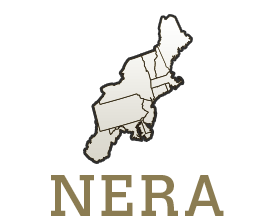
NE1007: Ovarian and Environmental Influences on Embryonic/Fetal Mortality in Ruminants
(Multistate Research Project)
Status: Inactive/Terminating
NE1007: Ovarian and Environmental Influences on Embryonic/Fetal Mortality in Ruminants
Duration: 10/01/2002 to 09/30/2007
Administrative Advisor(s):
NIFA Reps:
Non-Technical Summary
Statement of Issues and Justification
Impaired reproductive performance is one of two major causes of reduced productivity for dairy cattle and represents the major cause of reduced profitability for meat animal species. Despite recent advances in estrous synchronization, overall fertility in dairy herds has declined during the past ten years, both nationally and internationally. Data from the 1996 NAHMS Dairy Study indicate that 11.6% of dairy cows are not pregnant 150 days after calving. The economic impact on dairy profitability is significant. The current NE-161 project has focused on endocrine manipulation of follicular development and luteal function. During the next 5 years, it is proposed to study mechanisms by which nutritional, management and environmental factors impact ovarian activity and the subsequent effect on pregnancy and calving rates.
During the previous NE-161 project period, major progress was made in understanding that the duration of ovarian follicular development preceding ovulation is key to fertility in cattle. Persistent follicles develop under sub-luteal phase progesterone conditions such as during low dosage progestogen treatment for estrous synchronization (Kinder et al. 1996). However, through collaborative efforts of the NE-161 project, it was discovered that follicles that persisted for longer than the length of a normal follicular wave (12 days) resulted in decreased pregnancy rate after ovulation (Cooperative Regional Research Project, NE-161, 1996). The benefits to fertility of having a shorter duration of follicular development were reinforced by further collaborative work. Comparing dairy cows with three waves vs. two waves of follicular development in the cycle prior to ovulation and insemination, the ovulatory follicle was younger and smaller in three wave cows and resulted in a higher pregnancy rate (Cooperative Regional Research Project, NE-161, 2002). Similar differences were also noted in studies with beef cows (Ahmad et al., 1997).
The recognition that a shorter duration of preovulatory follicular development leads to enhanced fertility has made protocols for synchronized breeding more effective. For example, the development of persistent follicles during estrous synchronization can be corrected by recruitment and selection of a new ovulatory follicle by injection of gonadotropin-releasing hormone (Thatcher et al., 1996). This, in turn, has led to development of methods for both synchronization of follicular development and corpus luteum regression to effectively program insemination time (Ovsynch/TAI) without need for estrous detection (Burke et al., 1996; Pursley et al., 1997 a,b; Stevenson et al., 1996). Using this approach and comparing the expected size of the ovulatory follicle with fertility, Vasconcelos et al. (1999) found that pregnancy rates were higher from smaller, younger follicles ie. consistent with results of the previous NE-161 project. Most recently, pre-synchronization of lactating cows with prostaglandin F2a was applied to the Ovsynch/TAI protocol to optimize a short duration of follicular development and increased fertility (Moriera et al., 2001).
Although tools are now available to manage follicular development and allow timed insemination, several field studies have raised concerns about large early pregnancy losses in lactating cows. By using ultrasound imaging of embryos between days 25-32 days after AI, pregnancy losses ranged from 14-40 % before subsequent pregnancy diagnosis at 50-98 days (Vasconcelos et al., 1999; Cartmill et al., 2001; Moreira et al., 2001). Previously Vasconcelos et al. (1997) found that 10.5 % of lactating dairy cows that were pregnant at 28 days had lost the pregnancy by day 42. Based upon return intervals exceeding 27 days after breeding, Thatcher et al. (1994) estimated that late embryonic death rate was 10.6 % in heifers. Attachment of the embryo in the uterus is initiated around day 30 in the cow, with marked development of the placentomes between days 30 and 40 (Melton et al., 1951; King et al., 1982). Earlier authors have illustrated pregnancy losses occurring both before (Van Cleeff et al., 1991) and after day-25 of gestation (Schallenberger et al., 1989; Kastelic et al., 1991; Van Cleeff et al., 1991; Wolff, 1992; Smith and Stevenson, 1995).
