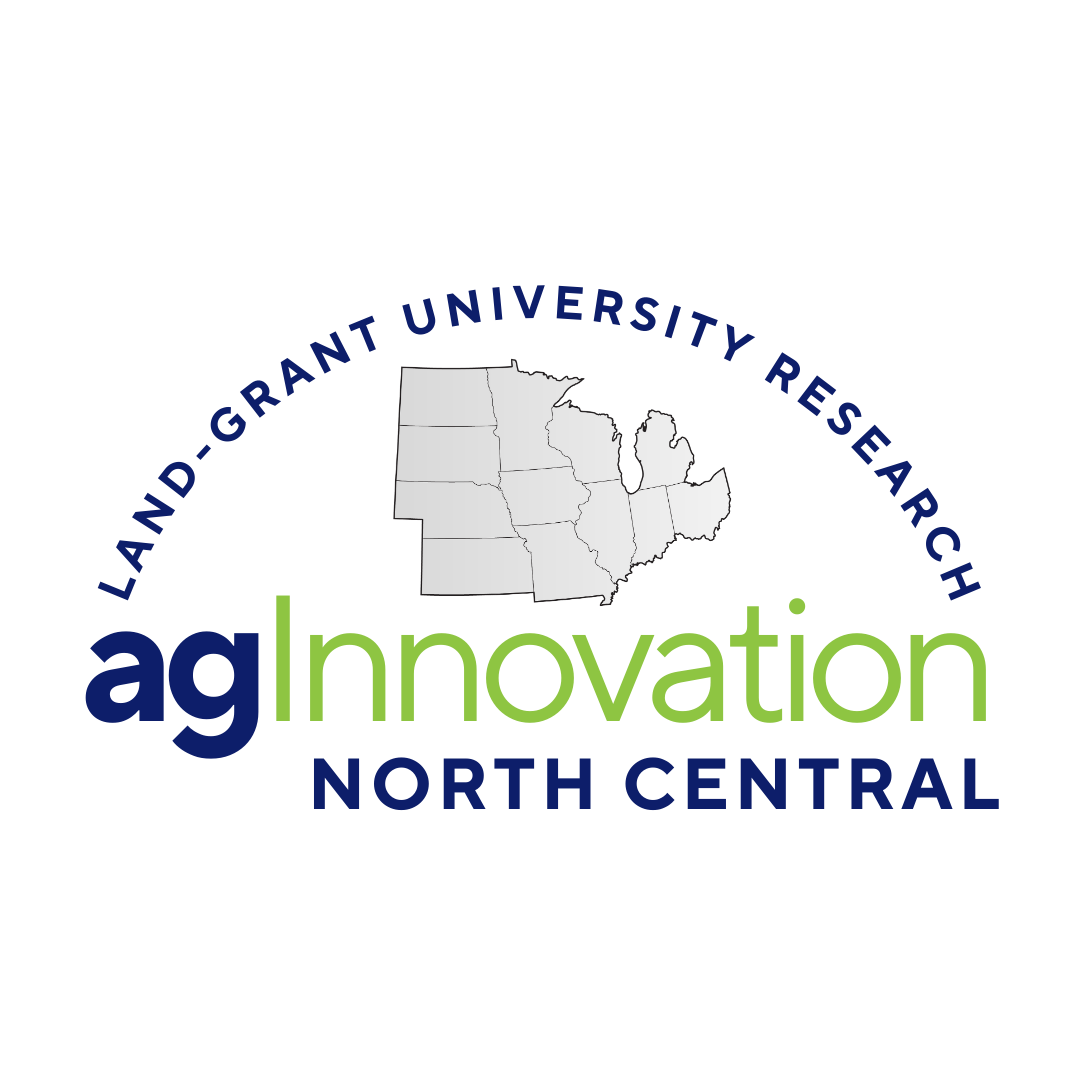
NC_OLD1180: Control of Emerging and Re-emerging Poultry Respiratory Diseases in the United States
(Multistate Research Project)
Status: Inactive/Terminating
NC_OLD1180: Control of Emerging and Re-emerging Poultry Respiratory Diseases in the United States
Duration: 10/01/2009 to 09/30/2014
Administrative Advisor(s):
NIFA Reps:
Non-Technical Summary
Statement of Issues and Justification
THE NEED AS INDICATED BY STAKEHOLDERS. Poultry meat production has been continually increasing and consumption has surpassed all other meats. Because of the efficiency and competitive nature of the poultry industry, the cost of poultry meat is very attractive to consumers. Furthermore, the National Chicken Council released results of a survey showing that chicken was preferred to beef and pork, in terms of versatility, taste, ease of preparation, being healthful and nutritious, price and consistency of quality. In 2007, per-capita annual consumption of chicken was 90.6 pounds, the highest of any of the major meats. In addition, annual per capita turkey meat consumption was approximately 18 pounds. Beef consumption was 69 pounds per person on a retail weight basis, while pork was 52 pounds per person. A survey conducted by Bruskin Research found that 89% of the respondents ate chicken at least once per week and 36% of the respondents consumed chicken three times per week or more. The poultry industry is a highly integrated growth industry and is a major contributor to animal agriculture. The combined value of production from broilers, eggs, turkeys, and the value of sales from chickens in 2007 was $31.9 billion, up 24 percent from the $25.8 billion in 2006. Of the combined total, 67 percent was from broilers, 21 percent from eggs, 12 percent from turkeys, and less than 1 percent from chickens. In 2007, broiler production was valued at $21.5 billion up 21 percent from 2006. The number of broilers produced was 8.9 billion, slightly up from 2006. The value of turkeys produced during 2007 was $3.71 billion, up 4 percent from the $3.57 billion the previous year. Turkey production in 2007 totaled 7.87 billion pounds, up 5 percent from the 7.46 billion pounds produced in 2006. Chicken eggs are an important source of high quality protein and other nutrients in the diet.
IMPORTANCE OF THE WORK, AND WHAT THE CONSEQUENCES ARE IF IT IS NOT DONE. Respiratory diseases are the major group of diseases affecting poultry. Consequently, losses induced by these diseases are of major economic impact on the producer, the local economy and the U.S. economy. Many endemic respiratory infectious diseases in the U.S. continue to decrease the profitability of commercial poultry production. Viruses, bacteria and fungi cause respiratory diseases or interact to cause disease. Management of poultry is also a critical factor in controlling respiratory disease. One of the diseases, avian influenza (AI), has significant potential to impact the industry both in terms of animal losses and through export restrictions. AIV which is endemic in live poultry markets in the Eastern and Western U.S. will remain a significant threat to commercial poultry producers. A major AI outbreak occurred in commercial poultry in Virginia in 2002 resulting in $130 million in losses. Outbreaks in smaller commercial poultry populations occurred in Pennsylvania, Connecticut, Rhode Island, Minnesota, California, Delaware and Maryland.
The U.S. poultry industry is the world's largest producer and second largest exporter of poultry meat. Approximately 16% of the total production in the U.S. is exported (Economic Research Service, USDA; http://www.ers.usda.gov/Briefing/Poultry/). These export markets are subject to immediate restriction when poultry respiratory diseases such as AI or exotic Newcastle disease (END) are diagnosed and reported. Since our last project was initiated, important new threats to poultry production have become a concern of the industry and require a focus of our future project. A major epidemic of END occurred in California from September 2002-May 2003. Eradication of END cost more than $162 million, a value that does not include lost export trade at the time. Smaller outbreaks in non-commercial poultry occurred in Arizona and Nevada. An apparently unrelated END outbreak in backyard poultry also took place in Texas.
The agrobioterrorism threat to the U.S. food supply is a post 9/11 2001 reality. AIV and END are classified as agrobioterrorism agents. Our project will develop strategies for rapid diagnosis and control of these diseases.
Endemic respiratory diseases of poultry continue to collectively cost poultry producers millions of dollars annually. These include infectious laryngotracheitis (ILT), variant infectious bronchitis (IB), lentogenic ND, and infections caused by E. coli, avian mycoplasmas, Ornithobacterium rhinotracheale and P. multocida. Losses are realized from mortality and morbidity via condemnation at processing and poor performance (increased feed conversion and medication costs, decreased growth).
Considering the challenges outlined above, it is essential that the proposed studies proceed to help protect the nation's food supply and the economic well-being of farmers and the poultry industry.
THE TECHNICAL FEASIBILITY OF THE RESEARCH. The technical challenges posed by the goal of improved respiratory disease control are significant but approachable. Participants in the project have experience and training to undertake the work and complete the objectives. In addition, physical facilities, equipment, and other resources are committed by member institutions to guarantee the success of the proposed activities.
THE ADVANTAGES FOR DOING THE WORK AS A MULTISTATE EFFORT. Control of respiratory infectious diseases lends itself to collaborative multistate research. The diseases are endemic in many poultry producing states. Furthermore the challenges posed by the number of different disease etiologies and their complexities require a multistate effort.
NC1019 SIGNIFICANT FINDINGS AND PRODUCTIVITY. The understanding of respiratory diseases of poultry has been advanced considerably through the current NC1019 project which merged in 2004 with the now defunct NE-228 project, Epidemiology and Control of Emerging Strains of Poultry Disease Respiratory Agents. Of notable mention are the advances made in rapid diagnostics for avian influenza and Newcastle disease viruses and genotype identification of infectious bronchitis virus and infectious bursal disease virus. DNA and recombinant vector based vaccine technologies have also moved forward at a dramatic pace and hold much promise for improved control of respiratory diseases. Studies with the newly recognized diseases such as turkey rhinotracheitis and chicken swollen head syndrome caused by avian metapneumovirus have led to better understanding of the disease, development of diagnostic tools, and development of an experimental vaccine that has been used successfully to control the disease. The combination of these and other findings have led to very productive years of the current project as indicated by the number of papers published or in press of which many include joint publications. Please refer to the Composite Annual Station Reports attached to this proposal for more information on findings and publications for the years 2004-2008.
WHAT THE LIKELY IMPACTS WILL BE FROM SUCCESSFULLY COMPLETING THE WORK. The overall impact of a successful outcome will be improved diagnosis and control of respiratory diseases that will benefit the poultry industry. Impact of the research will be derived from identification of disease agent reservoirs such as wild birds, factors involved in agent transmission to poultry, the development and delivery of differential immuno and gene based diagnostics, determination of infection status, rapid strain identification, evaluation of vaccines, and the design and implementation of eradication protocols for selected agents. The overall outcome of the project is to produce findings that enable poultry industry to remain competitive and profitable.
