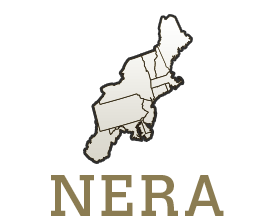
NE1037: Wood Utilization Research : Biofuels, Bioproducts, Hybrid Biomaterials Composites Production, and Traditional Forest Products
(Multistate Research Project)
Status: Inactive/Terminating
NE1037: Wood Utilization Research : Biofuels, Bioproducts, Hybrid Biomaterials Composites Production, and Traditional Forest Products
Duration: 08/01/2009 to 09/30/2011
Administrative Advisor(s):
NIFA Reps:
Non-Technical Summary
Statement of Issues and Justification
The Need
The United States is the world's largest producer and consumer of forest products, with US consumption of wood exceeding our consumption of metals, plastics and masonry cement - combined - each year. The forest products industry is a major contributor to the nation's economy. In 2004, the US sold approximately $260 billion of wood products, which comprised about six percent of the total US manufacturing output, and directly employed more than one million employees nationwide. However, the American forest products manufacturing industry is facing stiff international competition. The volume and value of imported manufactured forest products is increasing annually. American jobs in many traditional wood and paper industry sectors are being lost, primarily to foreign competition. Because many forest products manufacturers are small-to-medium sized firms, and are located in rural areas or at the rural-urban interface, this foreign competition has also resulted in a disproportionate impact on rural economies. To remain competitive and to protect and expand our rural economies, American forest products producers must innovate.
Our current infrastructure and economy rely on foreign fossil-based fuels, power, and products, which presents a serious challenge to our economic stability and national security. The continuing and increasing consumption of fossil fuels also poses environmental challenges such as pollution and green house gas emissions. Viewed with a new perspective, these challenges also present excellent opportunities for the forest products industry. Our forests provide a vast storehouse of renewable domestic feedstock for production of biomass-derived fuels, power, and chemicals. Properly managed forests also provide important environmental benefits such as carbon sequestration, ecological balance, and wildlife habitats. The forests of our country have the potential to provide the biological resources needed to replace fossil-based energy and products with renewable, non-food based bio-energy resources and bio-products.
In President Bush's 2007 State of the Union address and the recently signed 2008 Farm Bill, significant changes were made in our energy policy to encourage the production of renewable and alternative fuels from non-food biomass. The Senate is expected to consider legislation to impose limits on greenhouse gas emissions and to allow companies to trade pollution credits. President Obama's administration is also expected to promote green energy initiatives. Significant scientific and engineering breakthroughs are required to realize the vision of replacing fossil-based energy and products with renewable bio-based fuels and products. At the same time, continued innovation in the use of biobased composites in construction and infrastructure applications, and development of advanced biomaterials requires efficient utilization of our forest-based resources. Research and educating students for the workforce are equally important if the US is to remain competitive in this traditional economic sector.
This project continues work initiated in NE 506. The objectives have been narrowed and focus on two interrelated areas: The development and application of innovative structural biomaterials from wood, lignocellulose and hybrid materials, and the production of new and innovative biofuels/biochemicals from wood.
Project goals include development of novel wood composites and hybrid wood, lignocellulose and polymer matrix composites. Research in this area requires initial study of the selection and harvesting of feedstock to define optimal processing methods for separating biomass that is best suited for use in structural biomaterials and biomass that will be used for conversion to biofuels and chemicals. Research on advanced engineered biocomposites is included to insure that this new feedstock can be used to produce new structural materials intended to optimize use of our native natural resources. Advancements in research on traditional wood products and composites, such as oriented strand board (OSB) and oriented strand lumber (OSL), will also be expanded, as will development of new polymer matrix composites and hybrid composites produced from inorganic/biomaterial mixtures. Additionally, we will seek novel applications for nanocomposite materials through the study of nanomaterials from wood, such as carbon nanotubes (CNTs) and cellulose nanofibers. Further, we will investigate the incorporation of nanoparticle fillers to enhance the strength and durability of composites, and explore the interfacial properties of composite materials at the nano scale to produce bio-based composites of greater strength.
Coupling the harvest and initial processing of biomass with the study of the production of nanomaterials and novel composites allows us to exploit unique opportunities in the area of biofuels and biochemical production from wood and lignocellulose biomass. One example is the extraction of hemicellulose from the furnish used in the production of OSB and other composite products. This wood furnish for products ranging from particleboard to OSB is produced with a large surface to volume ratio, providing an opportunity for ready extraction of compounds from the wood. Research shows that extracted strands used in the production of OSB actually offer some improved properties for panel production, encouraging extraction of a new feedstock for use in biofuels production [1]. In addition, we seek to develop efficient and environmentally benign biomass conversion processes for hemicellulose and other cellulosic by-products. Delignification processes using recyclable chemicals and select bioprocessing methods will be developed to produce lignin-free carbohydrates. Chemical and biological methods of pretreating and saccharifying lignocellulosic biomass for the production of fermentable sugars and new chemicals will be explored. Because most industrial ethanol production processes use only starch and glucose, modified fermentation processes and alternative technologies will be developed to efficiently ferment xylan-rich lignocellulosic materials for production of liquid and gas biofuels including bio-oil, ethanol and hydrogen.
