations, several new indicators have been proposed for use within the northeast region. These proposed indicators will be tested in the proposed research under the monitoring guidelines of the National Technical Committee on Hydric Soils (2004). Our previous studies suggested that some of the monitoring guidelines used to identify reducing conditions were ineffective. Thus, in the proposed project, we will continue to test if monitoring protocols used to identify reducing conditions apply across the northeast. In particular, the viability and application of IRIS (Indicator of Reduction In Soils) technology will continue to be assessed. In our initial work under NE-1021, we developed amendments to Soil Taxonomy to accommodate subaqueous soils (Soil Survey Staff, in preparation), assembled a suite of terms to describe landscapes and parent materials for these soils of coastal systems (NCSS, 2005), and established the methodology to map the soils supporting these systems (Mapcoast, 2009). Now our focus shifts toward understanding freshwater subaqueous soils and building interpretations for subaqueous soils. Throughout the northeast federal, state, local agencies, and private companies have requested information relative to use and management of these resources for clam stocking, sustainable shellfish production, effects of dredging on benthic ecology, tidal marsh protection and creation, horseshoe crab habitat, submerged aquatic vegetation (SAV) restoration, and dune maintenance/replenishment. Although we have begun to investigate these relationships, there is still much to learn about subaqueous soils and the associated interpretations. There are still significant knowledge gaps regarding how these interpretations vary across the region. Essentially nothing is known regarding freshwater subaqueous soils. Our continued region-wide efforts will help develop subaqueous soil interpretations for the northeast region where both freshwater and coastal resources are in the forefront of use and management issues Our recent efforts have enabled us to improve our estimates of the amount of C stored in hydromorphic, wetland, and subaqueous soils in the northeastern US. Digital wetland inventory maps can be used to project soil C values using hydric soil indicators or major wetland types. This knowledge base can be used to assign C storage potentials to wetlands in various conditions from pristine to degraded. Our knowledge base is still quite limited regarding carbon sequestration rates, how these rates change over time, and how the effects of land use/cover change on C pools can be mitigated. In the proposed investigations we will focus on estimating soil C pools and sequestration across a range of soils and landscapes: including upland systems, man-made marshes, and subaqueous wetland systems. Our goal is to be able model the effects of land use and climate change on soil C pools across the subaerial and subaqueous continuum. The current NE-1021 project has established a framework for the systematic study of hydromorphic, hydric, and subaqueous soils across the northeastern US. Results from the proposed extension of NE-1021 will help us to develop quantitative spatial models to predict and represent the landscape distribution of hydromorphic, hydric, and subaqueous soils and hydromorphic soil properties. A continuation of this project will provide a forum to advance our knowledge of these systems and the associated soils and provide an outlet for the dissemination of our knowledge across the region to stakeholders that are seeking answers to their use and management questions. Working within the proposed regional framework will allow for testing of hypotheses across climatic gradients, across parent material types (coastal plain, residual, and glacial), and among different types and settings of coastal, riverine, and subaqueous soils. Testing these hypotheses is not possible for a single investigator working within a single state and must be done at the regional level. Addressing these questions within a regional framework is also critical because the major agencies that use the soils information that pedologists collect, such as USDA-NRCS, USACOE, USEPA, all work in a region-wide context. In addition, working groups such as the New England Hydric Soil Technical Committee and Mid-Atlantic Hydric Soils Committee (see NEHSTC, 2004 and MAHSC, 2004 as examples of their work), who offer guidance to regional regulatory bodies like the New England Water Pollution Control Commission (http://www.neiwpcc.org/), need soils information that is not restricted by state boundaries. Recent focus of the USACOE and other federal agencies to develop regional supplements as amendments to the 1987 Wetlands Delineation Manual (Environmental Laboratory, 1987) provide additional incentive to work region-wide in applied research. Data gathered, relationships that are established, and interpretations that are made are therefore much more meaningful to the user if the science was tested within a region-wide context.
Related, Current and Previous Work
The proposed project is a continuation of NE-1021 which set out to develop a framework for the integration of studies investigating soils across the landscape from a hydropedological approach throughout the northeastern US. This project is aimed at improving our understanding of the processes, characteristics, and interpretations of hydromorphic, hydric, and subaqueous soils, and to inventory wetland and soil carbon resources using field indicators of hydric soils, geospatial modeling, and remote sensing. The NE-1021 project has a web site to keep track of project activities, maintain standard protocols, and highlight project accomplishments http://www.caf.wvu.edu/plsc/ne1021/index.htm. The web site is housed at West Virginia University and maintained by Dr. Jim Thompson. We will continue to use the website to help maintain strong ties and partnerships within the project participants and serve as a source of information to those interested in the application of pedology to environmental and land use issues in the northeast.