The specific NCRA Crosscutting Research Areas and Priority Research Objectives addressed directly or indirectly by this NC1019 research proposal are:
i. Agricultural Production, Processing and Distribution. Objective 1. Develop alternative agricultural production systems to enhance economic competitiveness in the rural landscape; and Objective 2. Develop improved animal, plant and microbial production, processing and marketing systems that are competitive, profitable and environmentally sound over the long term.
ii. Genetic Resources Development and Manipulation (Genomics and Germplasm). Objective 1. Develop new genotypes that increase product value, enhance global competitiveness, improve human nutrition, nurture environmental quality and foster rural development.; Objective 2. Broaden and enrich the knowledge base about genomics; Objective 3. Collect, preserve, share, enhance and evaluate germplasm at the molecular, cellular and/or organismal levels; and Objective 5. Develop increased knowledge of the interactions and interrelationships of the various life forms.
iv. Natural Resources and the Environment. Objective 7. Develop remediation systems to reduce agricultural, non-agricultural, and chemical waste contamination of soil, water and air; and Objective 10. Document the link between animal welfare/behavior, care and management and their environment.
v. Economic Development and Policy. Objective 1. Develop profitable technologies and systems. Determine the potential profitability of production, processing and distribution technologies (innovations, i.e., agricultural information, technology, precision agriculture) that are environmentally sound and socially acceptable; Objective 2. Enhance U.S. global competitiveness. Enhance international market development by analyzing factors including the increasing adoption of agricultural biotechnology that determine U.S. competitiveness in global markets and analyze alternative policies to modify these factors to the advantage of U.S. agriculture; and Objective 3. Create new management decision-making models. Design optimal management systems for cropping systems, forest systems, non-cropped ecosystems, animal systems, whole farm and watershed systems, fishery and wildlife and data needs of agricultural businesses, research organizations and consumer groups.
The specific CREES/GPRA Goals and Strategic Objectives addressed directly or indirectly by this NC1019 research proposal are:
I. An Agricultural System That is Highly Competitive in the Global Economy. Objective1. Facilitate informed decisions by agricultural producers, policy officials, and other decision makers by developing and sharing knowledge promoting agricultural production and marketing; Objective 2. Expand the knowledge base leading to improvements in productivity and marketability, development of new and enhanced commercial products, and expansion of foreign and domestic market opportunities. Objective 3. Ensure the long-term economic viability and sustainability of production agriculture as it makes the transition from Federal subsidies to world market orientation; and Objective 4. Strengthen and coordinate the capabilities of the REE agencies to enable joint action and rapid response to emerging issues and problems in a global context.
II. A Safe and Secure Food and Fiber System. Objective 1. Reduce the impact of threats to agricultural production by expanding the knowledge base needed to rapidly and effectively manage pests, disease, and natural disasters.
IV. Greater Harmony Between Agriculture and the Environment. Objective 1. Promote sustainable agricultural production and enhance environmental quality by enabling producers to use cost-effective, environmentally friendly production practices and systems.
V. Enhanced Economic Opportunity and Quality of Life for Citizens and Communities. Objective 1. Promote the effectiveness of rural policies and programs by (a) enhancing understanding of the conditions that promote economic opportunities, and (b) identify rural needs.; and Objective 2. Promote new businesses and growth in existing businesses, including farms and ranches, by transferring knowledge and technologies developed by or in partnership with REE agencies to private sector entrepreneurs.
Related, Current and Previous Work
AVIAN INFLUENZA VIRUS (AIV). Avian Influenza (AI) is an economically important disease of domestic poultry and has caused considerable economic losses in many countries including the U.S. Migratory aquatic birds are the natural reservoir of influenza A viruses (Pereda et al., 2008). Some strains adapt to poultry and other mammals including humans and cause severe disease. The U.S. government spent $60 million in 1983-84 to eradicate a highly pathogenic (HP) H5N2 virus in poultry in Pennsylvania (Lasley, 1986). In 2004, a HPAI outbreak occurred in Texas, making it the first HPAI to be reported in the U.S. since 1983-84 (Lee et al., 2005). There have also been several costly outbreaks of low pathogenic (LP) AI affecting poultry flocks in 1988, 1991, and 1995. AIV infected turkeys in Minnesota from 2000-2002 (Halvorson, 2002) and flocks in California (Webby et al., 2003). From 1994-present, LPAIV has regularly been found in live bird markets (LBMs) in the northeastern U.S. (Spackman et al., 2003). LBM LP AIVs were the source of outbreaks in commercial poultry in Virginia in 2002 and Delmarva in 2004. In 1997-1998, a novel H3N2 triple reassortant (TR) virus containing gene segments from human, avian and swine origins emerged in the U.S. swine. The TR swine H3N2 virus crossed the species barrier to turkeys and since 2003, has caused disease in turkeys in North America (Tang et al., 2005; Yassine et al., 2008).
Humans are highly susceptible to type A influenza viruses. Four pandemics occurred in the 20th century (Li et al., 2008; Solórzano et al., 2007). The most devastating pandemic was the 1918 "Spanish flu" that killed 20-40 million people. The emergence of the next influenza pandemic is considered imminent. Unfortunately, little is known about the molecular features that enable influenza A virus to cause pandemic disease, although multiple genes, encoding both the surface and internal proteins of the virus, are thought to be involved. Avian and mammalian (especially swine) species are reservoirs of influenza viruses, which contribute genes in the evolution of pandemic strains (Horimoto & Kawaoka, 2005). Since 1997, cases of human infections with different AIV subtypes (H5N1, H7N7, H9N2) have been identified and raised the pandemic potential for direct transmission of AIV to humans (Fouchier et al., 2004; Kermode-Scott, 2004; Peiris et al., 1999; Subbarao et al., 1998). As the threat of a pandemic due to highly pathogenic H5N1 and other subtypes of AIV looms, a better understanding of the epidemiology among different species and biological and genetic basis of transmissibility of animal origin influenza viruses among humans is necessary. We have recently identified a triple reassortant H3N2 virus that can efficiently transmit between turkeys and swine and the finding makes these species an excellent model for studying interspecies transmission between avian and mammalian species (Yassine et al., 2007).
Several poultry producing regions in the U.S. are located in close proximity to aquatic bird refuges. The Delmarva region has a thriving commercial broiler chicken industry producing more than 560 million birds annually. Moreover, the Delmarva peninsula is a key stop-over for migratory birds in the Atlantic Flyway during the spring and fall (Wander & Dunne, 1981). The Delaware Bay area is host to as many as half a million shorebirds, including red knots and ruddy turnstones that carry AIV. These species time their northern migration with the arrival of the largest concentration of spawning horseshoe crabs along the Atlantic coast (Botton et al., 1994). In addition, southern migrating juvenile waterfowl, many carrying LPAIV (Webster et al., 1992) also stop-over on the Delmarva. Some wild bird origin LPAIV are capable of infecting commercial poultry (Landolt & Olsen, 2007) and it is important to evaluate the potential pathogenic of these viruses in poultry. Waterfowl origin H5N1, H7N3 and H6N2 LPAIVs and a shorebird H3N6 virus were evaluated in commercial chickens and turkeys (Ladman et al., 2008). LP H7N3, H6N2 and H3N6 subtype outbreaks have been reported in turkeys or chickens (Hirst et al., 2004; Jones & Swayne, 2004; Webby et al., 2003; Song et al., 2008).
Recent outbreaks of AI in different species including humans have highlighted the necessity to improve existing diagnostic tests and to develop new more rapid and specific methods to detect future outbreaks. With conventional methods of virus isolation and serologic testing (Swayne et al., 1998), the laboratory confirmation of AI takes a relatively long time because of the lack of capacity for providing high throughput, sensitive and specific assays. In the last decade, new-generation assays based on molecular techniques have become available and applied successfully to disease diagnostics and active surveillance programs (Brown, 2006). The majority of the new techniques are based on the amplification of specific nucleic acid sequences by polymerase chain reaction (PCR), or other similar methods (NASBA) (Lau et al., 2006). Real-time RT-PCR is widely used because it offers high specificity and sensitivity. However, the main disadvantage is the cost and the lack of multiplexing capability. To overcome the limit of multiplexing, microarray technology is being developed rapidly and has been applied to diagnosis of influenza A virus (Quan et al., 2007). However, due to its solid phase format in nature, cost and flexibility have been an issue for practical application. The microsphere-based assay is an emerging technology capable of multiplexing up to 100 different assays from a single sample (Mandy et al., 2001; Mariella, 2002). Considering the flexibility, ability to multiplex, and automated high throughput capabilities at an affordable price, microsphere-based assay has great potential in rapid AIV diagnostics.
The most commonly used AI vaccines are produced from chick embryo-propagated virus (Lee and Suarez, 2005). Live virus is formalin or beta propiolactone inactivated for use in poultry, mixed with an adjuvant, usually an oil-based adjuvant. Inactivated vaccines are often prepared from a stock virus of a given subtype or an autogenous strain. Inactivated vaccines, if properly prepared and administered, provide high levels of neutralizing antibody and protection from clinical disease. However, poor standardization of commercial vaccines can affect the immune response (Garcia et al., 1998; Swayne et al., 1999). A recombinant fowlpox virus (rFPV) vaccine expressing the H5 from A/Turkey/Ireland/83, developed in the 1980s, induced protection against lethal challenge with H5 influenza viruses (Bublot et al, 2006). The rFPV vaccine was commercialized and eventually approved for use by several countries as one of the first viral-vectored vaccines. Advantages of the rFPV vaccine over inactivated AI vaccines are; 1) single administration at 1 day of age and early onset (1 week) of protection, 2) easy monitoring or differentiation of infected from vaccinated animals. Despite this potential, most of the protection studies with rFPV vaccine have used 1-day-old chickens, and further studies should be conducted in various poultry of different ages.
Experimental recombinant vaccines for AIV are currently being developed to improve and overcome the drawbacks of current vaccines. Live viral vectors have been engineered to express either the H5 or H7 antigens (Luschow et al., 2001; Schlesinger and Dubensky, 1999; Schultz-Cherry et al., 2000; Toro et al., 2008). Using a recently developed plasmid-based reverse genetics system for influenza, viruses can be rescued from cloned cDNA for the purposes of producing reassortant influenza viruses (Neumann et al., 1999). A reverse genetics technique was used for the rapid generation of reassortant viruses that may serve as candidate vaccine strains for AI (Liu et al., 2003; Lee et al., 2004b). In addition, live-virus vaccines were developed for AI (Song et al., 2007; Wang et al., 2008). Live-virus vaccines are advantageous since they induce mucosal immune responses and cell-mediated immunity, which may give wider cross-protection and longer-lasting immunity compared to inactivated vaccines. In broilers, vaccines are often administered during later stages of embryonation, usually at 17 18 days of incubation (Ricks et al., 1999). In ovo vaccine delivery is cost-effective and has largely replaced post-hatch injection of Marek s disease vaccine in broilers in the U.S. (Kreager, 1998). Replication-defective live influenza virus that induces strong immune responses, but does no generate infectious virus in hatched chicks warrants investigation.