In this collaborative multi-state research initiative, we will focus on two main areas: innovative structural biomaterials, and production of biofuel/biochemicals from wood. These areas encompass a very wide range of topics, from research on sustainable wood harvesting methods, production of value-added biomaterials including biofuels, bioconversion processes, fermentative processes, nano-technologies, new biocomposite product development. The value of working on solid structural biomaterials on the same multistate project with the development of liquid and gaseous fuels is that processing efficiencies can be achieved when byproducts or waste residues from one process are used for the other. Process efficiencies will be explored as part of all components of research undertaken in this proposal.
The overarching theme is development of novel and efficient uses for traditional forest products. Participation in this integrated research effort will extend the capabilities of several research groups at different universities, further our understanding of wood science and utilization, and ultimately reinvigorate our forest products industry through technological innovations in processes, materials, and markets.
The Importance of the Work
The importance of wood utilization research at the National level has been highlighted in a 2006 United States General Accountability Office report [2] that identified national needs and recognized that research and education in this field are crucial to ensuring proper utilization of wood, a important natural resource and our nation's most widely used structural material.
Over the last 50 years, the United States has become dependent on foreign energy sources. With our shrinking petroleum base, it is critical that we develop our own biobased resources and develop structural materials that reduce our reliance on foreign energy sources. Development of advanced engineered wood composites is critical because these materials can replace energy-intensive construction materials like steel and concrete. Further, the development of biobased adhesives will allow wood composites, and advanced hybrid composites to be bonded together using forest-based derivatives. Development of hybrid structural composite products using nanoparticle reinforced thermoplastics combined with underutilized hardwoods holds great potential. Biopolymers and bioplastics from wood and other lignocellulose resources is another area that researchers in this Hatch multistate project will focus on.
A multistate effort focusing on new methods to produce biofuels, bioproducts and polymers from woody biomass through biological and chemical processes is needed to help focus our wood utilization efforts in the US on areas linked to efficient, yet sustainable, forest resource utilization. Similarly, continued development of new bio-based composite materials and improvement of traditional harvesting and milling techniques compatible with these materials is vital to the future of building construction in America. The production of the next generation of building products in the US must be done in an energy efficient and environmentally sustainable, manner. It is also important that production be done locally and regionally to reduce the costs and energy consumption involved in transportation, and to help rebuild the American economy. This project will focus on wood utilization research in several states, linking varied research programs in collaborations designed to ensure efficient and sustainable forest resource utilization. Benefits in addition to a reduction in dependence on foreign oil include economic stability and growth, increased industrial capacity, job creation and development of green technologies.
Technical Feasibility of the Research
The technical feasibility of the proposed research is high, with well-developed facilities and highly skilled personnel in the participating University programs. Some example University programs highlight the type of facilities available for this multistate effort:
The University of Maine is a leader in the field of wood composites and biobased products. The 48,000-ft2, ISO-accredited Advanced Engineered Wood Composites Center at the University of Maine is a state-of-the-art facility for integrated composite-materials/structural-component development with in-house capabilities for developing a composite product or structure from the conceptual stage through research, manufacturing of prototypes, comprehensive testing and evaluation, code approval and commercialization. The Center contains laboratories for composite materials manufacturing science, polymer/interface science, environmental-durability testing, mechanical testing, nondestructive evaluation (NDE), advanced microscopy, and large-scale multi-degree-of-freedom static and dynamic structural testing. The Center also houses two pilot plants: a Wood Plastic Composites Pilot Plant and an OSB/OSL Pilot Plant. It is currently undergoing a 12,000ft2 expansion with focused activities on nanocomposites and composites for renewable energy production
West Virginia is the third most heavily forested state with 12 million acres of forestland and more than 80% of its land in forest coverage. West Virginia has been successful in attracting wood products industries that use new technologies to better utilize Appalachian hardwoods. The West Virginia University Division of Forestry and Natural Resources has been leading the way in research and extension efforts that meet the needs of private and public sectors in West Virginia. West Virginia University provides research leadership to the forest products sector in the Appalachian region and increases the success of the primary processing and manufacturing sectors of the wood products industry.