As pointed out earlier, fewer data have been collected on embryonic death in beef cows after synchronization of estrus and fixed-time insemination. In the study reported by Bridges et al. (1999) only one of 71 cows pregnant at day 39 failed to calve. Four studies have been done in animals that were inseminated 12 hours after detection of estrus. In Brahman crossbred heifers, fertilization rate was 93% of intact ova, 78% had intact embryos on day 16 and 72% were pregnant on day 35 (Smith et al., 1982). Beal et al. (1992) diagnosed pregnancy by ultrasonography at 25, 45 and 65 days in 205 beef cows that initially had 138 viable embryos. Losses were 6.5% to day 45 and another 1.5% to day 65. Lamb et al. (1997) measured embryo mortality in Bos taurus heifers on three ranches with herds of 169 to 439 head. These heifers had been inseminated 12 hours after they were first detected in estrus in response to an injection of PGF2 17 days after withdrawal of MGA, which had been fed at 0.5 mg/day for 14 days. Conception rates as determined by ultrasonography at 29 to 33 days after insemination ranged from 44 to 67%. Of 525 pregnant heifers, 4.2% did not have viable embryos at palpation 60 to 90 days after the end of the breeding season. Dunne et al. (2000) measured embryo survival at slaughter on day 14 as 68%. By ultrasonography at day 30, their estimate was 76% pregnant, while at full term, 71.8% calved, so that the late embryonic and fetal loss was 4.2 percentage points. Thus they concluded that most losses occurred before day 14 and that losses after day 30 were approximately 5.5%. Clearly late embryonic losses are lower in beef cattle than in dairy cattle.
Drost et al. (1999) have used embryo transfer (ET) to attempt to overcome some of the effects of heat stress in lactating dairy cows in the summer in Florida. Conception rates at day 42 were improved from 21.4% for AI to 35.4% for ET from superovulated donors. Embryo mortality, as estimated from the difference in pregnancies at day 42 and cows with high progesterone on day 22, was 64.7% in cows bred AI and 41.3% in cows given ET.
There is evidence that late embryonic loss precedes luteolysis. In 7 of 8 heifers in which embryonic death was detected between days 25 and 40 post breeding, the onset of luteal regression, as detected by ultrasonography, began at least 3 days after embryonic death, as indicated by loss of heartbeat (Kastelic et al., 1991). In another study utilizing 70 pregnant cows, 7 pregnancies were lost between days 35 and 42 after breeding; embryonic death in each of these 7 cows preceded luteal regression, detected by ultrasonography and declining concentrations of progesterone in milk (Wolff, 1992). Schallenberger et al. (1989) observed an increased secretion of PGF2 between days 30 and 36 in pregnant heifers, one of which lost the pregnancy. However, from the magnitude and timing of secretion of PGF2 , or the luteal response to it, no firm conclusions could be drawn.
Lulai et al. (1994) induced new corpora lutea on day 36 to 40 of pregnancy, during progestogen treatment and after induced regression of the original corpora lutea. When present on the ovary adjacent to the pregnant uterine horn, but not contralateral, induced corpora lutea were maintained after progestogen withdrawal and supported the pregnancy. In research conducted at West Virginia, maintenance of pregnancy was examined after induction of new corpora lutea between days 27 and 54 post breeding (Wright et al. 1994). This was done in cows in which original corpora lutea had either regressed or been removed earlier and pregnancy had been maintained with an exogenous progestogen. After induction of new corpora lutea,, progestogen was withdrawn gradually and pregnancy was maintained only when the new corpus luteum was induced on the ovary adjacent to the embryo.