A plasmid-based reverse genetics system for influenza A viruses allows manipulation of the viral genome (Song et al., 2007; Sorrell et al., 2007). The system is currently used to map specific amino acid residues involved in the interspecies transmission of H9, H2 and H7 influenza viruses, which are believed, along with H5 viruses, to have the greatest potential to become pandemic strains. Our studies are elucidating the most important factors in the spread and pathogenesis of influenza viruses from aquatic bird reservoirs to land-based poultry and mammals.
Plasmid-based reverse genetics (Hoffmann et al., 2000; 2001) is also being used for generation of reassortant H7N2, LPAI viruses. Genes HA, NP, NA, M and NS from different strains have been amplified using universal primers (Hoffmann et al., 2001). The amplification of other genes of interest is in progress. The amplified genes were cloned in pHW2000 (acquired from St. Jude Children's Research Hospital). We propose to modify M1 and NS1 segments of the virus to generate temperature sensitive (ts) and attenuated viruses for evaluation as vaccine candidates.
Avian pathogenic Escherichia coli (APEC) involved in respiratory disease is a major cause of economic loss for the poultry industry. It is well established that certain APEC serogroups are most commonly associated with colibacillosis in poultry, including O1, O2, and O78 strains (Nolan, L.K., 2003). It is thought that most APEC infections are secondary in nature, affecting an immunocomprimised bird previously infected with a primary viral or bacterial agent. However, recent genotyping data suggests that some APEC isolates appear to be primary pathogens, while others appear to be commensal isolates taking advantage of an immunocomprimised host (Johnson et al., in press).
INFECTIOUS BRONCHITIS VIRUS (IBV). Infectious bronchitis (IB) is an economically important disease of commercial chickens. The causative agent, IB virus (IBV), a coronavirus, is highly contagious, causing respiratory, kidney disease and egg production losses (Cavanagh and Gelb, 2008). Three major viral proteins comprise the virion: spike (S), membrane (M) and the nucleocapsid (N) (Cavanagh and Gelb, 2008). The S1 subunit of S induces neutralizing antibodies (Cavanagh et al., 1988) and is one of the least conserved segments of the genome. Variability within the S gene is largely responsible for the vast genotypic and serotypic diversity of IBV. Field strains differing from vaccines may not cross-protect, creating a challenge when developing vaccine programs. Monitoring IB by isolating, identifying and characterizing field isolates is important in commercial (Gelb et al., 1997; Gelb et al., 1991; Gelb et al., 1983; Gelb et al., 2005, Winterfield et al., 1964; Ziegler et al., 2002). Understanding IB s antigenic diversity, pathogenicity, persistence and other important properties will require study of the complete genome. A recent report described a new and unique genome structure of some Australian strains (Mardani et al., 2008).
INFECTIOUS BURSAL DISEASE VIRUS (IBDV). Infectious bursal disease (IBD), caused by the birnavirus, infectious bursal disease virus (IBDV), is characterized by destruction of the lymphoid cells of the bursa of Fabricius (BF). Severe B-cell suppression (Kibenge et al., 1988) leads to immunosuppression resulting in exacerbation of host susceptibility to other disease agents, as well as a reduction of the ability of the chicken to respond to vaccines (McFerran et al., 1980). Two distinct serotypes of IBDV exist. Serotype I strains are pathogenic for chickens, whereas serotype II viruses, mostly isolated from turkeys, are avirulent for chickens (Ismail et al., 1988). Antigenic variant strains break through the immunity provided by vaccination (Snyder et al., 1988; Ismail and Saif, 1991). Ongoing monitoring-isolation, identification and characterization- of variants is critical to assess the effectiveness of vaccines and their ability to prevent/control immunosuppression. Challenge trials using broilers are an important tool to assess the effectiveness of breeder vaccination programs, the primary strategy in controlling IBD. Detection and differentiation of IBDV is accomplished using monoclonal antibodies, PCR-gene sequencing, PCR-restriction fragment length polymorphisms (RFLP), and real time RT-PCR (Snyder et al., 1988; Lin et al., 1994; Jackwood et al., 1997; Jackwood and Sommer, 1997; 1998; 2002; Peters et al., 2005; Wu et al., 1992; 2007). The potential introduction of very virulent IBDV (vvIBDV) in U.S. poultry is a serious concern because the virus causes high mortality (van den Berg et al., 2000).
The genome of IBDV consists of two segments of double-stranded RNA. The smaller segment B encodes VP1, a 97-kDa multifunctional protein with polymerase activity (Kibenge et al., 1988). The larger segment A encodes a 110-kDa-precursor protein in a single large open reading frame (ORF), which is processed into mature VP2, VP3 and VP4 proteins (Hudson, et al., 1986). VP2 and VP3 are major structural proteins of the virion, whereas VP4 is a minor protein involved in the processing of the precursor protein (Azad et al., 1987; Jagadish et al., 1988). VP2 is the major protective immunogen and is responsible for inducing neutralizing antibody. Antigenic determinants also reside in VP2 and variability within the gene is noted (Bayliss et al., 1990; Heine et al., 1991; Vakharia et al., 1994). VP3, as a group specific antigen, forms a complex with VP1, which may have an essential role for the morphogenesis of the virion (Lombardo et al., 1999). Segment A also encodes a 17-kDa nonstructural VP5 protein from a small ORF, which is found in infected cells (Mundt et al., 1995). VP5 is not essential for replication and a reverse genetics-generated VP5-deficient mutant did not induce bursal lesions in chickens (Yao et al., 1998). Expression of VP2,4,3 polyprotein suppressed proliferation of bursal lymphocytes independent of infection as well as suppressed cell growth of transiently transfected DT40 B lymphocytes, suggesting that IBDV polyprotein is a mediator of immunosuppression (Peters et al., 2004).
In addition to recombinant subunit vaccines, other strategies to control IBDV infection, include ribozyme (Akin et al., 1999), DNA vaccination (Chang et al., 2002; 2003), and reverse genetics (Mundt et al., 2003) have been pursued for anti-viral infection or vaccination of chickens. However, DNA plasmid carrying VP2 gene of IBDV, co-administered with another DNA plasmid carrying chicken interferon-r gene, failed to achieve protection against IBDV challenge (Hsieh et al., 2006). Nevertheless, chickens primed with a DNA plasmid carrying the VP2 gene and subsequently, boosted with killed IBD vaccine achieved 100% protection (Hsieh et al., 2007).
NEWCASTLE DISEASE VIRUS (NDV). NDV infects various species of wild and domestic birds (Alexander, 1989; Alexander and Senne, 2008). Newcastle disease (ND) varies in its degrees of severity, ranging from a subclinical infection to severe disease causing very high mortality. NDV strains are categorized into three main pathotypes for chickens: lentogenic (low virulence) (Hitchner and Johnson, 1948), mesogenic (intermediate virulence) (Beaudette and Black, 1946), and velogenic (high virulence) strains (Beach, 1942; Doyle, 1927). Regardless of the severity of the pathotype, vaccination usually protects birds from clinical disease (Boney et al., 1975). NDV is a member of the genus Avulavirus in the family Paramyxoviridae (Fauquet et al., 2005). The RNA genome is single stranded, negative-sense, consisting of 15,186 nt (Krishnamurthy et al., 2000). Using reverse genetics, we recovered lentogenic strain LaSota and mesogenic strain Beaudette C from cloned cDNAs (Krishnamurthy et al., 2000; Huang et al., 2001). Reverse genetics allowed us to evaluate the role of the V protein, HN protein, and the internal proteins (N, P, and L) in virulence. Our study suggested that the V protein significantly contributes to virulence and inhibits alpha IFN (Huang et al., 2003). The HN protein determines tissue tropism but plays a minimal role in virulence (Huang et al., 2004a; Panda et al., 2004). We also demonstrated that the L gene contributes to virulence, while the N and P genes play minor roles (Rout and Samal, 2008). We have also used recombinant LaSota as vectors for vaccine development against emerging human infections (i.e., H5N1 avian influenza, SARS, and Ebola) and other pathogens (i.e., IBDV) (DiNapoli et al., 2007a; 2007b; Ge et al., 2007; Huang et al., 2004b). NDV genes have been incorporated into viral vectors for multivalent and in ovo delivery (Sun et al., 2008).
AVIAN PNEUMOVIRUS (APV). Avian pneumovirus (APV) causes an acute respiratory disease of turkeys (Goyal et al., 2003). APV infection was first detected in the U.S. in Colorado in 1996, in Minnesota in 1997, and more recently has spread to adjacent states. The disease causes economic losses due to mortality, reduced egg production, bodyweight loss, and increased condemnations. Mortality may reach 30% in cases complicated by secondary bacterial infections. APV belongs to the genus Metapneumovirus, subfamily Pneumovirinae of the family Paramyxoviridae (Lamb and Kolakofsky, 2001). The RNA genome is a non-segmented, negative-sense of 14,150 nt. The genomic is organized into eight genes; 32-leader-N-P-M-F-M2-SH-G-L-trailer-52 (Govindarajan and Samal, 2005). We have recently developed a reverse genetics system for APV/C strain Colorado (Govindarajan et al., 2006). This system allows us to recover infectious APV/C from cloned cDNAs. We have also shown that APV can be engineered as a vector to express foreign genes. The establishment of the rescue system will facilitate the study of APV molecular biology, disease pathogenesis and to develop novel recombinant vaccines.
INFECTIOUS LARYNGOTRACHEITIS VIRUS (ILTV). Infectious laryngotracheitis (ILT) has become one of the most economically important diseases of commercial broiler chickens in the U.S. Numerous outbreaks of have been reported in most poultry production areas; Alabama (Dawe, 1995), California (Linares et al., 1994), the Delmarva peninsula region (Keeler et al., 1993; Ritter, 2000, 2007; and Stewart-Brown, 2006), Georgia (Andreasen et al., 1989), Mississippi (Montgomery et al., 2007), North Carolina (Guy et al., 1989) and Pennsylvania (Davison and Miller, 1988; Keller et al., 1992). An outbreak that occurred on the Delmarva peninsula from 1998-2000 cost the broiler industry $4 million (Ritter, 2000).
ILT is characterized by moderate to severe respiratory distress, conjunctivitis, and tracheitis (Guy and Bagust, 2003). Affected flocks experience increased mortality, poor feed conversion and reduced growth. Infectious laryngotracheitis virus (ILTV) is an alphaherpesvirus. The virus does not survive for extended periods outside the host, and is sensitive to heat, drying, and disinfection (Guy and Bagust, 2003).
Epidemiologic investigations of recent outbreaks in the U.S. point to common sources of infection such as recovered or vaccinated birds, and the introduction of the virus via movement of contaminated humans/equipment or infected poultry at processing (live haul). Vaccination with modified live chicken embryo origin (CEO) vaccines has been used in areas where ILT is endemic but can result in the occurrence of long-term carrier birds due to the ability of the virus to become latent in the sensory ganglia (Bagust et al., 1986; Williams et al., 1992; Keeler, 2001).