The University of Tennessee has extensive research programs to serve agriculture and forestry producers. The Tennessee Forest Products Center is the only such full-time research facility among U.S. university programs. Undergraduate and graduate students are included in the research programming of the Center and gain valuable experience in conducting research. The Tennessee Forest Products Center houses a new 10,000 sq. ft. facility, which contains 3-1200 sq. ft. processing labs, a 30-person training facility, 3-500 sq. ft. wet labs, 1-500 sq. ft. PLC room, and offices for faculties and graduate students.
The University of Kentucky has an active research and extension program in wood utilization. The Robinson Forest is one of the largest research and educational forests in the United States, and is managed for research, teaching, and extension education by the University of Kentucky, Department of Forestry. In addition, the Wood Utilization Center in Kentucky was established in 1963, and has a 14,000 square foot facility that contains an industrial hardwood furniture manufacturing laboratory, with teaching space and a 10,000 board foot hardwood lumber dry kiln.
The University of Minnesota has a history of wood science related research and education dating back to the 1920s. Similar to other participating academic units nation wide, it is home to the Department of Bioproducts and Biosystems Engineering (BBE; formerly called the Department of Forest Products in the College of Natural Resources) and Natural Resources Research Institute (NRRI). The wood and forest products research in the BBE Department is primarily conducted in Kaufert Laboratory of Forest Products and Wood Science in St. Paul, which houses the Biofuels and Bioproducts Innovation Laboratory which has extensive biomass characterization and analytical facilities, biomass thermo-chemical and biochemical conversion capabilities, fungal culture laboratory certified by the American Type Culture Collection, Advanced Bio-based Materials Characterization Lab, basic particle-composite manufacturing facilities, and controlled environment laboratories. The University of Minnesota Imaging Center, Rheology Lab and Characterization Facility at the Twin Cities campus have state-of-the-art material characterization equipment and can be accessed at reasonable user fees, with experts available to provide assistance on equipment training and/or data interpretation.
The NRRI facility in Duluth Minnesota houses among others pilot plants for wood materials and composite making and testing.
Advantages of Conducting the Work as a Multi-State Effort
Multi-disciplinary research is needed to improve our understanding of the fundamentals of the key mechanisms for biomass production and conversion and to develop economically viable biofuels. At the same time, continued research is needed to advance composite technologies, particularly nano reinforcement of biobased products, to permit the wood products industry to remain competitive. However, research efforts in these areas are currently fragmented. The advantage of conducting this research as a Multistate Hatch project is that the synergistic effects of the larger group of researchers, who will share knowledge within related projects, will allow greater productivity and efficiency than could be achieved at any one of the University programs alone. Funding will permit the exchange of researchers and students from each of the programs, and facilitate the attendance of meetings where researchers from all Universities can share research results and discuss future research directions. In the proposed collaborations, shared research facilities and collaborative research would result in substantial cost savings to the participating Universities. As one example, the University of Tennessee does not have appropriate facilities for the development of pilot scale wood plastic composites (WPCs), hybrid composite materials composed of wood particles and polymers, which have seen a very large market acceptance in recent years. The University of Maine has State-of-the-Art pilot scale facilities for WPC production. University of Tennessee researchers have now been able to develop their research ideas and see the fruition of their bench chemistry results translated into large-scale products through collaboration with the University of Maine. This is just one example of the synergies that will be developed through the support of a joint multistate project in this area.
The Likely Impact of the Successful Completion of the Work
It is anticipated that completion of this multistate research project will bring together some of the best research minds and facilities for research on wood and biobased products in the United States. It is anticipated that the program will include participation from 17 States, from Hawaii to Maine and Alaska to Louisiana. The completed multistate research effort in this area will provide a comprehensive view of forest utilization, ranging from an improved understanding of utilization of southern pine and bottomland hardwoods in the south, to broader applications for underutilized species in the spruce fir forests of the north, and Douglas-fir in the west.
Through participation in this integrated multistate research effort, we hope to extend our capabilities allowing the participating University programs to contribute to an enhanced understanding of wood science and utilization. Even though each University program will focus on specific wood species and research projects, the basic understanding that will be developed in subfields ranging from wood processing for composites, to sustainable harvesting techniques, to biochemical and microbial processing will be transferable to the specific systems being researched at other member institutions. Sharing of the information through this proposed multistate Hatch project will foster streamlined relationships between the participating programs and it will provide the framework needed for collaborative research to be conducted by all University programs.
Related, Current and Previous Work
Previous research under Hatch Multistate project NE506 has advanced our understanding of wood science and wood utilization. Characterization of wood and biomass has been conducted at a number of US programs, both within and outside of the NE 506 project. The review below provides a sampling of research at some of these University-based programs.