Objectives
-
Evaluate the potential use of field indicators of hydric soils to characterize wetland hydroperiods with respect to frequency, depth, and duration of water table fluctuations; test the effectiveness of proposed hydric soil indicators to identify 'problem hydric soils'; test monitoring protocols used to identify reducing conditions to determine if they are effective within a range of soil conditions within the northeast; and investigate the hydraulic properties of hydromorphic soils with episaturation.
-
Initiate the development of a set of subaqueous soil-based use and management interpretations for applications in shallow-subtidal habitats of the northeast; investigate the spatial extent freshwater subaqueous soils in riverine settings in the northeast; and document the physical, chemical, and morphological properties of freshwater subaqueous soils.
-
Quantify and better understand carbon pools in a range of hydromorphic, wetland, created wetland, and subaqueous soil settings; test the relationship between surface soil C and field indicators of hydric soils; and test the application of various digital geospatial analysis tools and related statistical analysis to model C-pools across the landscape based on point and polygonal carbon data.
Methods
We are currently in the last year of the five-year multistate project (NE-1021). At recent NE-1021 meetings we discussed the research outlined in this proposal and where the various investigators felt their efforts and expertise should be applied. The focus areas were designed based on the objectives of the project and the expertise of the participants in our current project. These focus areas, however, are meant to be flexible so that new collaborators may join the project. HYDROPEDOLOGY FOCUS AREA (INVESTIGATORS) Hydric and Hydromorphic Soil Conditions and Indicators: (O'Geen, Rabenhorst, Stolt, Thompson, Vasilas, Veneman) Subaqueous Soil Interpretations and Freshwater Subaqueous Soil Identification and Characterization: (Drohan, Rabenhorst, Stolt, Thompson) Carbon Pools, Sequestration, and Spatial Distribution: (Galbraith, Thompson, Needelman, Lin) Our basic premise is that both soil and water are connected across the landscape in a seamless pattern from the seasonally-saturated soils of the upland to the continuously inundated subaqueous soils along the coast or river. Thus, by taking a hydropedological approach across the northeast region, we hope to study the cause and effect relationships between water and soil along this continuum. To do this, we will study a gradient of representative soils based on wetness. Sites will be chosen so that representative areas across the region are studied. Standard procedures, protocols and analytical methods will be followed by each investigator to insure that results are comparable across the region. These methods are detailed below. HYDRIC AND HYDROMORPHIC SOIL CONDITIONS AND INDICATORS Recent research led to the proposal of two new hydric soil indicators for the northeast. These two indicators, Mesic Spodic and Sandy Redox, were proposed as test indicators to the National Technical Committee for Hydric Soils. In addition, several other indicators and hydromorphic soils are being investigated (prior to submittal of another proposal or are currently listed as a test indicator because of their problematic nature) including young dunal deposits in barrier island systems, soils derived from red Triassic or Paleozoic sediments, soils having episaturation, clayey soils, and certain soils in floodplains. We will select representative sites in cooperation with NRCS personnel and members of the federal and regional hydric soil committees to monitor. Sites chosen to be monitored will be representative of the indicators and/or soil conditions of concern. At least three sites will be chosen to monitor for each indicator and/or condition of concern. For hydric soil indicators, we will monitor areas of the landscape that are obviously wetland, obviously upland, and a transitional area. Each site will be monitored at each of these locations. Selected sites (Coastal Plain and Allegheny Mountains) will be chosen to evaluate the relationship between Field Indicators of Hydric Soils and hydroperiod characteristics related to water table fluctuations. In particular, we will focus on hydric soil indicators and duration of saturation. Each site will be instrumented and monitored using methods similar to those described by Faulkner et al. (1989) and NHSTC (2004). Wells will be constructed of slotted PVC pipe with a well point on one end and a PVC cap on the other. Each well will be equipped with a Maximum Water Table Level Device to measure water table levels (Morgan and Stolt, 2004). We will use data loggers set at selected locations set at 6 hour intervals to establish water table rise and fall rates following precipitation. The loggers will be rotated among sites until a pattern has been established between precipitation and rise in the water table level. Precipitation amounts will be recorded with rain gauges and climate data collected at nearby weather stations. Soil temperature will be measured at each site at depths of at 12.5 cm in sandy soils, 25 cm in loamy soils, and 50 cm in all soils with a soil thermometer. Selected sites will have temperature monitored with temperature loggers. Atmospheric temperatures will also be recorded at sites that are more than 30 km from a climate station. Bud scores will be documented at the beginning of each growing season at the hydric soil indicator sites. Detailed vegetation data will