Bridges et al. (2000) removed original corpora lutea on day 26 of pregnancy, induced new corpora lutea between days 28 and 31 and examined patterns of secretion of PGF2 and estradiol during days 31 through 35. In cows with higher concentrations of PGF2 , more progesterone was secreted by the induced corpus luteum and maintenance of pregnancy tended to be higher. In addition, there was a tendency for more pregnancies to be maintained when concentrations of estradiol were lower.In the pooled data from this study and Wright et al.(1994), when a new corpus luteum was induced on the ipsilateral ovary later than day 36 after mating, 21 of 21 pregnancies were maintained. However, when the corpus luteum was induced on or before day-36 after mating, only 15 of 30 pregnancies were maintained. Further studies of the timing and nature of embryonic deaths after day 25 of pregnancy and the hormonal patterns with which they are associated are needed.
It is disturbing that treatment protocols have been designed to avoid the early embryonic death associated with persistent follicles in the dairy cow, but losses of pregnancy during the late embryonic and early fetal period appear unacceptably high. The degree of late embryonic loss varies widely among different studies and makes a large contribution to the overall low pregnancy rate to AI in dairy cattle that presently stands at less than 40 % ( Butler, 1998). These late embryo losses may be attributable to alteration in corpus luteum function at any point between maternal recognition of pregnancy through embryo attachment and further compromised by other factors such as heat stress (Cartmill et al., 2001).
Advantages of performing this work as a multi-state effort include the ability to commit larger numbers of animals under similar, but different management systems to the research effort, overlapping approaches with collaborative efforts and technologies can be directed toward several objectives simultaneously, and shared experience and data analysis make the interactions more beneficial.
Related, Current and Previous Work
Luteal function and embryo/fetal survival
Recent work at the West Virginia Station showed that pregnancy loss prior to day 45 was greater (p<0.01) in cows with low serum concentrations of progesterone at 28 to 37 days of gestation (p=0.01). This led to the conclusion that embryonic mortality after maternal recognition of pregnancy is associated with the level of function of the corpus luteum during day 28 to 37. The regulation of progesterone (P4) production by the corpus Luteum (CL) is complex (Hansel et al., 1973; Niswender and Nett, 1994). Presently, there is evidence that substances such as matrix metalloproteinases (MMPs) and tissue inhibitors of metalloproteinases (TIMPs), which govern remodeling of the extracellular matrix (ECM), may also play important roles in the regulation of steroidogenesis.
The ECM is implicated in modulating a variety of cellular processes, including differentiation and apoptosis (Smith et al., 1999; Hulboy et al., 1997). In turn, the ECM is acted upon by a large family of proteolytic enzymes known as MMPs (Birkedal-Hansen et al., 1993; Woessner, 1998). Among the MMPs, most notable are the gelatinases, MMP-2 and MMP-9, which degrade type IV collagen, a constituent of luteal tissue (Silvester and Luck, 1999). Because of the ability of MMP family members to degrade all components of the ECM, their activities are tightly monitored by the TIMPs, especially TIMP-1 and TIMP-2 (Salamonsen, 1996). Thus, the integrity of the ECM depends on the balance between MMPs and TIMPs.
The network of ECM proteins is connected to the cytoskeleton by integrins (Calderwood et al., 2000; Choquet et al., 1997). Disruption of the ECM-integrin may impact cytoskeletal stability, leading to altered cellular function. Indeed, in sheep CL following a one hour-long systemic infusion of PGF2 , luteal TIMP proteins decrease dramatically at 1 hour, followed by an increase in MMP-2 activity, which coincided with the nadir in plasma P4 at 8 hours (Towle et al., 2000). Others have noted the disintegration of the ovine luteal cytoskeleton after PGF2 treatment (Murdoch, 1996). Thus, it appears that perturbations of MMP/TIMP relationships may lead to alterations in the ECM, affecting the stability of the cytoskeleton, which is critical for cellular steroidogenesis (Niswender et al., 2000).