Live ILTV CEO vaccines are commonly used in broilers and are administered via the drinking water or aerosol spray. However, these methods often result in a high proportion of chickens that fail to develop protective immunity (Robertson and Egerton, 1981). In addition, the use of CEO vaccines has been associated with the spread of vaccine virus to non-vaccinates causing increasing vaccine reactions, a result of bird-to-bird passage and the production of latently-infected carriers (Guy and Bagust, 2003). Transmission from infected to non-infected farms within a wind vector has been implicated in recent outbreaks (Johnson et al., 2005). Ritter (2007) described two modes of spread of ILT. Walk-in transmission occurs when clinical signs appear near the poultry house door, indicating spread by people. Blow-in transmission occurs when signs appear along sidewall air inlets of a poultry house located near a road, indicating spread by wind carrying the virus from live haul trucks.
Although progress has been made in the areas of biosecurity, vaccination, and other prevention measures, knowledge gaps still exist in the persistence and recurrence of ILTV. To fully understand the pathogenesis and reasons for recurring outbreaks, additional research is needed on viral dose required, and its effects on incubation period, duration of shedding, latency, and survival of ILTV in the poultry house environment.
Objectives
-
Identify reservoirs of infectious respiratory disease agents in wild birds and poultry.
-
Develop improved diagnostic capabilities including real time PCR as well as other rapid on-farm tests for economically important respiratory diseases.
-
Investigate the pathogenesis and polymicrobial interactions of specific infectious agents associated with poultry respiratory diseases (this includes interactions with underlying immunosuppressive agents).
-
Develop new prevention and control strategies for poultry respiratory diseases.
Methods
Objective 1. Identify reservoirs of infectious respiratory disease agents in wild birds and poultry.AVIAN INFLUENZA VIRUS (AIV) (AUBURN, CONNECTICUT, DELAWARE, INDIANA, MARYLAND, MINNESOTA, OHIO)
Surveillance will be performed in commercial poultry, backyard poultry, live bird markets and auctions, wild aquatic birds, and swine. AIV will be detected by virus isolation real-time RT-PCR. AIV specimens will be sent to USDA National Veterinary Services Laboratory in Ames, Iowa for HA and NA typing. The HA genes of selected isolates, including all H5 and H7 subtypes, will be sequenced and compared to sequences in GenBank. The survivability of AIVs in poultry litter at different times, temperatures, and litter moistures in the poultry house environment will be examined.
The susceptibility of guinea fowl, turkeys, domestic ducks, and geese to AIVs from wild bird reservoirs (Pereda et al., 2008) will be determined. Molecular changes associated with adaptation and transmission of AIVs in domestic birds (Sorrell et al., 2007) and the role of aerosol, particle size, and virus morphology for efficient transmission in avian species will be investigated.
The antiviral resistance of wild birds isolates by sequencing the N gene and using the fluorescence-based neuraminidase assay. AIV survival in the wild and domestic bird environment will be examined.
AVIAN PARAMYXOVIRUS (GEORGIA) A serological survey Will be conducted in U.S. poultry to determine the prevalence of antibodies to avian paramyxovirus 2, 3, 4, 6, 7, 8, and 9.
AVIAN PNEUMOVIRUS (APV) (INDIANA, OHIO) APV sero-surveillance studies will be conducted in hunter-killed waterfowl, trapped wild birds and in commercial turkeys. Virus detection from respiratory and/or gastrointestinal specimens will also be performed and isolates will be typed.
AVIAN POXVIRUS (ILLINOIS) Avianpox viruses isolated from domestic and wild birds will be genetically characterized and differentiated
E. COLI (DELAWARE) Avian E. coli from respiratory-diseased broiler chickens will be isolated and characterized by O antigen serotyping (Blanco et al., 1998), molecular virulence factors tsh, iss, iucC, IntI and TraT (Johnson et al., 2004; Kaipainen et al., 2002), and pulse field gel electrophoresis (PFGE) to establish diversity (Goering 2004; Tenover et al., 1995).
INFECTIOUS BRONCHITIS VIRUS (IBV) (CONNECTICUT, DELAWARE) IBV surveillance in poultry will be performed. Isolates will be identified using PCR-sequencing (Gelb et al., 2005) and RAPD tests specific for the S1 subunit.
INFECTIOUS BURSAL DISEASE (IBDV) (DELAWARE, OHIO) IBDV surveillance will be conducted in commercial chickens. Bursae will be collected weekly from broilers up to 4-weeks of age.
INFECTIOUS LARYNGOTRACHEITIS VIRUS (ILTV)(AUBURN, DELAWARE, GEORGIA, MARYLAND) ILTV surveillance in commercial chickens will be performed. The minimum infective dose, latency, incubation period, and duration of shedding of ILTV in will be determined in chickens. Potential sources of infection in the poultry house environment will be identified such as dust, litter, water drinkers, feeders, walls, fans, and darkling beetles. Specimens will be tested by virus isolation and real time (RT)-polymerase chain reaction (PCR). Isolates will be characterized using PCR and restriction fragment length polymorphism (RFLP) to determine if they are wild type or vaccine origin. The potential establishment of ILTV and circulation on farms where vaccines were recently used, where ILT recently occurred, and in vaccine zone buffer area will be studied.
MYCOPLASMA (GEORGIA) Pathogenic avian mycoplasmas in poultry will be isolated and characterized.
NEWCASTLE DISEASE VIRUS (NDV) (DELAWARE, GEORGIA) NDV field strains from diseased poultry will be isolated and characterized.
Objective 2. Develop improved diagnostic capabilities including real time PCR as well as other rapid on-farm tests for economically important respiratory diseases.
AVIAN INFLUENZA VIRUS (AIV) (AUBURN, OHIO, GEORGIA) Methods for AIV detection will be evaluated by comparing results obtained using the HA test, antigen capture (AC) ELISA, and RRT-PCR. A suspension array system for detecting and subtyping AIV will be developed. A rapid multiplex assay for identifying HA subtypes using a microsphere-based assay in combination with branched DNA (bDNA) signal amplification technology will be developed.
AIV serological tests for differentiating infected from vaccinated animals (DIVA) will be developed.
AVIAN POXVIRUS (ILLINOIS) Genetic-based methods will be used to investigate the identification of poxviruses in samples in which virus isolation is not possible by conventional procedures.
INFECTIOUS BRONCHITIS VIRUS (IBV)(DELAWARE) Variant field isolates will be sequenced to determine their genome organization (Mardani et al., 2008).
INFECTIOUS LARYNGOTRACHEITIS VIRUS (ILTV)(DELAWARE, GEORGIA) ILTV gene targets will be identified for diagnostic applications and for relating genetic elements to phenotypic characteristics, e.g. pathogenicity, latency, and persistence. BAC cloning of ILTV will be performed to facilitate whole genome sequencing. ILTV antibody responses to recombinant vaccines and baculovirus-expressed ILTV glycoproteins will be evaluated.
MYCOPLASMA (GEORGIA) The palatine cleft will be studied as a potential sample site for the improved molecular detection of pathogenic avian mycoplasmas.
NEWCASTLE DISEASE VIRUS (NDV) No activity planned.
ORNITHOBACTERIUM RHINOTRACHEALE (ORT) (MINNESOTA, USDA-NADC) Reagents to facilitate the diagnosis of ORT will be prepared. Proteomic techniques will be used to identify immunoreactive antigens and will be tested as ELISA reagents. Specific peptide sequences identified from the studies will be synthesized and used in ELISA. A 3-primer PCR assay for identification of ORT will be developed and optimized. Universally conserved forward and reverse primers, as well as an ORT-specific forward primer, all derived from the 16S rRNA genes, will be used.
Objective 3. Investigate the pathogenesis and polymicrobial interactions of specific infectious agents associated with poultry respiratory diseases (this includes interactions with underlying immunosuppressive agents).
AVIAN INFLUENZA VIRUS (AIV) (DELAWARE, MARYLAND, MINNESOTA, OHIO) H5 LPAI isolates of wild bird origin will be characterized in chickens, ducks and turkeys. High and low poultry-adapted viruses and an isolate that shows preferential replication and transmission in ducks and turkeys, but not in chickens will be studied. The genetic basis of interspecies transmission will be studied. Innate resistance will be studied through the expression of toll like receptors and cytokine and chemokine responses following influenza infections of poultry.
The pathogenicity of wild aquatic bird and domestic poultry isolates for poultry; broiler chickens, ducks, and turkeys will be characterized. Clinical disease, virus/genome shedding in the trachea and cloaca, microscopic pathology, and seroconversion will be evaluated. The molecular basis of transmissibility of AIVs in land-based avian intermediate hosts will be determined. Reassortant viruses containing one or more genes from one transmissible AIV strain and the rest from a non-transmissible AIV strain will be engineered (Song et al., 2007). Reverse genetics viruses will contain genes from land-based avian and mammalian influenza viruses. Transmission will be tested in avian species and in organ cultures to determine the potential of domestic birds as amplifiers of potential pandemic viruses (Wan et al., 2007). Molecular markers of pathogenicity for chickens of HP H5N1 viruses other than the polybasic amino acid HA cleavage region will be studied (Hulse et al., 2004). Host chicken responses to the viruses will be characterized and will define the contributions of PB1F2 and NS1 to the virulence of AIVs for birds and mammals (Jackson et al., 2008).
A chicken mesenchymal stem cell model to study pathogenic mechanisms of AIV will be developed.
AVIAN PNEUMOVIRUS (APV) (USDA-SEPRL) The pathogenic properties of APV in turkeys using the Colorado and Minnesota isolates and their cell culture-passaged variants will be studied. Comparative sequence analysis will be performed. Viral pathogenesis will be evaluated based on clinical signs, viremia, virus shedding, and antibody response (Kapczynski, 2004). Choanal swabs will be tested by virus isolation and titration in susceptible cell cultures (Kapczynski, 2004; Yu and Estevez, 2006). APV antibodies will be detected by ELISA and virus-neutralization. APV recombinants with alterations/deletions within specific genes will be generated. Recombinant viruses will be characterized in cell culture and animals. Attenuated APV recombinants will be tested as potential vaccine candidates. Vaccine-induced protection will be assessed by challenge of immunity. DIVA (differentiating infected from vaccinated animals) diagnostic tests will be developed (Pasick, 2004) using competitive ELISA targeting different viral epitopes.