be collected at each monitoring station. Redox potential will be measured at 12.5 cm in sandy soils, 25 cm in loamy soils at each location. Five platinum electrodes will be used to determine average redox potential at a given location. Redox potential will be measured with voltmeter that is attached to a reference electrode (calomel cell) and the platinum electrode. A salt agar bridge will be installed at each location for the calomel cell. Samples will be collected at 12.5 cm in sandy soils, 25 cm in loamy soils to test for ferrous Fe using alpha-alpha dipyridyl. Each monitoring station will have a detailed profile description. Samples will be collected from the depths that redox potential measurements are being made to determine soil pH and organic carbon content. Selected sites will be sampled to examine DCB and ammonium oxalate extractable Fe. Over the last four to five years, IRIS technology has been developed and work has focused on understanding how to efficiently make the IRIS devices and what principles guide their use and application (Rabenhorst and Castenson. 2005; Rabenhorst and Burch. 2006; Jenkinson and Franzmeier. 2006; Rabenhorst et al., 2008a; Rabenhorst et al, 2008b). Although some work has been done to compare the performance of IRIS devices to other standards for redox assessment (Castenson and Rabenhorst, 2006) there is a need for more thorough assessment and documentation. Selected landscapes will be identified where soils transition between better drained upland settings and more poorly drained wetland settings. Along these transects, study sites will be identified where IRIS devices will be installed according to recently developed protocols (Rabenhorst, 2008) and data will also be collected using other approaches for documenting reducing conditions such as Eh measurements using Pt electrodes. This should result in a more thorough understanding regarding the appropriate way to understand and to interpret the data generated from the use of IRIS tubes. At a fundamental level, we understand the necessity for soils to be saturated in order for reducing conditions to develop as soil microorganisms oxidize soil organic matter which results in the onset of anaerobic, or more reducing conditions. In principle, we would expect that greater quantities of organic matter in the soil would result in a more rapid onset of reduction. Preliminary experimentation has produced mixed results with some data confirming this postulation but other data confounding it (Jaffe, 2008). Further experiments will be conducted and study units will be stratified by soil landscape, in order to eliminate some of the variation observed in prior experiments and to better describe the relationship between these factors. Therefore, soil samples with various levels of organic carbon will be collected from A horizons from soils situated along a topohydrosequence. These soil materials will be used to construct mesocosms for evaluating the effect of soil organic carbon on the development of reducing conditions. Saturated mesocosms will be monitored for Eh, pH and for reaction with alpha-alpha-dipyridyl so that the onset of reducing conditions can be compared with the levels of soil organic carbon. For the hydromorphic soils having episaturation, infiltration and hydraulic conductivity (Ksat) will be measured using double ring infiltrometers, tension infiltrometers, and compact constant head permeameters (Amoozemeters). Field studies using dye and/or other chemical tracers will also be conducted to assess the role of natural structure and preferential flow (particularly as related to the presence of restrictive horizons such as fragipans) on water movement through soilscapes. SUBAQUEOUS SOILS Over the last 10 years, we have developed methods and procedures for identifying, classifying, and mapping estuarine subaqueous soils and landscapes. In this study, we will continue to expand our knowledge of estuarine subaqueous soils, begin to develop soils information regarding freshwater subaqueous soils, and initiate the development of a set of interpretations from soils data for management applications in shallow-subtidal habitats (use and management interpretations). Sites will be chosen to match these goals. Order 3 preliminary mapping of regional freshwater subaqueous soil distribution will be conducted using existing freshwater wetland, lake, and riverine geomorphic data in conjunction with adjacent soils information derived from Soil Survey Geographic Database (SSURGO) data and geomorphic information (elevation, slope gradient, slope aspect, surface geology) derived in part from digital elevation model (DEM) data. Models of freshwater subaqueous soil distribution will be derived from water body specific cross sections used to collect topographic and parent material soil mapping information from the above data sets. Where used on linear flow networks, data mining transects will run perpendicular to flow direction and incorporate stream geomorphology data, watershed contributing area information, and parent material distribution on a watershed basis for upstream contributing areas per reach (Order 3 scale). Using existing parent material information of water body adjacent soils, and derived energy dynamics of water bodies (stream order for example) to infer potential geoturbation, and thus a less developed subaqueous soil, we will develop predictions of taxonomic classifications to the Great Group level across study areas. Field sampling of selected areas from the modeled region will be used to further refine taxonomic classification