There are also more direct relationships between MMPs/TIMPs and steroidogenesis. The steroid acute regulatory protein, StAR, and TIMP-1 share a 124-base pair nucleotide homology (Hartung et al., 1995). In addition, TIMP-1 concentrations are correlated with P4 concentrations in follicular fluid, and this corresponds to the ability of TIMP-1 to stimulate steroid production (Boujrad et al., 1995). In contrast, the activities of MMP-2 and MMP-9 are inhibited by P4 (Marbaix et al., 1992).
Relationship between immune cells and the corpus luteum:
Regression of the corpus luteum is associated with the dramatic accumulation of immune cells, especially monocytes and macrophages (Townson et al., 1996; Bowen et al., 1996; Naftalin et al. 1997; Penny et al., 1999; Lawler et al. 1999; Olson and Townson, 2000; Townson et al., 2002). Immune cells secrete cytokines such as tumor necrosis factor (a cytokine of macrophage origin) and interferon- (a cytokine of T-lymphocyte origin) that together inhibit progesterone production and are extremely toxic to luteal cells (Benyo and Pate, 1992). Interestingly, expression of tumor necrosis factor increases within the bovine corpus luteum around the time of luteolysis (Shaw and Britt, 1995). Additional effects of cytokines derived from immune cells include potent stimulation of luteal prostaglandin production (Nothnick and Pate, 1990; Fairchild and Pate, 1991; Benyo and Pate, 1992; Townson and Pate, 1994, 1996; Sakumoto et al., 2000), induction of apoptosis (Rueda et al., 1997; Friedman et al., 2000), and the induction of major histocompatibility complex (MHC) class II molecules on luteal cells (Benyo et al., 1991). Increased expression of MHC class II molecules is particularly significant. It suggests that there are interactions of steroidogenic cells with cells of the immune system (Benyo et al., 1991; Fairchild and Pate, 1991; Petroff et al., 1997), which may influence luteal function. More recently, it has been speculated that immune cells within the bovine corpus luteum may contribute to toxic effects that result in early embryonic loss (Buford et al., 1996). However, an underlying assumption in these studies is the idea that immune cell recruitment in the bovine corpus luteum occurs only during the latter stages of the estrous cycle to facilitate luteolysis. This assumption is based upon relatively few studies, of limited scope, in which populations of immune cells within the bovine corpus luteum were characterized (Lobel and Levy, 1968; Penny et al., 1999). In particular, there have been no comparative studies that directly assess the extent of immune cell recruitment and relative numbers of immune cell populations within the corpus luteum of non-pregnant and pregnant cows. Such studies are of fundamental importance, and would provide insight concerning the potential influence of inflammatory cells on luteal function and the maintenance of pregnancy.
Immune cell recruitment within the corpus luteum:
Although the physiological role of immune cells in the corpus luteum is not clearly understood, the striking accumulation of these cells within the corpus luteum at the time of luteal regression raises a fundamental question concerning the mechanisms that are responsible for such recruitment. Murdoch (1987) was among the first to suggest that a chemoattractant might be responsible for the recruitment of immune cells into the corpus luteum of ruminants. More recently, with the characterization of a large superfamily of structurally and functionally related chemokines (Oppenheim et al., 1991; Miller and Krangel, 1992), at least one chemokine, monocyte chemoattractant protein-1 (MCP-1), is found to be expressed in the corpus luteum and is associated with the accumulation of immune cells during luteal regression (Townson et al., 1996; Bowen et al., 1996; Tsai et al., 1997; Haworth et al., 1998; Penny et al., 1998; Townson et al., 2002). In the cow, MCP-1 mRNA following prostaglandin F2 -induced luteolysis (Tsai et al., 1997; Penny et al.,1998) and general characterizations of immune cell populations within the CL have been described (Lobel and Levy, 1968; Penny et al., 1999). More recently, it has been determined that there is increased expression of MCP-1 mRNA and protein in the bovine corpus luteum by Day 12 postovulation, several days prior to the onset of luteal regression (Townson et al., 2002). Furthermore, this increase in MCP-1 expression is accompanied by a significant accumulation of immune cells in the corpus luteum at mid-cycle. These observations raise a provocative question of whether or not the role of immune cells within the corpus luteum extends beyond merely eliminating cells and cellular debris during luteolysis.