E. COLI (DELAWARE, MINNESOTA) The polymicrobial interactions of Mycoplasma gallisepticum, NDV, or IBV with avian pathogenic E. coli (APEC) involved in respiratory disease in chickens will be investigated. E. coli pathogenicity will be assessed using virulent IBV infection to predispose broiler chickens to E. coli infection to distinguish between pathogenic and non-pathogenic E. coli (Smith et al., 1985; Bree et al., 1989). Several well-characterized APEC field isolates representing suspected primary pathogens and suspected commensal opportunists will be used as bacterial challenge strains. In other experiments, broiler chicks will receive one of the following: 1) IBV; 2) Mycoplasma gallisepticum; 3) NDV; or 4) no inoculation. Birds will be challenged with either an APEC isolate which is a suspected primary pathogen, or one which is a suspected commensal opportunist. At 6 days post-challenge, tissues will be examined for the presence of the above-mentioned organisms using qRT-PCR (Gelb et al., 2007; Grodio et al., 2008; Skyberg et al. 2008).
INFECTIOUS BRONCHITIS VIRUS (IBV) (GEORGIA, MINNESOTA) The potential interference between ILTV, NDV and IBV live vaccines in chickens will be investigated. The interaction of IBV, E. coli, and mycoplasma involved in respiratory disease in chickens will be studied.
INFECTIOUS BURSAL DISEASE VIRUS (IBDV) (DELAWARE, INDIANA, MINNESOTA, OHIO) The pathogenic and antigenic properties of field strains will be characterized. IBDVs from broiler chickens will be sequenced-VP2 (D. Jackwood & Sommer, 1997). Progeny challenge of immunity trials will be performed using commercial broiler chicks to assess the maternal protection provided via breeder vaccination.
Viral-host cell interactions of IBDV will be studied. Chickens will be inoculated with engineered IBDV with mutations in structural and/or non-structural genes (Mundt and Vakharia, 1996). Histopathology, flow cytometric analysis of the B-lymphocytes, B-lymphocyte proliferation assay (Peters et al., 2004), and bursal cytokine mRNA profiles (Sijben et al., 2003) will be performed. IBDV strains and isolates obtained from U.S. and Asia will be characterized. The hypervariable region of the VP2 gene (Lin, 1994; Vakharia et al., 1994) will be sequenced and analyzed. Poultry will be challenged with IBDV strains and host responses characterized (Snyder et al., 1988; Ruby et al., 2006). The impact of IBDV infection on the pathogenicity of AIV and avian mycoplasma will be determined. Histopathology, ELISA, cytokine mRNA profiles, and viral and bacterial loads (Peters et al., 2005) will be performed.
The mechanisms of pathogenesis and immunosuppression by IBDV and its interactions with common respiratory viruses will be examined. The frequency of occurrence on broiler and layer farms will be examined to identify IBDV strains present during a respiratory disease outbreak. RT-PCR will be used to detect virus and nucleotide sequencing of the hypervariable region of VP2 will be performed.
The persistence and distribution of IBDV in turkeys will be studied. Chickens with and without antibodies to IBDV will be challenged and evaluated for viral persistence.
Molecular epidemiologic studies will be conducted on IBDV isolates from broiler and layer chicken flocks to identify genetic diversity and to correlate molecular characteristics with phenotypic properties of the virus. IBDV will be identified in bursae using RT-PCR and sequencing of the hypervariable region of VP2. Isolates with unique combinations or uncommon amino acids at key positions in VP2; 222, 253, 254, 279, 284, 318 and 323 will be studied.
INFECTIOUS LARYNGOTRACHEITIS VIRUS (ILTV) (ARKANSAS, DELAWARE, GEORGIA) ILTV virulence genes will be identified. To identify virulent genes, deletion mutants of ILTV will be inoculated to SPF chickens to determine their pathogenicity. Viral genes including surface proteins will be deleted using homologous recombination (Devlin et al., 2007; Fuchs et al., 2000). An ILTV transmission model will be developed for comparing recent isolates, reference strains and chicken embryo origin (CEO) vaccines. The model will use broiler chickens assigned to three groups in the same colony house; Inoculates (with ILTV via the combined eyedrop/intratracheal routes), direct contacts (will share feed and drinking water; and indirect contacts (separated from the inoculates and direct contacts via a wire fence). Broilers will be evaluated for clinical disease (eye lesions, and coughing), virus and seroconversion. The potential for interference between ILTV, NDV and IBV live vaccines in chickens will be investigated.
MYCOPLASMAS (GEORGIA, MINNESOTA) The genetic relatedness and pathogenicity of selected field isolates of respiratory viruses and mycoplasmas will be studied in chickens.
NEWCASTLE DISEASE VIRUS (NDV) (GEORGIA, MARYLAND, USDA-SEPRL) The potential for interference between ILTV, NDV and IBV live vaccines in chickens will be investigated.
Molecular determinants and mechanisms of NDV pathogenesis will be identified. Functions of genes in viral replication and pathogenesis will be investigated using a chimeric virus (Yan and Samal, 2007; Rout and Samal, 2008).
Infectious clones will be engineered to study the molecular basis of tissue tropism and the basis for variation in clinical disease associated with different NDV strains. Identification of the genetic determinants of different clinical disease forms is expected to reveal additional virulence determinants to the well-known virulence determinant-the fusion gene cleavage activation site. The pathotypes of rescued virus populations will be determined by mean death time (MDT) of embryonating chicken eggs and intracerebral pathogenicity index (ICPI) and intravenous pathogenicity index (IVPI) assays (Alexander, 1998). Current live and inactivated vaccines are formulated utilizing the lentogenic B1 and LaSota strain originally isolated in the 1940s which are antigenically and genetically distinct from current virulent strains. SEPRL will investigate the efficacy of vaccines produced from more recent NDV isolates and determine if they provide improved protection. Initially, inactivated vaccines will be produced using contemporary NDV field isolates. Then safe live vaccines with genetic elements of more recent isolates will be engineered. Isolates will be obtained from surveillance of wild birds and live bird markets to identify contemporary NDV strains. The isolates will be characterized by sequencing, characterizing their virulence in poultry, and creating a database linking geographical, temporal, and biological data to the genomic information.
ORNITHOBACTERIUM RHINOTRACHEALE (ORT) (USDA-NADC) Virulence factors for ORT using proteomics and iTRAQ technologies will be identified.
POULTRY ENTERITIS SYNDROME (PES) (MINNESOTA) Poult enteritis syndrome (PES) will be experimentally reproduced and interactions of PES and respiratory infections will be studied.
Objective 4. Develop new prevention and control strategies for poultry respiratory diseases.
AVIAN INFLUENZA VIRUS (AIV) (AUBURN, CONNECTICUT, DELAWARE, INDIANA, OHIO)
In-house composting to inactive AIV (Malone et al., 2006) will be studied. Egg-grown AIV will be added to create a contaminated compost material. Residual live AIV will be determined (Dormitorio et al., 2007).
The potential of the Mx gene to confer resistance of broilers to AIV challenge will be assessed. Broilers from two commercial breeder companies will be evaluated using HP H5 AIV challenge. Broilers from each source will be tested for expression (high vs. low) of Mx gene.
RNA interference (RNAi) is recently discovered natural antiviral mechanism that was shown to be present in chickens. High efficiency and specificity against their target, ability to function in variety of cell type made RNAi the most widely used agent for sequence specific suppression of viral replication. Despite numerous successes the biggest weakness of current approach is the ease of viral escape from suppression sometimes through a single mutation which is especially relevant for RNA viruses such as influenza which show high rate of mutation. Several approaches will be tested for the ability to prevent influenza escape including anti-influenza shRNA cocktails, second generation escape resistant microRNA, and expression of extended RNAi hairpins.
Site-directed mutagenesis for development of an attenuated AIV vaccine will be developed (Taylor et al., 1985; Hoffmann et al., 2000; Babapoor et al., 2007). Vaccine candidates will be characterized with wild type viruses in vitro and then in chickens and turkeys by evaluating immunogenicity, virus replication, and transmissibility. The H, N, and M genes of AIV will be used to develop DNA-based and vector-based molecular vaccines. The experimental approaches will be similar to those described for IBD. Attenuated DIVA (differentiating infected from vaccinated animals) vaccines for AIV will be developed by selecting NS1 deletion variants from TK/OR/71-del (H7N3) (Wang et al., 2008). Reassortants will also be engineered for HA subtypes H3 and H5 by incorporating a selected NS gene into recent field strains of those subtype viruses using the traditional reassortment method and reverse genetics. Further attenuation of the variants will be conducted by introducing five temperature sensitive (ts) mutations (Song et al., 2007) in the PB1, PB2, and NP genes of candidate vaccine strain (NS variants). The different vaccine candidates will be characterized/compared with wild type viruses in vitro and in chickens and turkeys. The protection of a replication-defective adenovirus recombinant vaccine (Vaxin, Inc.) containing the H5 and H7 HA genes of AIV will be studied further. Research has shown the vaccine protected chicks vaccinated in ovo and day of age against HP H5 AIV (Toro and Suarez, 2007). A transgenic yeast vaccine (for drinking water vaccination) expressing the HA from a LP AIV (duck isolate) will be developed. AIV antibody HI titers of chickens given the experimental yeast vaccine will be compared to titers of chickens given a commercial inactive vaccinated (Wu et al., 2004).
AVIAN PNEUMOVIRUS (APV) (MARYLAND, MINNESOTA) A safe and effective recombinant APV vaccine will be developed. Recombinant APVs will be generated entirely from cloned cDNA based on independent mechanisms of attenuation (Govindarajan et al., 2006). Vaccine candidates will be evaluated for their ability to protect turkeys vs. APV challenge. The engineered vaccine viruses will also be marker vaccines enabling easy but accurate serological differentiation between infected and vaccinated birds.
Using reverse genestics, a biomarker containing recombinant APV will be developed to differentiate vaccine from wild type APV. The N, P, M2 and L genes will be co-transfected along with the complete AMPV cDNA in order to produce infectious virions. Different biomarkers, GFP, 6x His tag, or »DNA, will be evaluated for use in the recombinant virus (Kong et al. 2006; 2007).
INFECTIOUS BRONCHITIS VIRUS (IBV) (CONNECTICUT, DELAWARE) The efficacy of recombinant IBV DNA vaccines to protect chickens against heterologous strain challenge will be evaluated. The pathogenicity of selected IBV field isolates (novel S1 gene variants) for commercial broilers will be determined as well as the potential protective efficacy of live virus vaccines in challenge of immunity trials.
INFECTIOUS BURSAL DISEASE VIRUS (IBDV) (INDIANA) DNA vaccination for chickens will BE optimized to minimize reversion to virulence and environmental contamination. To enhance immunity (Chang et al., 2002; Chang et al., 2003), SPF or IBDV-maternally-derived broiler chickens will be injected intramuscularly or in ovo with a chimeric DNA plasmid (carrying VP2 gene of IBDV and chicken cytokine or immunoregulatory gene in the same plasmid). A killed infectious bursal disease vaccine or a viral vector inserted with the VP2 gene will also be tested to further enhance immunity. Chickens will be challenged with homologous or heterologous IBDV and host immune response will be evaluated.