of studied soils to the family level (where possible or needed). Potential interpretations will be identified based on water system type (riverine, wetland, lake) and anticipated data user needs. Specific site selection for field monitoring of all subaqueous soil environments will be based on a number of factors including: geomorphic setting; depositional environment; presence, absence, density, and type of SAV; type of wetland, lake, riverine system, estuary or subestuary; and the types of landforms and soil-parent materials on the adjacent upland environment. Each site chosen to study should be representative of the water bodies in the study area. Terminology for landforms, parent materials, and taxonomic classification of the soils will follow those established by Soil Survey Staff (2002), NCSS (2005), and Soil Survey Staff (in press). Amendments to the terminology will be discussed and agreed upon among the project participants.A submerged topography (bathymetric) map, at a minimum scale of 1:10,000-scale and with contour intervals of 30 cm or less, will be constructed for each study site (Demas, 1998; Bradley, 2001). These bathymetric maps will be used as a base map to delineate landforms and landscape units (Demas and Rabenhorst, 1998; Bradley and Stolt, 2002) and to derive landscape attributes such as percent slope, land-surface shape, and geographic location. Soils will be examined at multiple locations within each landscape unit. Sampling locations will be chosen in order to capture both the variability and extent of soil types within each landscape unit. Sampling will be done either by wading or from a boat. The spatial location of each description will be recorded using a Global Positioning System (GPS). Soils will be described using samples collected with a standard bucket auger. For very soft and fluid material (high n-value soils) and organic soils, a MacCauley peat sampler will be used. Based on these descriptions, representative soils will be sampled for laboratory analysis from each landscape unit using a vibracorer (Lanesky et al., 1979), standard bucket auger, or a McCauley peat sampler. Core barrels will be cut open length-wise and the soils described following standard procedures (Soil Survey Staff, 1993). All soil samples (vibracore, bucket auger, and McCauley sampler) will be frozen until needed for lab analysis.
Soil samples will be analyzed for percent coarse fragments, percent shell fragments (larger than 2 mm), pH, electrical conductivity, particle size distribution (PSD), levels of sulfides, CaCO3, and organic carbon. Particle size analysis will follow methods outlined in Gee and Bauder (1986). Salts will be removed through dialysis tubing. The clay fraction will be determined by pipette and sand fractions separated by sieving. Percent coarse fragments (rocks and shell fragments larger than 2 mm) will be determined by weight. We will make measurements of electrical conductivity and pH following standard and modified Soil Survey Staff (2004) guidelines. Samples will be moist incubated at room temperature for approximately 2 to 4 months. During this time the pH will be recorded on a weekly basis to determine potential acidity. Levels of organic carbon and CaCO3 will be determined using a C analyzer (ie. Carlo-Erba, Milan, Italy).
In regard to use and management interpretations, eelgrass productivity, shellfish productivity, and the effects of placing dredged materials on the land surface will be evaluated. Three different delineations of the same soil-landscape unit will be investigated to address dispersion and to allow for statistical analysis. Delineations and sampling locations within delineations will be chosen at random from the selected soil-landscape units. All of the sampling and monitoring will be done within a 15 meter radius of the sampling location. All locations will be recorded using a Global Positioning System (GPS). At each soil-landscape unit, eelgrass cover will be quantified by using a line-intercept transect (Bauer, 1943). Presence or absence of eelgrass will be noted by a diver at one-meter intervals along a fifteen-meter transect line. Three random transects estimates will be averaged for each delineation. Relationships between landscape units, eelgrass density, and soil properties will be assessed. Shellfish productivity will be estimated using a mark and recapture study design. 2 m x 2 m plots will be established at each sampling location. Change in shell length over the 15 week period will provide a measure of the growth rate (Grizzle and Morin, 1989). The effect of placing dredge materials on the soil surface will be evaluated using mesocosms experiments set up in the greenhouse. The mesocosms will be composed of 60 cm long 10 cm in diameter PVC columns packed with subaqueous soil materials collected from the upper 50 cm of selected soil-landscape units from each estuary. Soil materials will be thoroughly mixed and packed in the columns to reach a bulk density of approximately 1.0 to 1.2 g cm-3. Mesocosms will be left out-of-doors in an open, yet, protected location. Leachate will be collected and analyzed for pH, salinity, and pH. After oxidation and leaching, the soil materials placed in the mesocosms will be described and compared to the initial materials. Mesocosms samples will be analyzed for particle-size distribution, organic carbon, CRS, AVS, pH, potential acidity, and salinity before and after (with the exception of particle-size analysis) the oxidation and leaching experiments. CARBON SEQUESTRATION Terrestrial (subaerial) soils play an