Effects of environment, metabolism and health on oocyte maturation, fertilization and embryonic/fetal survival in dairy cattle:
Worldwide, heat stress is a major cause of low fertility, with pregnancy rates decreasing from 40-60% in the winter to 10-20% in the summer in tropical and subtropical areas of the world (reviewed in Wolfenson et al., 2000). Even when cooling systems are used, the fertility of dairy cows is lower in the summer (Hansen, 1997). Lactating dairy cattle are more susceptible to the effects of heat stress than are beef breeds because of their high internal heat production. Even in temperate climates dairy cows can be affected by hyperthermia, since the critical temperatures are as low as 25-27 C (Hansen, 1997). The increase in milk yield in recent years has compounded the effects of heat stress on fertility since it is more difficult for high yielding cows to maintain normothermia due to their higher metabolic heat production. It is difficult to determine how hyperthermia affects reproductive function since it could potentially affect every organ and system in the body. Changes such as redistribution of blood flow among body organs, respiratory alkalosis, and alterations in food intake are all general responses to heat stress that could indirectly affect reproductive function. Within the reproductive system heat stress could affect oocyte quality, uterine function, or ovarian function.
Diminished folliculogenesis (Badinga et al., 1993; Wolfenson et al., 1995; Wilson et al., 1998), reduced conception rates (Badinga et al., 1985; Wolfenson et al., 1988), altered luteal function (Abilay et al., 1975; Vaught et al., 1977; Rosenberg et al., 1982), and embryonic loss (Biggers et al., 1987) have all been reported in cows following periods of heat stress. A direct effect of hyperthermia on the hypothalamo-pituitary-ovarian axis was suggested as a causative factor of infertility (Flowers and Day, 1990). At the current time there is only very limited information available on the characteristics of bovine follicular cells in vitro after exposure to either heat stress in vivo or to heat stress in vitro (Wolfenson et al., 1997) and there is no information about the effects of heat stress on the responses of follicular, theca and granulosa cells to the pituitary gonadotropins, LH and FSH. Similarly, there is little information on the effects of heat stress on steroidogenesis by the corpus luteum. There is inconsistency as to whether heat stress increases (Abilay et al., 1975; Roman-Ponce et al., 1981), decreases (Rosenberg et al., 1982; Wise et al., 1988b), or does not alter (Biggers et al., 1987; Wise et al., 1988a) luteal progesterone production. In addition, there is only limited knowledge of the direct effects of heat stress on luteal function (Khann et al., 1994). In the current study, experiments have been designed to determine the extent to which heat stress affects follicular and luteal steroidogenesis. Specifically, we will test the in vitro effects of heat stress on production of steroids by ovarian follicles, the responses of follicular cells to gonadotropins, steroidogenic enzymes within luteal cells, and the capacity of luteal cells to respond to gonadotropin/lipoprotein stimulation.
Increased genetic capability for milk production coupled with changes in nutritional management and larger herd size has been associated with a decline in fertility of lactating cows (Butler, 1998; Darwash et al., 1999). Nutritional requirements shift abruptly after parturition as milk production rapidly increases and the ensuing negative energy balance (NEBAL) results in mobilization of body fat with accumulation of triglycerides in the liver (Rukkwamsuk et al., 1999), a longer interval to first ovulation, and reduced fertility (Butler and Smith, 1989; Jorritsma et al., 2000).