At the University of Alaska, Fairbanks and at several other Universities [3], chemical analysis of fast growing wood species, such as birch, has allowed researchers to evaluate their potential for biofuels and biochemicals production. This research is linked to Near-Infrared Spectroscopy and multivariate analysis research focused at Washington State University [4], where wood scientists are testing the chemical traits of the trees to determine if the species can serve as feedstock for biofuels production. In addition, studies at Oregon State University on improved inventory analysis of biomass and monitoring of its flow are undergoing [3].
Research on isolation of hydrocarbons from woody materials is being developed at several universities with different, but interrelated, foci. Researchers at the University of Tennessee [5] and University of Maine [6, 7] have focused on the pre-extraction of biochemicals and biofuels from OSB flakes. In related research at the University of Maine [8], studies are being conducted on the selective cleavage of the lignin-carbohydrate linkages to produce lignin-free carbohydrates for high-value chemicals and biofuels. Additionally, enzymatic and non-enzymatic processes involved in wood degradation by fungi are being explored [9], which can selectively modify wood components for biofuels and biochemical production. Ongoing research at Iowa State University [10] is developing a novel delignification technology using recyclable chemicals. Research underway at the University of Kentucky [11, 12] is exploring the use of bacteria in saccharification of solid polysaccharide for ethanol production.
The quest for new and useful chemicals from plants is ongoing with efforts from different universities. The pharmaceutical properties of the bark of different birch species have been investigated by the University of Minnesota Duluth's Natural Resources Research Institute, in collaboration with the University of Alaska Fairbanks' School of Natural Resources and Agricultural Sciences [13]. Currently the raw materials for many pharmaceuticals are derived from petroleum, so the use of wood as a resource of fine chemicals offers the opportunity to reduce our dependence on oil.
Research on wood based biocomposites has focused on three major efforts. In the first biocomposites area, disaster resistant and blast resistant panels have been developed that help to protect humans from earthquake, tsunami and hurricane disaster, and ballistic attacks. Research at Oregon State University focuses on improvement of wood-framed structures' performance for safe residence [14, 15]. Related research in advanced wood-hybrid panels the Advanced Engineered Wood Composite's Center has developed blast-resistant structures with fiber reinforce polymer coated wood frame members for application in military force protection. Panels developed as part of this effort are currently being used in Afghanistan to help protect American troops there [16].
In the biocomposites area, research focused on wood plastic composite (WPC) enhancement is leading the way for the development of new hybrid materials which have high resistance to biologic degradation agents [17] and the ability to be molded to meet any desired spatial conditions. This will help in providing new materials for exterior applications, such as decking, siding, fencing and waterfront construction. Researchers at Oregon State University [18] and University of Maine [19] are developing new WPC products for high temperature applications, such as under-the-hood automobile components [20]. Studies on structural application of WPC are underway at both Washington State University [21] and Oregon State University [22].
Work is also continuing at several Universities on the application and incorporation of nanomaterials into composites. Synergies in research have focused on the incorporation of nanoclays and nanofillers into composite products to improve mechanical properties and durability. Researchers at the University of Tennessee have developed a new method for isolation of cellulose fibrils and focused on the mechanical properties of nanocomposites reinforced with cellulose fibrils [23]. Related research at Oregon State University [24] and University of Maine [25] focuses on cellulose nanocrystals for advanced materials application. Researchers have also discovered a unique process for producing carbon nanotubes, a highly valuable commodity, from wood fiber [26]. Synergies in these research areas are continuing to develop.
Objectives
-
Production of new and innovative biofuels/biochemicals from wood.
-
Development and application of innovative structural biomaterials from wood, lignocellulose and hybrid materials.