important role in the global carbon cycle in that they store large amounts of carbon, and if managed to do so, may provide an enhanced sink for atmospheric CO2 (Lal et al., 1998; Houghton et al., 1999; Ingram and Fernandes, 2001). Our recent studies (Jespersen and Osher, 2007; Mapcoast, 2009), suggest that subaqueous soils may also be a large and essentially unaccounted for sink for photsynthetically-fixed C in global C budgets. In the proposed studies, we will examine C sequestration along the hydrologic gradient across the region. Potential sites will be screened based on soils and land use data. GIS layers will be utilized in the initial screening procedure. Soils that have a considerable extent within the area will be considered first for study. Recognizing a systematic relationship will allow for modeling soil organic C (SOC) stocks that have not been adequately identified and enumerated using soil survey information. Landscape scale estimates of soil C will be derived from polygon-based (SSURGO) and point-based (from USDA-NRCS and other databases) data to produce complete and more accurate predictions of C storage. Environmental correlates to soil C (e.g., remotely sensed land use/land cover data, geology, terrain attributes derived from DEM, and climate data) will be used to improve spatial predictions of SOC. Spatial disaggregation techniques will be used to improve the spatial detail of predictions derived from the polygon data. For the point data, various pedometric techniques, such as linear regression, tree models, generalized linear models, geostatistical approaches, hybrid methods, and other multivariate statistical techniques will be tested. Soil C estimates developed from the point data and the polygon data will be combined using weights derived from the accuracies associated with each prediction method. Considerable carbon pool data are available for subaerial landscapes in the soil survey databases and from our recent NE regional C related projects. Although we have collected some subaqueous soil C information as part of the current NE-1021 project, there are still considerable knowledge gaps regarding differences in C pools among landscape units and estuaries. Therefore, we will continue to investigate whether SOC pools differ systematically within and among soil-landscape units of a range of estuaries. We will quantify C pools in the upper1 m of the subaqueous soils. Undisturbed samples will be collected with a split core sampler, vibracore (Lanesky et al. 1979), or McCauley peat sampler. Total carbon will be determined using an automated C analyzer (ie. Carlo-Erba, Milan, Italy; Nelson and Sommers, 1996). Bulk density measurements will be used to convert carbon values to a mass per area basis so that pools can be summed over the entire landscape (ie. Homann and Grigal, 1996). In estuarine settings, salt marshes store a large and significant amount of soil carbon (Rabenhorst, 1995; Chmura et al., 2003; Choi and Wang, 2004). Few data, however, are available regarding carbon pools and sequestration rates in restored marsh systems. Restored wetlands, created wetlands, and wetlands impacted by human and ecosystem stressors have lower C than pristine wetlands (Fajardo, 2006). In natural marshes, high net primary productivity and low decomposition rates lead to high carbon concentrations. If a marsh is able to accrete with sea-level rise, exceptionally high carbon sequestration rates can occur relative to other terrestrial systems. Sequestration rates in restored marshes may be higher than in natural marshes if factors such as increased nutrient availability lead to increased net primary production without causing an increase in decomposition rates. However, rates may be lower if the restored marshes do not have the same net primary productivity of natural marshes. Methane emissions may offset carbon sequestration in some tidal marshes. In this study we will examine C dynamics in plots of restored and natural marshes. Feldspar markers and shallow elevation benchmarks will used to measure carbon sequestration. Soils will be sampled by horizon and analyzed for bulk density, carbon, and total N. Vegetation will be sampled and analyzed for dry mass, carbon, and total nitrogen.
Measurement of Progress and Results
Outputs
- An annual project report highlighting the results for the previous year will be made available on the project website, and forwarded to participants in the related project focus areas identified above.
- Participants will submit appropriate research findings for publication in peer reviewed journals.
- Any amendments related to Soil Taxonomy, National Indicators of Hydric Soils, or other soil survey related documents will be composed and submitted for consideration.
- Research sites will be incorporated into bi-annual Northeast Soil Genesis and Soil Survey Work Planning Conference fieldtrips. These fieldtrips rotate throughout the region and run on opposite years.
- A final report will be issued at the conclusion of the project.
Outcomes or Projected Impacts
- This research will result in improved region-wide guidelines for the identification of soils that although wet, do not display typical hydromorphic features associated with seasonally saturated or hydric soils. New hydric soil indicators will be proposed and submitted for inclusion as part of the National Indicators of Hydric Soils: Northeast Supplement. Research focused on approaches to measure reducing conditions within soils may have significant impact on how reducing conditions within wetlands are measured and evaluated.