One important link between NEBAL and lower fertility is through timing of first postpartum ovulation; the early commencement of ovulatory cycles is associated with improved conception rate (Butler, 2001; Darwash et al., 2001). NEBAL alters follicular development and delays first ovulation in postpartum dairy cows (Beam and Butler, 1999). Low energy availability during NEBAL not only suppresses pulsatile LH secretion, but also reduces ovarian responsiveness to LH stimulation (Butler, 2001) via low plasma glucose and insulin. Plasma concentrations of insulin-like growth factor-I (IGF-I) are also directly related to energy status and ovarian follicular development (Beam and Butler, 1999; Webb et al., 1999). The importance of insulin and IGF-I for regulating postpartum ovarian follicular development demonstrates that the functional capacity of the somatotropic axis is intertwined with ovarian activity during NEBAL. Low IGF-I is a consequence of simultaneous hypoinsulinemia and reduced expression of hepatic GH receptor (GHR-1A; Kobayashi et al., 1999), because insulin treatment can increase circulating IGF-I through increased hepatic expression of mRNA for IGF-I and GHR-1A (Butler and Butler, 2001).
While accepting the general benefits on fertility of an early postpartum return to ovarian cycles, we should be mindful of the interaction with uterine health. Multiparous cows ovulating before 21 days postpartum exhibited poorer reproductive performance than those ovulating later in association with a high incidence of persistent corpora lutea (Smith and Wallace, 1998) resulting from uterine infection (Opsomer et al., 2000). Evidence of uterine infection and endometritis are clearly associated with reduced fertility to insemination (Loeffler et al., 1999; Jorritsma et al, 2000; Mahmoudzadeh et al., 2001) indicating that health problems must be considered part of the overall trend for lower fertility in high yielding cows.
Another important link between NEBAL and fertility is the carryover effect for lower blood progesterone. Plasma progesterone concentrations in cows selected for high milk yield were 25 to 50% lower during the second and third luteal phase than in control line cows (Lucy and Crooker, 2001). Optimizing progesterone concentrations is important since plasma progesterone was higher by 4-7 days after AI in cows that became pregnant than in non-pregnant cows (Cooperative Regional Research project, NE-161, 1996). Progesterone stimulation by day 5 advances development of the conceptus (Garrett et al., 1988), which by day 16 must produce sufficient quantities of interferon tau to inhibit the uterine luteolytic mechanism (Mann and Lamming, 2001).
The "memory" by which early NEBAL translates into reduced progesterone production two months later was hypothesized by Britt (1992) to represent detrimental effects on ovarian follicles during their early growth and development leading to lower progesterone secretion after ovulation. Indeed, a clear demonstration of prolonged detrimental carryover effects on the bovine follicle was recently reported showing that an acute heat stress affected follicular steroidogenesis 24 days later (Roth et al., 2001). Fertility in dairy cows reflects the cumulative influence of metabolic, endocrine, and postpartum health components that have been modified and exaggerated by selection for high milk yield. Energy balance seems to be the most important factor related to poor embryo survival, but the complex interactions of all these factors must be considered if we are to develop new strategies to improve fertility.
Wrenzycki, et al., (2000) have also shown that diet type has an effect on embryo metabolism. Rations supplemented with either a starch based concentrate, barley, which favors the formation of proprionate, or a high fiber concentrate (citrus pulp), which favors production of acetate, were compared for their effect on embryo metabolism. Embryos were recovered from superovulated beef heifers on days 6, 7 or 8 (morula through expanded blastocyts) and metabolism of pyruvate assessed utilizing the hanging drop method developed by Reiger, et al.(1992). Embryos recovered from heifers fed the starch based, gluconeogenic diet, metabolized significantly more pyruvate than those recovered from the heifers fed the fiber based ration and increased the relative abundance of Cu/Zn superoxide dismutase in day 8 embryos. The inverse relationship between these two parameters suggests that the embryos from starch fed heifers were under metabolic stress. This could be related to the observation that high levels of glucose inhibit embryo development in vitro. However, it is not known if the effects observed are due to changes in the fluid milieu of the reproductive tract or possible long term effects of the rations on blood parameters that may impact the developing oocyte during follicular maturation.