INFECTIOUS LARYNGOTRACHEITIS VIRUS (ILTV) (ARKANSAS, AUBURN, DELAWARE, MARYLAND) A safe, live virulent gene deletion ILT vaccine produced in a chicken cell line will be developed. Protection efficacies of the genetically attenuated vaccine will be tested by in vivo challenge studies.
On farm methods for reducing ILTV contamination will be developed including; poultry house heating, windrow composting, litter treatments, and insect control. Methods will be developed for reducing biofilms in drinkers which may harbor ILTV. Swabs taken from artificially ILTV adulterated dust, litter, drinkers and darkling beetles (adults and lesser meal worms larvae) will be tested by virus isolation/rPCR.
Extension programs on practical, cost-effective methods to prevent and control ILT outbreaks on the Delmarva peninsula will be developed and implemented. Findings will be shared with poultry companies and growers. Strategies will include identification of ILT hot spots and eliminating or reducing their impact in the transmission cycle. Findings on incubation period, shedding, and latency may also have an impact on vaccination strategies, culling, and transport of infected but marketable birds to the processing plant when it comes to timing and live haul routing.
The efficacy of recombinant vaccines to protect vs. ILT challenge will be evaluated.
AVIAN PNEUMOVIRUS (APV) (MINNESOTA) A killed mucosal vaccines to protect turkeys and chickens against respiratory viral infections including APV will be developed. A biomarker containing recombinant APV to differentiate vaccine from wild type virus will be designed. The G gene from a vaccine strain of APV (MN-1a-p63) will be cloned into an infectious clone to generate a recombinant vaccine.
NEWCASTLE DISEASE VIRUS (NDV) (DELAWARE) The efficacy of recombinant vaccines to protect vs. ND challenges will be evaluated.
P. MULTOCIDA (USDA-NADC) Improved live, non-virulent, modified P. multocida strains based on deletion of known virulence factors and a non-living vaccine based on bacterial ghost vaccine technology will be developed.
Measurement of Progress and Results
Outputs
- Collect and maintain new isolates of respiratory disease agents for research.
- Characterize emerging disease agents in terms of their pathogenicity, antigenicity and genetic composition.
- Identify key microbial genes and antigens associated with the immune response for use as vaccine candidates.
- Develop antisera, antigens, cloned genes, and primer sets for diagnostic test applications such as ELISA, PCR, gene arrays and protein assays.
- Develop disease control strategies via the use of vaccines, where appropriate, and specific biosecurity measures.
- Output 6. Provide research updates via presentations at regional, national , and international meetings (e.g. Transmissible Disease of Poultry Committee meeting at United State Animal Health Association Meeting and American Association of Avian Pathologists-sponsored meetings) to ensure information dissemination to all stake holders. <p> Output 7. Disseminate information through refereed publications. Sponsor symposia, workshops on research on poultry respiratory diseases, and informal discussions at national and international meetings. Continue to use our web site for dissemination of new information. http://www.wisc.edu/ncra/nc228index.htm
Outcomes or Projected Impacts
- Research will produce greater understanding of disease reservoirs, processes and control interventions.
- Reduced mortality and condemnation will result through the use of improved diagnostic tools, vaccines and bio-security measures.
- Consumers will enjoy safe, healthy, and competitively priced eggs and poultry meat products.
Milestones
(2009): Strive to increase collaborative efforts amongst NC-1019 and AICAP participants.(2011): Develop faster, accurate and sensitive diagnostic tools to detect/monitor poultry respiratory diseases.
(2013): Develop measures to identify and control high path AIV, ENDV and VVIBDV.
Projected Participation
View Appendix E: ParticipationOutreach Plan
Post annual reports on project web page (http://lgu.umd.edu/lgu_v2/homepages/home.cfm?trackID=10516).
E-mail annual reports to industry (producers and biologics manufacturers).
Present findings at industry-sponsored and professional society meetings.
Publish findings in referred journals.
Sponsor specific respiratory disease symposia at regional and national meetings.
Organization/Governance
GOVERNANCE The research technical committee shall consist of one technical committee representative from each cooperating agency as appointed or otherwise designated by the respective organization, and administrative advisor appointed by the Association of North Central Experiment Stations Directors, and a representative of the Cooperative State Research, Education and Extension Service (CSREES).
The Governance shall consist of an elected chair of this committee and a secretary. All officers are to be elected for (at least) renewable two-year terms to provide continuity. The assigned AA will provide administrative guidance.
All decisions by a committee will be made in an open and democratic process. To ensure fairness in decision making, voting is restricted to one vote per respective entity; an entity being a SAES, VMES, CES, federal agency, etc.
Meetings will be held annually at the time and place mutually agreed upon by the technical committee with the approval of the Administrative Advisor. COMMITTEE GOVERNANCE: Chair: The Chair of the committee is responsible for organizing the meeting agenda, conducting the meeting, and assuring that task assignments are completed. The chair is elected for at least a two-year term, to provide continuity. The Chair is eligible for re-election. The Chair will be responsible for continuity and coordination of the project, including such activities as the annual meetings, special meetings and/or workshops to address various aspects of the project, and communication with the administrative advisor and/or administering committees. Such coordination will occur continuously throughout the project period. The annual meeting of the technical committee will serve as a monitoring session for coordination.
Secretary: The Secretary is responsible for the distribution of documents prior to the meeting. The Secretary is also responsible for keeping records on decisions made at meetings (a.k.a. keeping the minutes), maintaining an updated roster of participants (as a list server), and assisting in the preparation of the accomplishments report (i.e., the SAES-422). The Secretary will serve as the chair in the absence of the elected chair.
Members: The Project Members are responsible for working to ensure the overall success of the NC-1019 by completing their proposed research, fostering collaborations, providing timely progress updates and information exchanges, and attending the annual meetings.
Literature Cited
Akin, A, Wu, C. C., and Lin, T. L. 1999. Amplification and cloning of infectious bursal disease virus genomic RNA segments by long and accurate PCR. J. Virol. Methods 82: 55-61.
Akin, A., Wu, C. C., and Lin, T. L. 1999. A ribozyme targeted to RNA polymerase gene of infectious bursal disease virus effectively cleaves and inhibits expression of the viral gene product. Acta Virologica 43:341-47.
Akin, A., Wu, C. C., Lin, T. L., and Keirs, R. W. 1993. Chemiluminescent detection of infectious bursal disease virus with a PCR-generated non-radiolabeled DNA probe. J. Vet. Diagn. Invest. 5:166-73.
Alexander, D. J. Newcastle disease and other paramyxoviruses. In: A Laboratory Manual for the Isolation and Identification of Avian Pathogens, eds. D. E. Swayne, J. R. Glisson, M. W. Jackwood, J. E. Pearson, and W. M. Reed, Kennett Square, Pennsylvania: American Association of Avian Pathologists, pp. 156-63. 1998.
Alexander, D.J., 1989. Newcastle disease, H.G. Purchase, L.H. Arp, C.H. Domermuth, and J.E. Pearson (ed.), A laboratory manual for the isolation and identification of avian pathogens, 3rd ed. American Association for Avian Pathologists, Kennett Square, PA p. 114-120.
Alexander, D.J. and D.A. Senne. Newcastle Disease. 2008. In: Diseases of Poultry, 12th ed. Y.M. Saif, A.M. Fadly, J.R. Glisson, L.R. McDougald, L.K. Nolan, and D.E. Swayne, eds. Iowa State Univ. Press, Ames, Iowa. pp 75-100.
Andreasen, J.R., J.R. Glisson, and P. Villegas. 1989. Differentiation of vaccine strains and Georgia field isolates of infectious laryngotracheitis virus by their restriction endonuclease fragment patterns. Avian Dis. 34:646-656.
Azad, A. A., Barrett, S. A., and Fahey, K. J. 1985. The characterization and molecular cloning of the double-strand RNA genome of an Australian strain of infectious bursal disease virus. Virol. 143:35-44.
Babapoor, S., Zeinab H., Dipu M. K. and M. Khan. 2007. Rescue of an avian influenza subtype H7 vaccine candidate modified virus using reverse genetic technique. Proc 79th Northeastern Conf. on Avian Dis. Lancaster, PA, pp 27.
Bagust, T.J. 1986. Laryngotracheitis (Gallid-1) herpesvirus infection in the chicken 4. Latency establishment by wild and vaccine strains of ILT virus. Avian Path. 15:581-95.
Bayliss, C. D., Spies, U., Shaw, K., Peters, R. W., Papageorgiou, A., Muller, H., and Boursnell, M. E. 1990. A comparison of the sequences of segment A of four infectious bursal disease virus strains and identification of a variable region in VP2. J. Gen. Virol. 71:1303-12.
Beach, JR. Avian pneumoencephalitis. 1942. Proc Ann Meet US Livestock Sanit. Assoc. Mtg. 46:203-223.
Beaudette, F.R. and J.J. Black. Newcastle disease in New Jersey. Proc. Ann. Mtg. US Livestock Sanit. Assoc. 49:49-58. 1946.
Birch-Machin I, Rowan A, Pick J, Mumford J, Binns M. 1997. Expression of the nonstructural protein NS1 of equine influenza A virus: detection of anti-NS1 antibody in post infection equine sera. J. Virol. Methods 65:255-263.
Blanco, J. E., Blanco, M. A., Jansen, W. H., Garca V., Vazquez, M. L., Blanco, J. 1998. Serotyping of Escherichia coli isolates from septicaemic chickens in Galicia northwest Spain, Vet. Microbiol. 61: 229-235.
Boney, Jr., W.A., H.D. Stone, K.G. Gillette and M.F. Coria. 1975. Viscerotropic velogenic Newcastle disease in turkeys: Immune response following vaccination with either viable B1 strain or inactivated vaccine. Avian Dis. 19:19-30.
Botton, M.L., R.E. Loveland, and T.R. Jacobsen. 1994. Site selection by migratory shorebirds in Delaware Bay, and its relationship to beach characteristics and abundance of horseshoe crab (Limulus polyphemus) eggs. The Auk 111:605-16.
Bree, A., M. Dho, and J. P. Lafont. 1989. Comparative infectivity of axenic and specific-pathogen-free chickens of O2 Escherichia coli strains with or without virulence factors. Avian Dis. 33: 134-39.
Brown , I.H. 2006. Advances in molecular diagnostics for avian influenza. Dev. Biol. (Basel). 124:93-7.
Bublot, M., Pritchard N., Swayne D.E., Selleck P., Karaca K., Suarez D.L., Audonnet JC, Mickle TR. 2006. Development and use of fowlpox vectored vaccines for avian influenza. Ann. N. Y. Acad. Sci. 1081:193-201. Cavanagh, D. and J. Gelb, Jr. 2008. Infectious bronchitis. In: Diseases of Poultry, 12th ed. Y.M. Saif, A.M. Fadly, J.R. Glisson, L.R. McDougald, L.K. Nolan, and D.E. Swayne, eds. Iowa State Univ. Press, Ames, Iowa. pp 117-135.