Methods
The methods and procedures involved in a project with multiple states involved and with interrelated objectives are necessarily complex, and an overview of the primary Methods and procedures is provided. Biofuels and Biochemicals: In the area of production of biochemicals and biofuels, the fundamental approach is to work with the basic components of lignocellulosic materials to produce products of value, either for structural application, or for use as a bio-based fuel or chemical. Fischer-Tropsch (FT) chemistry has been used successfully to produce bio-oil but further research must be conducted with lignocellulosic materials to move this process from the pilot scale to commercial scale. The major problems in using FT with biomass are the production of tar and gases that may poison the catalyst during the gasification process [27]. Efficient production methods will be explored to reduce the production of contaminants and increase the yield of hydrogen and carbon monoxide. New catalysts that have higher tolerance for contaminants will also be explored. In the biofuels bioconversion arena, lignocellulose and wood may be hydrolyzed to produce low molecular weight polysaccharides and sugars that can be fermented to ethanol or other transportable fuels. One of the challenges to this approach is developing economical methods to hydrolyze lignocellulose while maintaining the final product at a pH appropriate for fermentation. Alternate approaches, including the enzymatic hydrolysis of lignocellulosic materials rather than acid hydrolysis, will be explored to produce a variety of chemical products. Because they are able to modify and/or degrade cellulose, hemicellulose, and lignin wood components, fungi have long been utilized in wood processing and wood modification to increase efficiency and reduce production costs [28, 29]. Despite industrial interest, the mechanisms involved in wood modification and bioconversion by fungi and other microorganisms have yet to be fully characterized. A more complete understanding of the metabolic pathways employed by these organisms could drive innovation in wood processing. Typical methods employed to date in participating PIs laboratories and pilot-scale facilities for hemicellulose extraction are hot water and steam extraction. Depolymerization of the hemicellulose has been done by acid hydrolysis. As one aspect of the proposed research, these methods will be supplemented using bacteria and fungi to directly depolymerize cellulosic and lignin fractions. Selective white rot fungi will be used to degrade lignin while liberating cellulose for bioconversion. Brown rot fungi will be examined for their potential to rapidly depolymerize holocellulose for later bioconversion by bacteria or yeasts. Bacterial as well as algae will be used with lignocellulose for bioconversion with bacterial fermentation processes used to produce products ranging from ethanol to hydrogen gas, and algae grown heterotrophically for the production of bio-oil. Chlorella protothecoides is currently being grown heterotrophically to determine if algal bio-oils can be produced using wood substrates. This work is being conducted using conventional medium (Proteose modified Bristol's medium [30] for algal growth), supplemented with pentose sugars and hemicellulose extracts to determine the effects on bio-oil production. Continued studies in this area, as part of the proposed research, will be conducted. Specific technologies involved in analysis and characterization of biomodification and bioconversion will include X-ray diffraction (XRD) [31], HPLC wood sugar analysis [32], immmunoabsorbant enzyme assays [33] and tools specifically developed to quantitatively and qualitatively evaluate lignin structure [34]. Research has indicated that extraction of useful constituents from wood can be accomplished while still maintaining the necessary physical and chemical integrity of the residual wood for use in composites [35]. Ethanol production from wood hemicelluloses is commercially feasible. The fact that hemicellulose removal from wood can be accomplished simply, with heat and water, makes this alternative attractive industrially. Also, wood material with some percentage of the hemicelluloses removed can have improved properties and still be used in many other applications, such as the production of pulp, oriented strand board (OSB), and particle board [6, 36]. Recent studies conducted on the mold susceptibility of hemicellulose-extracted OSB have shown that extracted boards are less susceptible to molds and some decay fungi compared to non-extracted boards [31, 37]. This is presumably due to the removal of the hemicelluloses as a nutrient source, as well as removal of of toxic compounds possibly produced and potentially also due to the decrease in water availability. Fermentation will be performed as part of some of the research outlined in this proposal. Although most current technologies use genetically modified organisms (GMOs) in the fermentation of pentose sugars, such as xylose found in hemicelluloses, it is important to note that these GMOs are used only in contained reactors and are not released into the environment. Research on the development of value-added products from extracted residues will focus on process optimization for the creation of new biocomposites using chemically and biologically pre-treated lignocellulose. Evaluation of the resultant product properties will include characterization of strength, durability and adhesion. Measurements will include pore size, wettability, crystallinity and microstructure. Structural Biomaterials: In the area of development and application of innovative structural biomaterials from wood, lignocellulose and hybrid materials, common composite production methods, such as hot/cold pressing, extrusion, pultrusion, laminating and vacuum/pressure-assisted resin infusion, will be employed for the fabrication of new biocomposite products. Physical and mechanical property testing, and chemical properties evaluation will be adopted from ASTM standards [38-40] to evaluate material properties. Both experimental and numerical techniques will be used to analyze the structures and materials. For traditional wood-based composites, general mechanical properties, such as tensile properties, bending properties, shear properties, fracture properties, and bonding durability will be tested according to corresponding ASTM standards [41]. Because of the polymeric nature of wood, hygro-mechanical behavior and time-dependent properties of the composite materials will also be investigated. Surface modifications using chemical or physical approaches will be used to improve adhesion and interface/interphase performance of the composite materials. SEM, TEM, AFM