- Subaqueous soil characterization data and inventories will augment soil survey data and mapping outputs from MLRA soil survey offices within the region.
- The subaqueous soils soil interpretation research results will begin to build a set of soil interpretations for subaqueous soils which at the present time is completely lacking.
- The C data from the soil resource inventories will allow for better estimates of carbon pools and sequestration along a landscape level gradient upland to subaqueous. We will also begin to understand the dynamics of C in anthropogenic systems such as restored wetlands.
Milestones
(2010): Update project website with new project objectives and research deliverables for communication to the committee members. Organize a meeting for all participants to attend. Coordinate participants within specific research focus areas based on their expertise and interests. Begin to evaluate field sites using reconnaissance surveys to identify appropriate sites for detailed studies.(2011): Establish appropriate field sites for detailed studies based on reconnaissance surveys. Establish research plots for subaqueous soil interpretations. Formulate plans and strategies for instrumentation, mapping, and sampling. Visit selected sites during biannual Northeast Pedology Fieldtrip. Meet to discuss coordination and project establishment. Update web site to include site information and discussions during the regional fieldtrip to selected sites.
(2012): Maintain monitoring and mapping. Describe and sample soils within various hydropedological entities (i.e. subaqueous, hydromorphic, hydric, and restored). Begin characterization efforts. Analyze morphologic data from hydric and seasonally saturated soils with site specific monitoring data. Collect and analyze carbon sequestration data. Monitor and sample plot level experiments. Visit selected sites during region soil survey work planning conference tours. Update web site to include site and monitoring information and discussions during the fieldtrip to selected sites.
(2013): Continue to monitor at instrumented sites.. Continue characterization efforts. Continue to analyze morphologic data from hydric and seasonally saturated soils with hydrologic data. Collect and analyze carbon sequestration data. Monitor and sample plot level experiments. Visit selected sites during biannual Northeast Pedology Fieldtrip. Update web site to include new monitoring and analytical information and discussions during the regional fieldtrip to selected sites.
(2014): Complete analysis, synthesize results across the region, and write final report and other output works.
Projected Participation
View Appendix E: ParticipationOutreach Plan
Results from the proposed multistate project activities will be published as project reports, on the project web site, and as peer-reviewed publications. Participating members involved in undergraduate teaching, graduate student advisement, and extension activities associated with Land Grant Universities will promote the general dissemination of knowledge developed from the proposed project activities. Research sites will be visited on local, regional, and national pedology, hydric soil, and soil-environmental science fieldtrips and workshops. Northeast Pedology Fieldtrips have been run at least every two years since 1985. Participants include National Cooperative Soil Survey personnel from the NE region and graduate students from participating schools. Fieldtrips are run every other year during the region soil survey work planning conferences. Annual fieldtrips are also run by the New England Hydric Soils Technical Committee and the Mid-Atlantic Hydric Soils Committee. These committees are made up of university faculty, consulting soil scientists, NRCS soil scientists, and state and regional regulators.
Organization/Governance
The core membership in the multi-state project will likely come from the current NE-1021 Multistate project including: Patrick Drohan (Penn State University), John Galbraith (Virginia Tech), Henry Lin (Penn State University), Brian Needelman (University of Maryland), Anthony OGeen (University of California-Davis), Martin Rabenhorst (University of Maryland), Mark Stolt (University of Rhode Island), James Thompson (West Virginia University), Bruce Vasilas (University of Delaware), and Peter Veneman (University of Massachusetts). A Chair, a Chair-elect, and a Secretary will be selected from the above participants. Representatives from the member institutions will meet at least annually to assign tasks and review progress on the current research project. Additional participants with expertise in pedology, mineralogy, soil ecology, hydrology, soil-environmental science, and other related disciplines will be invited to join the project.