Objectives
-
Examine events surrounding follicular development and the follicular to luteal transition that may affect embryonic/fetal mortality.
-
Examine mechanisms involved in insufficient or altered luteal function that may result in embryonic/fetal mortality.
-
Determine environmental and metabolic effects on oocyte maturation, fertilization and embryonic/fetal survival.
-
Methods
1. Examine events surrounding follicular development and the follicular to luteal transition that may affect embryonic/fetal mortality. Relationship of follicle size and preovulatory estradiol to embryo/fetal survival Lactating dairy cows at the New York Station (80 total; 40/season; 60-120 days lactation) will be treated during either July-September or January-March to synchronize ovulation and artificial insemination (OvSynch/TAI). The size of the largest ovarian follicle (presumptive ovulatory follicle) will be measured on the day of the second GNRH injection by ultrasonographic (US) exam of the ovaries. Blood samples will be collected daily from the US exam (d -1) until day +7 and then on days 14, 21, 28, 35 and 60. US examination for the detection of an embryo or fetus will be conducted on days 30 and 60 post-AI. Within each season, follicle size (< vs. > group mean) will be compared to peripheral concentrations of estradiol and pregnancy status on days 30 and 60. Factors involved in follicular development: Macrophage migration inhibitory factor (MIF) is a protein that regulates growth and proliferation of a variety of tissues. Large, steroidogenic luteal cells contain MIF, and the mRNA for MIF is highest in the developing CL. Granulosal, but not thecal, cells of preantral and antral follicles also contain MIF. The stage of follicular development in which MIF first appears is not known. This experiment will test the hypothesis that MIF is expressed in granulosal cells of primary, but not primordial follicles. Ovaries will be collected from lambs on days 7, 30, 150 and 240 days of age, fixed in Prefer fixative and processed for immunohistochemistry. Anti-bovine MIF antibody will be used to localize MIF within the ovary, as described by Bove et al. (2000). This antibody has been validated for use in sheep tissues. Follicles will be classified according to McNatty et al. (1999), and staining intensity will be recorded for follicles within each class by two independent observers. Types of follicles that contain MIF and cellular localization of MIF will be recorded. 2. Examine mechanisms involved in insufficient or altered luteal function that may result in embryonic/fetal mortality Timing of embryonic mortality and its relationship to progesterone in dairy cattle. a. Preliminary work: Patterns of progesterone were studied as predictors of pregnancy maintenance in dairy cows (N = 211) on 2 farms. Cows were bred either at spontaneous or synchronized estrus, by artificial insemination or natural service. Beginning on d 28 to 36 of gestation, cows were examined by ultrasonography for the presence of a viable embryo with a heartbeat and a blood sample was collected. Pregnant cows were examined again between d 45 to 51 and d 59 to 66 (Farm 1) or 3 times per week until d 60 (Farm 2) for presence of a viable embryo. Overall rate of embryonic mortality was 10.9% (12.7% for Farm 1 and 7.2% for Farm 2). Timing of embryo loss was classified as late embryonic (Measurement of Progress and Results
Outputs
- Research data on the effect of the follicle on subsequent embryonic/fetal survival in published form
- Research data on alterations in luteal function that result in embryonic/fetal mortality in published form
- Research data on environmental and metabolic effects on embryonic/fetal survival in published form
- Information that will help producers make informed management decisions to enhance embryonic/fetal survival in ruminants
- Present research results at professional meetings for animal scientists, veterinarians and other agricultural related professionals.
Outcomes or Projected Impacts
- Development of herd management strategies to minimize the effects of nutritional and heat stress on herd fertility.
- Further refine current estrous synchronization protocols to enhance embryo survival.