will be used to examine the material structure at the micron-, submicron-, and nanometer-scale. The molecular-level properties of the composites will be investigated using FTIR, DMTA, and DSC. One approach to improve OSB, OSL and other strand composite products will be the use of finite element modeling to combine density variations, void distributions, strand properties, strand geometry and orientation into one model to obtain an accurate simulation of the composites. Methods that can reduce the cost of adhesives will also be explored. Research in this area includes the development of novel wood adhesives containing solid residues of biomass fermentations from the biofuels/biochemicals research, and surface activation of wood strands by plasma or strong oxidative agents to reduce or eliminate the use of petrochemical based adhesive systems. WPCs are a relatively new type of wood-based composite in the marketplace. They are highly desirable for their maintenance-free aspects and their ability to resist biological degradation agents. However, their use in structural applications has been limited because of their limited mechanical properties including lower impact resistance, In addition, their higher density compared to pure plastics and solid wood means has added undesirable weight to structures. The low strength of WPCs has been associated with poor compatibility between lignocellulosic fiber and thermoplastics, resulting in insufficient interfacial adhesion and poor dispersion of the wood fibers in the plastic matrix. Surface modification of wood fiber and coupling agents will be explored to improve compatibility between different phases in the composites [42]. Studies on the incorporation of naofibers/nanoparticles into WPCs to improve the interphase properties in the fiber and plastic matrix will be conducted to improve the mechanical properties of WPCs. Nano-sized cellulosic materials, whose density is lower than common inorganic nano-fillers will also be explored as a light-weight reinforcing agents. Approaches such as the development of hollow or porous WPC products to increase the specific strength of the materials will be explored as well. Research on nanomaterials will focus on the control of nanoscale structures and functionality. Specifically, research will focus on: 1) liberation of nanodimensional components, such as nanodimendional cellulose fibrils and macromolecules in woody materials; 2) nanoparticle-reinforced polymer composites; and 3) analytical methods for the characterization of nanostructures. Techniques developed for other nanomaterials will be used as platforms for creating new wood-based materials and products. In the first focus area, chemical and physical approaches will be used to isolate nanomaterials from wood. These approaches include acid hydrolysis, nano-grinding, and high intensity ultrasonic treatment. In the second area, a major challenge is to obtain adequately stable dispersion of nanoparticles in the polymer matrix. One approach will be to use solvent-based dispersion systems to facilitate the process. Existing methods such as using surfactant, adding dispersion agents, surface modification, ultrasonic and shear blending will be used to improve the dispersion and compatibility of the nanoparticles and the polymer matrix. Creation of novel, functional self-assembling surfaces on lignocellulosic substrates will be explored to produce self-assembled nanocomposites. In the third area, currently available physical and chemical property instrumentation used in nanoscience and nanotechnology will be adapted to research on lignocellulosic materials. SEM, TEM, AFM, STEM, XRD and other techniques, such as light scattering, rheology measurements, and three-dimensional imaging systems will be used to examine the properties of biomaterials from wood.Measurement of Progress and Results
Outputs
- Value-added, durable wood based composite materials with improved performance.
- Novel bio-nanomaterials with innovative properties including carbon nanotubes from lignocellulose fiber.
- Fuels and high-value chemicals from woody materials including ethanol, acid acid, and bio-oils.
Outcomes or Projected Impacts
- Increased sustainability of forest resources.
- Improved conversion efficiencies of wood based materials to final products.
- Promoting transformation of forest product industry from resource-based industry to knowledge-based industry.
- Graduate students and undergraduate students will be educated as part of this research and will receive research training to develop the next generation of cellulosic biofuels experts.
Milestones
(2009): Analysis of potential synergies and joint objectives Initiate studies in biomass conversion, modified fermentation processes, nanomaterials and the development of new wood composites(2010): Initiate coordinated focus on needed analytical capabilities Develop coordinated outreach plans
(2011): Midpoint evaluation of project research thrusts and project outcomes Refine milestones for individual projects Continued development of needed analytical and testing capabilities Optimization of bioconversion, nanotechnology, and fermentation processes and new biocomposite product development
(2012): Continuation of outreach and technical transfer initiatives Process optimizations and product testing for value added biomaterials, commercialization as appropriate
(2013): Program completion and evaluation Development of objectives for the next five years
Projected Participation
View Appendix E: ParticipationOutreach Plan
The outreach plan for this project will be tailored to meet multiple objectives, multiple target audiences, and short- to long-term goals. Specific objectives for outreach include awareness building among interested audiences, skill building, and technology transfer. The two primary target audiences are other scientists (in both academia and industry) and upper level industry managers and investors.
This project presents a unique opportunity for traditional Agricultural Scientists to collaborate with Wood Utilization Scientists, and promote synergy between these two groups in a way that has not been broadly realized in the past. For outreach directed to awareness building, traditional outreach tools such as technical publications, presentations at professional meetings, newsletter articles from participating institutions, and websites will be used. These efforts will be targeted to a broad audience, other scientists and industry personnel. The short-term goal of these efforts will be to ensure a broad audience is familiar with the research and its results. Longer-term outcomes will be to spark interest among a subset of the target audience in learning about the research, and its commercial potential, in more detail.