Literature Cited
Bauer, H.L. 1943. The statistical analysis of chaparral and other plant communities by mean of transect samples. Ecol. 24:45-60. Bradley, M.P. 2001. Subaqueous soils and subtidal wetlands in Rhode Island. Masters Thesis. Dept. of Nat. Res. Sci., Univ. of Rhode Island, Kingston, RI. Bradley, M.P., and M.H. Stolt. 2002. Evaluating methods to create a base map for a subaqueous soil inventory. Soil Sci. 167:222-228. Castenson, K. L. 2004. Hydromorphology of Piedmont Floodplain Soils. M.S. Thesis. Univ. of Maryland, College Park, MD. 256pp. Castenson, K. L. and M. C. Rabenhorst. 2006. Indicator of reduction in soil (IRIS): Evaluation of a new approach for assessing reduced conditions in soil. Soil Sci. Soc. Am. J 70: 1222-1226. Chmura, G. L., S.C. Anisfeld, D.R. Cahoon, and J.C. Lynch. 2003. Global carbon sequestration in tidal, saline wetland soils. Global Biogeochemical Cycles 17(4): 1111. Choi, Y. and Y. Wang. 2004. Dynamics of carbon sequestration in a coastal wetland using radiocarbon measurements, Global Biogeochemical Cycles 18: GB4016, Demas, G.P. 1998. Subaqueous soil of Sinepuxent Bay, Maryland. PhD Dissertation. Department of Natural Resource Sciences and Landscape Architecture, Univ. of Maryland, College Park, MD Demas, G.P., and M.C. Rabenhorst. 1998. Subaqueous soils: a resource inventory protocol. In Proceedings of the World Congress of Soil Science, 16th. August 20-26th 1998, Symposium 17 on CD. Ann. Meeting Intern. Soc. of Soil Sci., Montpelier, France. Environmental Laboratory. 1987. Corps of Engineers wetland delineation manual. U.S. Army Engineer Waterways Experiment Station, Vicksburg, MS, USA. Technical Report Y-87-1. On-line at: http://el.erdc.usace.army.mil/elpubs/pdf/wlman87.pdf Faulkner, S. P., W. H. Patrick, Jr., and R. P. Gambrell. 1989. Field techniques for measuring wetland soil parameters. Soil Sci. Soc. Am. J. 53:883-890. Fajardo, G. I. 2006. Physical and Chemical Soil Properties of Ten Virginia Department of Transportation (VDOT) Mitigation Wetlands. Electronic Masters Thesis etd-02212006-173715, Virginia Tech, Blacksburg, VA. On-le at: http://scholar.lib.vt.edu/theses/available/etd-02212006-173715/ Gee, G.W. and J.W. Bauder. 1986. Particle size analysis. p. 383-409. In Klute, A (ed). Methods of Soil Analysis: Physical and Mineralogical Methods, Part 1, 2nd edition. Soil Sci. Soc. Am., Madison, WI. Grizzle, R.E., and P.J. Morin. 1989. Effects of tidal currents, seston, and bottom sediments on growth of Mercenaria mercenaria: results of a field experiment. Marine Biology 102:85-93. Homann, P.S., and D.F. Grigal. 1996. Below-ground organic carbon and decomposition potential in a field-forest glacial-outwash landscape. Biology and Fertility of Soils. 23:207-214. Houghton, R.A., J.L. Hackler, and K.T. Lawrence. 1999. The U.S. carbon budget: Contributions from land-use change. Science. 285:574-578. Ingram, J.S.I., and E.C.M. Fernandes. 2001. Managing carbon sequestration in soils: concepts and terminology. Agric., Ecosys. and Envir. 87:111117. Jaffe, V. B., M. C. Rabenhorst, B. A. Needelman, and B. R. James. 2008. Effect of Temperature and Organic Carbon on the Development of Reducing Conditions in Soils. Soil Sci. Soc. of Am. Meetings (Houston, TX) Oct. 5-9, Annual Meeting Abstr. Jenkinson, B.J., and D.P. Franzmeier. 2006. Development and evaluation of Fe-coated tubes that indicate reduction in soils. Soil Sci. Soc. Am. J. 70: 183-191. Jespersen, J., and L. Osher. 2007. Carbon storage in the soils of a mesotidal Gulf of Maine estuary. Soil Sci. Soc. Am. J. 71:372379. Lal, R., J. Kimble, R.F. Follett. 1998. Pedospheric processes and the carbon cycle. In Lal, R., J. Kimble, R.F. Follett, B.A. Stewart (eds.). Soil processes and the carbon cycle. Advances in Soil Science Series. CRC Press, Boston, MA. Lanesky, D.E., B.W. Logan, R.G. Brown, and A.C. Hine. 1979. A new approach to portable vibracoring underwater and on land. Journal of Sedimentary Petrology 49:654-657. Mapping Partnership for Coastal Soils and Sediments. 