Cavanagh, D, PJ Davis, and APA Mockett. 1988. Amino acids within hypervariable region I of avian coronavirus IBV (Massachusetts serotype) spike glycoprotein are associated with neutralization epitopes. Virus Res. 11:141-50.
Cha, W. H. 2008. Application of multiplex branched DNA method for the detection and study of avian influenza virus. Ph.D. Dissertation. The Ohio State University.
Chang, H. C., Lin, T. L., and Wu, C. C. 2003. DNA vaccination with plasmids containing various fragments of large segment genome of infectious bursal disease virus. Vaccine 21:507-13.
Chang, H. C., Lin, T. L., and Wu, C. C. 2002. DNA-mediated vaccination against infectious bursal disease in chickens. Vaccine 20:328-35.
Christman. S.A., B.W. Kong, M.M. Landry, H. Kim, and D.N. Foster. 2005. Modulation of p53 expression and its role in the conversion to a fully immortalized chicken embryo fibroblast line. FEBS Letter. 79:6709-15.
Cloud, S. S. Antigen Detection Systems. 1989. In: A laboratory manual for the isolation and identification of avian pathogens. H. G. Purchase et al., eds. Kendall/Hunt Publishing Company. Dubuque, IA. 3rd ed.
Davison, S. and K. Miller. 1988. Recent ILT outbreaks in Pennsylvania. Proc 37th Western Poult Dis Conf. Davis, CA, 133-35.
Dawe, J.F. 1995. ILT experience-Alabama. Proc 30th Natl. Mtg. Poultry Health Proc. Ocean City, MD, pp.32-34.
Devlin. J.M., G.F. Browning, C.A. Hartley, and J.R. Gilkerson. 2007. Glycoprotein G deficient infectious laryngotracheitis virus is a candidate attenuated vaccine. Vaccine. 25:3561-66.
DiNapoli, J.M., Kotelkin, A., Yang, L., Elankumaran, S., Murphy, B.R., Samal, S.K., Collins, P.L. and Bukreyev, A. (2007a). Newcastle disease virus, a host range-restricted virus, as a vaccine vector for intranasal immunization against emerging pathogens. Proc. Natl. Acad. Sci. USA 104:9788-93.
DiNapoli, J.M., Yang, L., Suguitan, A. Jr., Elankumaran, S., Dorward, D.W., Murphy, B.R., Samal, S.K., Collins, P.L. and Bukreyev, A. (2007b). Immunization of primates with a Newcastle disease virus-vectored vaccine via the respiratory tract induces a high titer of serum neutralizing antibodies against highly pathogenic avian influenza virus. J. Virol. 81:11560-68.
DiNapoli, J.M., A. Kotelkin, L. Yang, S. Elankumaran, B.R. Murphy, S.K. Samal, P.L. Collins, and A. Bukreyev. Newcastle disease virus, a host range-restricted virus, as a vaccine vector for intranasal immunization against emerging pathogens. 2007. Proc. Natl. Acad. Sci. USA 104:9788-93.
Dormitorio, T. V., J.J. Giambrone, K. Guo and G. Hepp. 2007. Evaluation of methods for detecting influenza viruses in wild aquatic birds. Poult. Sci. Assn. Ann. Mtg. San Antonio, TX. July 16-18.
Doyle, TM. A hitherto unrecorded disease of fowls due to a filter passing virus. J. Comp. Pathol. Therap. 40:144-69. 1927.
Eterradossi, N. and Y.M Saif. Infectious bursal disease. 2008. In: Diseases of Poultry, 12th ed. Y. M. Saif, A. M. Fadly, J. R. Glisson, L. R. McDougald, L. K. Nolan, and D. E. Swayne, eds. Iowa State Univ. Press, Ames, Iowa. pp 185-208.
Fauquet, C.M., Mayo, M.A., Mariloff, J., Desselberger, U., and Ball, L.A. 2005. Virus Taxonomy 8th report of the international committee on taxonomy of viruses. Elsevier Academic Press, pp. 655-68.
Flagella, Michael, Son Bui, Zhi Zheng, Cung Toung Nguyen, Aiguo Zhang, Larry Poster, Yunquing Ma, Wen Yang, Kimberly L. Crawford, Gary K. McMaster, Frank Witney, Yuling Luo. 2006. A multiplex branched DNA assay for parallel quantitative gene expression profiling. Anal Biochem. 352:50-60.
Fouchier RA, Schneeberger PM, Rozendaal FW et al. 2004. Avian influenza A virus (H7N7) associated with human conjunctivitis and a fatal case of acute respiratory distress syndrome. Proc Natl Acad Sci USA 101:1356-61.
Fuchs. W.K., K. Ziemann, J.P. Teifke, O. Werner, and T.C. Mettenleiter. 2000. The non-essential UL50 gene of avian infectious laryngotracheitis virus encodes a functional dUTPase which is not a virulence factor. J. Gen. Virol. 81:627-38.
Garcia A., Johnson H., Srivastava D.K., Jayawardene D.A., Wehr D.R., Webster R.G. 1998. Efficacy of inactivated H5N2 influenza vaccines against lethal A/Chicken/Queretaro/19/95 infection. Avian Dis. 42:248-56.
Ge, J., G. Deng, Z. Wen, G. Tian, Y. Wang, J. Shi, X. Wang, Y. Li, S. Hu, Y. Jiang, C. Yang, K. Yu, Z. Bu, and H. Chen. 2007.Newcastle disease virus-based live attenuated vaccine completely protects chickens and mice from lethal challenge of homologous and heterologous H5N1 avian influenza viruses. J. Virol. 81:150-58.
Gelb, J., B. S. Ladman, M. J. Licata, M. H. Shapiro, and L. R. Campion. 2007. Evaluating viral interference between infectious bronchitis virus and Newcastle disease virus vaccine strains using quantitative reverse transcription-polymerase chain reaction. Avian Dis. 51:924-934.
Gelb, J., Jr., C. L. Keeler, Jr., W. A. Nix, J. K. Rosenberger and S. S. Cloud. 1997. Antigenic and S-1 genomic characterization of the Delaware variant serotype of infectious bronchitis virus. Avian Dis. 41:661-669.
Gelb, J., Jr., J. H. Leary, and J. K. Rosenberger. 1983. Prevalence of Arkansas-type infectious bronchitis virus in Delmarva peninsula chickens. Avian Dis. 27:667-678.
Gelb Jr., J., Y. Weisman, B.S. Ladman, and R. Meir. 2005. S1 gene characteristics and efficacy of vaccination against infectious bronchitis virus field isolates from the United States and Israel (1996 to 2000). Avian Pathology 34: 194-203.
Gelb, J., Jr., J. B. Wolff, and C. A. Moran. 1991.Variant serotypes of infectious bronchitis virus isolated from commercial layer and broiler chickens. Avian Dis. 35:82-87.
Giambrone, J.J., O. Fagbohun, and K.S. Macklin. 2008. Management practices to reduce infectious laryngotracheitis virus in poultry litter. J. App. Poult. Res. 17: 64-68.
Goering, R. V. 2004. Pulsed-field gel electrophoresis, In D. H. Persing, F. C. Tenover, J. Versalovic, Y.-W. Tang, E. R. Unger, D. A. Relman, and T. J. White (ed.), Molecular microbiology, diagnostic principles and practice. ASM Press, Washington, D.C. p. 185-196.
Govindarajan, D. and Samal, S.K. 2005. Analysis of the complete genome sequence of avian metapneumovirus subgroup C indicates that it possesses the longest genome among metapneumoviruses. Virus Genes 30: 329-31.
Govindarajan, D., Buchholz, U.J. and Samal, S.K. (2006). Recovery of avian metapneumovirus Subgroup C from cDNA: Cross recognition of avian and human metapneumovirus support proteins. J. Virol. 80:5790-97.
Goyal, S.M., Lauer, D., Friendshuh, K., and Halvorson, D.A. 2003. Seroprevalence of avian pneumovirus in Minnesota turkeys. Avian Dis. 47: 700-06.
Grodio, J. L., K. V. Dhondt, P. H. OConnell, and K. A. Schat. Detection and quantification of Mycoplasma gallisepticum genome load in conjunctival samples of experimentally infected house finches (Carpodacus mexicanus) using real-time polymerase chain reaction. Avian Path. 37:385-391. 2008.
Gross, W. G. 1994. Diseases due to Escherichia coli in poultry. In Escherichia coli in Domestic Animals and Humans. C. L. Gyles (ed.), CAB Intl: Wallingford, UK, pp. 237-259.
Guy, J. S. and T. J. Bagust. Laryngotracheitis. 2003. In: Diseases of Poultry, 11th ed. Y. M. Saif et al. Iowa State Univ. Press, Ames Iowa. pp. 149-150.
Guy, J.S. and T.J. Bagust. 2003. Laryngotracheitis. In Y.M. Saif, J.J. Barnes, J.R. Glisson, A.M. Fadly, L.R. McDougald, and D.E. Swayne (eds.). Diseases of Poultry, 11th ed. Iowa State Press. Ames, IA, 121-134.
Guy, J.S., H.J. Barnes, L.L. Munger, and L. Rose. 1989. Restriction endonuclease analysis of ILTV: comparison of modified live vaccine viruses and North Carolina field isolates. Avian Dis. 33:316-323.
Halvorson DA (2002). The control of H5 or H7 mildly pathogenic avian influenza: a role for inactivated vaccine. Avian Pathol. 31:5-12.
Heffels-Redmann and E. Kaleta, ed., 2006. Proc. V. International Symposium on Avian Corona- and Pneumoviruses and Complicating Pathogens. VVB Laufersweiler Verlag, Rauischholzhausen, Germany.
Heine, H. G., Haritou, M., Failla, P., Fahey, K., and Azad. A. 1991. Sequence analysis and expression of the host-protective immunogen VP2 of a variant strain of infectious bursal disease virus which can circumvent vaccination with standard type I strains. J. Gen. Virol. 72:1835-43.
Hirst M., C.R. Astell, M. Griffith, S.M. Coughlin, M. Moksa, T. Zeng, D.E. Smailus, R.A. Holt, S. Jones, M.A. Marra, M. Petric, M. Krajden, D. Lawrence, A Mak, R. Chow, D.M. Skowronski, S.A. Tweed, S. Goh, R.C. Brunham, J. Robinson, V. Bowes, K. Sojonky, S.K. Byrne, Y. Li, D Kobasa, T. Booth, and M. Paetzel. 2004. Novel avian influenza H7N3 strain outbreak, British Columbia. Emerg. Infect. Dis. 10:2192-95.