Outreach directed towards skill building may be seen as the 'next level' in engagement between the participating institutions and the target audience. Once research is at the stage for application, pilot demonstrations and workshops will be used for this form of outreach. These forms of outreach provide participants with a greater depth of understanding of the research and the abilities to apply what they have learned in their work. For scientists, the short- to mid-term goals for skill-building outreach will be to foster continued innovations in their research programs; for industry personnel, the goals are the implementation of product and process innovations in their companies.
Outreach focused on technology transfer is significantly different than that focused on awareness building or skill building. For technology transfer, one of the goals is public dissemination of information, and another mid- to long-term goal is commercialization of the research. As such, target audiences may include both industry scientists as well as potential investors when appropriate.
Organization/Governance
The University of Maine structured the original NE 506 proposal and was asked to continue this effort to develop the current proposal. Dean E. Ashworth will continue to serve as Station Director. Dr. F. Servello will serve as Administrative Advisor. Dr. Barry Goodell has served as the Scientific Coordinator and has been responsible for preparing proposals, coordinating annual meetings, generating interim and termination reports, and soliciting scientific input from all participants for NE 506. NE temp 1521 will be structured similarly and it is anticipated that Professor Goodell will coordinate efforts on this project initially until a Chair can be elected at the first formal meeting. It is anticipated that the two objectives would be assigned to two vice-Chairs to develop and coordinate project efforts in specific areas.
Major decisions will be brought to a vote before a committee of the whole, with each program having one vote.
Literature Cited
1.Paredes, J.J. and S. Shaler. Oriented strand board (OSB) from hot water extracted wood in ECOWOOD'08 the 3rd International Conference on Environmentally-Compatible Forest Products. 2008. Porto, Portugal.
2.Wood Utilization - Federal Research and Product Development Activities, Suuport, and Technology Transfer, in Report to Congressional Requesters. 2006, United States Government Accountability Office.
3.NIMSS, NE506 Wood Utilization Research on US Biofuels, Bioproducts, Hybrid Biomaterials Composites Production, and Traditional Forest Products in Multistate Research Activity Accomplishments Report. 2008.
4.Maranan, M.C. and M.P. Laborie, Analysis of hydrid poplar energy traits by NIR spectroscopy. Journal of Biobased Materials and Bioenergy, 2007. 1(1): p. 155-162.
5.Sattler, C., et al., Effects of hot water extraction of oriented strand board (OSB) wood flakes. Clean, 2008. 36(8): p. 674-681.
6.Parades, J.J., et al., Influence of hot water extraction on physical and mechanical behavior of OSB. Forest Products Journal, 2008. 58(12): p. 56-62.
7.Parades, J.J., et al., Surface characterization of red maple strands after hot water extraction. Wood and Fiber Science, 2009. 41(1): p. 1-13.
8.Fort, R.C.J., et al., Selectivity in the oxidation of carbohydrate-bound lignin. I. Docking of lignin models to the laccase from Trametes versicolor. Proceedings of the 13th ISWFPC, Auckland, NZ, 2005. 3: p. 87.
9.Goodell, B., D. Nicholas, and T. Schultz, Wood Deterioration and Preservation: Advances in Our Changing World. American Chemical Society Series. 2003: Oxford University Press. 465.
10.Kim, T.H. and Y.Y. Lee, Fractionation of corn stover by hor-water and aqueous ammonia treatment. Bioresource Technology, 2006. 97: p. 224-232.
11.Chinn, M.S., S.E. Nokes, and H.J. Strobel, Influence of process conditions on end product formation from Clostridium thermocellum 27405 in solid substrate cultivation on paper pulp sludge. Bioresource Technology, 2007. 98(11): p. 2184-2193.
12.Chinn, M.S., S.E. Nokes, and H.J. Strobel, Influence of moisture content and cultivation duration on Clostridium thermocellum 27405 end-product formation in solid substrate cultivation on Avicel. Bioresource Technology, 2008. 99(7): p. 2664-2671.
13.Packee, E.C., Taxonomy and evolution of Alaska birches, in Agroborealis. 2004, University of Alaska Fairbanks. p. 20.
14.Baxter, P., T.H. Miller, and R. Gupta, Seismic screen, evaluation, rehabilitation and design provisions for wood-framed structures. Practice Periodical on Structural Design and Construction, 2007. 12(4): p. 200-209.
15.Lindt, J.W.v.d., et al., Performance of wood-fram structures during Hurricane Katrina. Journal of Performance of Constructed Facilities, 2007. 21(2): p. 108-116.
16.Dolloff, A., UM blast-resistant wood impresses Army, in Bangor Daily News 02/10/2008. 2008. p. 1.