2009. Soil Survey Data for Ninigret Pond. http://www.ci.uri.edu/projects/mapcoast/data.html; accessed January 30, 2009. Mid-Atlantic Hydric Soils Committee 2004. A guide to hydric soils in the Mid-Atlantic Region, ver. 1.0. L.M. Vasilas and B.L. Vasilas (eds.). USDA, NRCS, Morgantown, WV. . http://www.wv.nrcs.usda.gov/mlra/04_mayMidAtlSoils.pdf Morgan, C.P., and M.H. Stolt. 2004. A comparison of several approaches to monitor water table fluctuations. Soil Sci. Soc. Am. J. 68:562-566. National Cooperative Soil Survey. 2005. Glossary of terms for subaqueous soils, landscapes, landforms, and parent materials of estuaries and lagoons. Subaqueous Soils Committee Report, National Cooperative Soil Survey http://nesoil.com/sas/Glossary-Subaqueous%20Soils.pdf; accessed January, 30. 2009. National Technical Committee for Hydric Soils. 2004. Technical Note 11: Technical Standards for Hydric Soils. http://soils.usda.gov/use/hydric/ntchs/tech_notes/index.html Nelson, D.W. and L.E. Sommers. 1996. Total carbon, organic carbon and organic matter. p. 961-1010 In D.L. Sparks et al. (eds.), Methods of soil analysis. Part 3; Chemical Methods, Book Series 5. Soil Sci. Soc. Am., Madison, WI. New England Hydric Soils Technical Committee. 2004. Field Indicators for Identifying Hydric Soils in New England, New England Interstate Water Pollution Control Commission. Wilmington, MA. Pantaleoni, E., R. Wynne, J. Galbraith, and J. Campbell. 2009. A logit model for predicting wetland location using ASTER and GIS. Inter. J. of Rem. Sens. Accpt. 2008. Pantaleoni, E., R. Wynne, J. Galbraith, and J. Campbell. 2009. A comparison of CART and logistic regression for mapping wetland types in the Coastal Plain of Virginia using the ASTER sensor. Inter. J. of Rem. Sens. Accpt. 2008. Rabenhorst, M. C. 1995. Carbon storage in tidal marsh soils. pp 93-103. In Lal, Kimble, E. Levine B.. Stewart (eds.) Soils and Global Change. Proceedings of the International Soil Symposium on Greenhouse Gases Carbon Sequestration. Columbus, Ohio. April 5-9, 1993. Lewis Publishers, CRC, Boca Raton. Rabenhorst, M. C. and K. L. Castenson. 2005. Temperature Effects on Iron Reduction in a Hydric Soil. Soil Sci. 170: 734-742. Rabenhorst, M. C. and S. N. Burch. 2006. Synthetic Iron Oxides as an Indicator of Reduction in Soils (IRIS). Soil Sci. Soc. Am. J. 70: 1227-1236 Rabenhorst, M. C., R. R. Bourgault, and B. R. James. 2008a. Iron(III) (Hydr)Oxide Reduction in Wetland Soils: Mineralogical composition of IRIS paints. Soil Sci. Soc. Am. J. 72: 18381842. Rabenhorst, M. C., Douglas W. Ming, Richard V. Morris, and D. C. Golden. 2008b. Synthesized Iron Oxides Used as a Tool for Documenting Reducing Conditions in Soils. Soil Sci. 173: 417-423. Rabenhorst, M. C. 2008. Protocol for Using and Interpreting IRIS Tubes. Soil Survey Horizons 49: 74-77. Soil Survey Staff. 2004. Soil survey laboratory methods manual. Soil Survey Investigations Report No. 42, Ver. 4.0. (Ed.): Rebecca Burt. USDA-NRCS, Washington, D.C. On-line at: ftp://ftp-fc.sc.egov.usda.gov/NSSC/Lab_Methods_Manual/SSIR42_2004_view.pd Soil Survey Staff. 1993. Soil survey manual. Agric. Handbook No. 18, USDA-NRCS, U.S. Govt. Print. Off., Washington, D.C. On-line at: http://soils.usda.gov/technical/manual Soil Survey Staff. 2002. Glossary of landforms and geologic materials. Part 629, National Soil Survey Handbook, USDA-NRCS, Nat. Soil Surv. Center, Lincoln, NE. On-line at: ftp://ftp-fc.sc.egov.usda.gov/NSSC/Soil_Survey_Handbook/629.doc United States Department of Agriculture, Natural Resources Conservation Service. 2006. Field Indicators of Hydric Soils in the United States, Version 6.0. G.W. Hurt and L.M. Vasilas (eds.). USDA-NRCS, in cooperation with the National. Technical Committee for Hydric Soils. Vepraskas, M. J. and S. W. Sprecher (eds). 1997. Aquic conditions and hydric soils: The problem soils. SSSA Special Publication No. 50, Soil Sci. Soc. Amer., Inc., Madison, WI. Zurheide, P. K. 2009. Hydromorphology of Anamalous Bright Loamy Soils on the Mid-Atlantic Coastal Plain. M.S. Thesis. Univ. of Maryland, College Park, MD. 317pp.