17.Schirp, A., et al., Biological degradation of wood-plastic composites (WPC) and strategies for improving the resistance of WPC against biological decay. ACS Symposium Series 982 Development of Commercial Wood Preservatives Efficacy, Environmental, and Health Issues, ed. T.P. Schultz, et al. 2008, Washington, DC: American Chemical Society.
18.New WPC technology may boost industry, in Natural & Wood Fiber Composites. 2006, Lou Rossi. p. 7.
19.Chen, J. and D.J. Gardner, Dynamic mechanical properties of extruded nylon-wood composites. Polymer Composites, 2008. 29(4): p. 372-379.
20.O'Neill, S.R.C., D.J. Gardner, and S.M. Shaler, Thermoplastic composites containing lignocellulosic materials and methods of making the same. Patent pending.
21.Duchateau, K., Structural design and performance of composite wall-foundation connector elements, in Civil and Environmental Engineering. 2005, Washington State University.
22.Wang, Y., Morphological characterization of wood plastic composite (WPC) with advanced imaging tools : developing methodologies for reliable phase and internal damage characterization, in Department of wood science and engineering. 2007, Oregon State University: Corvallis.
23.Cheng, Q., S. Wang, and T.G. Rials, Poly vinyl alcohol nanocomposites reinforced with cellulose fibrils isolated by high intensity ultrasonication. Composites Part A, 2009. 40: p. 218-224.
24.Noorani, S., J. Sinonsen, and S. Atre, Nano-enabled microtechnology: polysulfone nanocomposites incorporating cellulose nanocrystals. Cellulose, 2007. 14: p. 557-584.
25.Gardner, D.J., et al., Adhesion and surface issues in cellulose and nanocellulose. Journal of Adhesion Science and Technology, 2008. 22: p. 545-567.
26.Goodell, B., et al., Carbon Nanotubes Produced from Natural Cellulosic Materials. Journal of Nanoscience and Nanotechnology, 2008. 8(5): p. 2472-2474.
27.Tijmensen, M.J.A., et al., Exploration of the possibilities for production of Fischer Tropsch liquids and power via biomass gasification. Biomass and Bioenergy, 2002. 23: p. 129-152.
28.Gadd, G.M., Fungi in Bioremediation. 2001, New York: Cambridge University Press.
29.Messner, K., et al., Overview of white-rot research: Where we are today. Wood Deterioration and Preservaton. 2003. 73-96.
30.Andersen, R., Algal Culturing Techniques. 2005: Elsevier Academic Press.
31.Howell, C., et al., Temporal changes in wood crystalline cellulose during degradation by brown rot fungi. Biodeterioration and Biodegradation, 2009. In press.
32.Kaar, W.E., et al., The complete analysis of wood polysaccharides using HPLC. Journal of Wood Chemistry and Technology, 1991. 11(4): p. 447-463.
33.Howell, C. and J. Jellison, Biological variability in the oxalate/oxalate decarbxylase system among five isolates of the wood-degrading fungus Meruliporia incrassata. IRG/WP 06-10573, 2006.
34.Filley, T.R., et al., Microbial production of phenolic-rich lignin residues in coarse woody debris: A laboratory degradtion of red spruce wood by two common brown rot fungi. Organic Geochemistry, 2002. 33(111-124).
35.Jara, R., et al., Influence of hemicellulose extraction on suitability for oriented strand board (OSB) production - Extraction study, in First conference on biorefineries. 2006: Univ. de Concepcion Chile.
36.Mao, H., et al., Technical economic evaluation of a hardwood biorefinery using the "near-neutral" hemicellulose pre-extraction process. J. Biobased Materials and Bioenergy, 2008. 2(2): p. 177-185.
37.Taylor, A., O. Hosseinaei, and S. Wang, Mold susceptibility of oriented strand board made with extracted flakes. IRG/WP 08-40402, 2008.
38.ASTM standards Related to WOOD FUNDAMENTAL TEST METHODS AND PROPERTIES. ASTM International. West Conshohocken, PA. www.astm.org.
39.ASTM standards Related to WOOD PANEL PRODUCTS. ASTM International. West Conshohocken, PA. www.astm.org.
40.ASTM standards Related to LUMBER AND ENGINEERED WOOD PRODUCTS. ASTM International. West Conshohocken, PA. www.astm.org.
41.ASTM standards Related to WOOD ADHESIVES. ASTM International. West Conshohocken, PA. www.astm.org.
42.Tze, W.T.Y., et al., Evaluation of load transfer in ther cellulosic fiber/polymer interphase using a micro-raman tensile test. Wood and Fiber Science, 2007. 39(1): p. 184-195.