Hitchner, S.B. and E.P. Johnson. A virus of low virulence for immunizing fowls against Newcastle disease (avian pneumoencephalitis). Vet..Med. 43:525-530. 1948.
Hoffmann E, J. Stech, Y. Guan, R. G. Webster, and D. R. Perez. 2001. Universal primer set for the full-length amplification of all influenza A viruses. Arch. Virol. 146:2275-89.
Hoffmann, E. G. Neumann, Y. Kawaoka, G. Hobom, and R. G. Webster. 2000. A DNA transfection system for generation of influenza A virus from eight plasmids. Proc. Natl. Acad. Sci. 23; 97: 6108-13.
Horimoto T and Kawaoka Y. 2005. Influenza: lessons from past pandemics, warnings from current incidents. Nat. Rev. Microbiol. 3:591-600.
Hsieh, M. K., Wu, C. C., and Lin, T. L. 2007. Priming with DNA vaccine and boosting with killed vaccine conferring protection of chickens against infectious bursal disease. Vaccine, 25: 5417-27.
Hsieh, M. K., Wu, C. C., and Lin, T. L. 2006. The effect of co-administration of DNA carrying chicken interferon-g gene on protection of chickens against infectious bursal disease by DNA-mediated vaccination. Vaccine, 24: 6955-65.
Huang, Z., Elankumaran, S., Abdul, A. S., and Samal S. K. 2004b. A recombinant Newcastle disease virus (NDV) expressing VP2 protein of infectious bursal disease (IBDV) protects against NDV and IBDV. J. Virol. 78: 10054-63.
Huang, Z., Krishnamurthy, S., Panda, A. and Samal, S.K. 2001. High-level expression of a foreign gene from the most 3-proximal locus of a recombinant Newcastle disease virus. J. Gen. Virol. 82: 1729-1736.
Huang, Z., Krishnamurthy, S., Panda, A., and S.K. Samal. 2003. Newcastle disease virus V protein is associated with viral pathogenesis and functions as an alpha interferon antagonist. J. Virol. 77:8676-85.
Huang, Z., Panda, A., Elankumaran, S., Rockemann, D.D., and Samal, S.K. 2004a. The hemagglutinin-Neuraminidase protein of Newcastle Disease Virus determines tropism and virulence. J. Virol. 78:4176-84.
Hudson, P. J., McKern, N. M., Power, B .E., and Azad, A. A. 1986. Genomic structure of the large RNA segment of infectious bursal disease virus. Nucleic Acids Res. 14:5001-12.
Hulse D.J., Webster R.G., Russell R.J., Perez D.R. 2004. Molecular determinants within the surface proteins involved in the pathogenicity of H5N1 influenza viruses in chickens. J. Virol. 78:9954-64.
Ismail N. M., and Saif, Y. M. 1991. Immunogenicity of infectious bursal disease viruses in chickens. Avian Dis. 35:460-469.
Ismail, N. M., Saif, Y. M., and Moorhead, P. D. 1988. Lack of pathogenicity of five serotype 2 infectious bursal disease viruses. Avian Dis. 32:757-759.
Jackson D., Hossain M.J., Hickman D., Perez D.R., Lamb R.A. A new influenza virus virulence determinant: the NS1 protein four C-terminal residues modulate pathogenicity. 2008. Proc Natl Acad Sci U S A. Mar 18;105:4381-6. Epub 2008 Mar 11. PMID: 18334632 [PubMed - indexed for MEDLINE]
Jackwood, D. and M. W. Jackwood. 1998. Molecular identification procedures. In: A laboratory manual for the isolation and identification of avian pathogens. Eds. Swayne, et al. Amer. Assn. of Avian Pathologists, New Bolton Center, Kennett Square, PA. pp. 235-40.
Jackwood, D. J, and Sommer, S. E. 1997. Restriction Fragment length polymorphisms in the VP2 gene of infectious bursal disease virus. Avian Dis. 41:627-37.
Jackwood, D. J, and Sommer, S. E. 1998. Genetic heterogeneity in the VP2 gene of infectious bursal disease virus detected in commercially reared chickens. Avian Dis. 42:321-39.
Jackwood, D. J., and S. E. Sommer. 2002. Identification of infectious bursal disease virus quasispecies in commercial vaccines and field isolates of this double-stranded RNA virus. Virol. 304:105-113.
Jackwood, D. J., R. J. Jackwood, and S. E. Sommer. 1997. Identification and comparison of point mutations associated in classic and variant infectious bursal disease viruses. Virus Res. 49:131-37.
Jagadish, M. N., Staton, V. J., Hudson, P. J., and Azad, A. A. 1988. Birnavirus precursor polyprotein is processed in Escherichia coli by its own virus-encoded polypeptide. J. Virol. 62:1084-87.
Johnson, T. J., C. W. Giddings, S. M. Horne, P. S. Gibbs, R. E. Wooley, J. Skyberg, P. Olah, R. Kercher, J. S. Sherwood, S. L. Foley and L. K. Nolan. 2002. Location of increased serum survival gene and selected virulence traits on a conjugative R plasmid in an avian Escherichia coli isolate. Avian Dis. 46: 342-52.
Johnson, Y.J., N. Gedamu, M.M. Colby, M.S. Myint, S.E. Steele, M. Salem, and N. Tablante. 2005. Wind-borne transmission of infectious laryngotracheitis between commercial poultry operations. Int. J. Poult. Sci. 4:263-67.
Jones, Y. L. and D. E. Swayne. 2004. Comparative pathobiology of low and high pathogenicity H7N3 Chilean avian influenza viruses in chickens. Avian Dis. 48:119-128.
Kaipainen, T., T. Pohjanvirta, N. Y. Shpigel, A. Shwimmer, S. Pyorala and S. Pelkonen. 2002. Virulence factors of Escherichia coli isolated from bovine clinical mastitis. Vet. Microbiol. 85: 37-46.
Kapczynski, D. R. 2004. Development of a virosome vaccine for protection in turkeys against avian metapneumovirus subtype C. Avian Dis. 48:332-343.
Keeler, C.L. 2001. New approaches to control LT. Proc 36th Natl. Mtg. Poultry Health Proc.: Ocean City, MD. 30-31.
Keeler, C.L., J.W. Hazel, J.E. Hastings, and J.K. Rosenberger. 1993. Restriction endonuclease analysis of Delmarva field isolates of infectious laryngotracheitis virus. Avian Dis. 37:418-26.
Keller, L.H., C.E. Benson, S. Davison, and R.J. Eckroade. 1992. Differences among restriction endonuclease DNA fingerprints of Pennsylvania field isolates, vaccine strains, and challenge strains of ILTV. Avian Dis. 36:575-81.
Kermode-Scott B 2004. WHO confirms avian flu infections in Canada. British Med. J. 328:913.
Kibenge, F. S. B., Dhillon, A. S., and Russell, R. G. 1988. Biochemistry and immunology of infectious bursal disease virus. J. Gen. Virol. 69:1757-75.
Kong B.-W., L.K. Foster, and D.N. Foster. 2006. Comparison of Avian Cell Substrates for Propagating Subtype C Avian Metapneumovirus Virus Res. 116:58-68.
Kong B.-W., L.K. Foster, and D.N. Foster. 2007. Establishment of an immortal turkey turbinate cell line suitable for avian metapneumovirus propagation. Virus Res. 127:106-115.
Kong B.-W., L.K. Foster, and D.N. Foster. 2008. Species-specific deletion of the viral attachment glycoprotein of avian metapneumovirus. Virus Res. 132:114-121.
Kong B.-W., L.K. Foster, and D.N. Foster. 2008. A method for the rapid isolation of virus from cultured cells. BioTechniques. 44:97-99.
Kong B.-W., D. F. Carlson, S.C. Fahrenkrug and D.N. Foster. 2008. Application of the sleeping beauty transposon system to avian cells. Animal Genetics. 39:180-186.
Kreager K.S. 1998. Chicken industry strategies for control of tumor virus infections. Poultry Sci. 77: 1213-16.
Krishnamurthy, S., Huang, Z. and Samal, S.K. 2000. Recovery of a virulent strain of Newcastle disease virus from cloned cDNA: expression of a foreign gene results in growth retardation and attenuation. Virol. 278: 168-82.
Ladman, B., C. Pope, J. Gelb, Jr., R. Slemons, and C. Driscoll. 2008. Evaluation of Low Path Avian Influenza Viruses of Wild Bird Origin in Commercial Turkeys and Broiler Chickens. Proc. 144th Amer. Vet. Med. Assn./Amer. Assn. Avian Path. Ann. Mtg. New Orleans, LA.
Lamb, R.A. and Kolakofsky, D. 2001. Paramyxoviridae: The viruses and their replication. In: Fields Virology. 4th Ed. Eds. D.M. Knipe and P.M. Howley. Lippincott Williams and Wilkins. Philadelphia.
Landolt G.A. and C.W. Olsen. 2007. Up to new tricks? A review of cross-species transmission of influenza A viruses. Animal Health Res. Rev. 8:1-21.
Lasley F.A. 1986. Economics of avian influenza: control vs. noncontrol. In: Proceedings of the second international symposium on avian influenza. C.W. Beard (ed.). U.S. Animal Health Association: Richmond, VA, 390-399.
Lau, L.T., Fung, Y.W., Yu, A.C. 2006. Detection of animal viruses using nucleic acid sequence-based amplification (NASBA). Dev Biol (Basel). 126:7-15.
Lee, C. W., Senne, D.A., Suarez, D.L. 2004. Generation of reassortant influenza vaccines by reverse genetics that allows utilization of a DIVA (differentiating infected from vaccinated animals) strategy for the control of avian influenza. Vaccine 22: 3175-81.
Lee, C.W., Suarez, D.L. 2005. Avian influenza virus: Prospects for prevention and control by vaccination. Animal Health Res Rev. 6:1-15.
Lee C.W., Swayne D.E., Linares J.A., Senne D.A., Suarez D.L. 2005. H5N2 avian influenza outbreak in Texas in 2004: the first highly pathogenic strain in the United States in 20 Years? J. Virol. 79:11412-21.
Lee, C. W., Y. J. Lee, D. A. Senne, D. L. Suarez. 2006. Pathogenic potential of North American H7N2 avian influenza virus: A mutagenesis study using reverse genetics. Virol. 353:388-95.
Lee, C.W., and D. L. Suarez. 2004. Application of real-time RT-PCR for the quantification and competitive replication study of H5 and H7 subtype avian influenza virus. J. Virol. Methods 119:151-58.
Li F.C.K., Choi B.C.K., Sly T., Pak A.W.P. 2008. Finding the real case-fatality rate of H5N1 avian influenza. J. Epidemiol. Community Health. 62:555-559.
Lin, T. L., Wu, C. C., Rosenberger, J. K, and Saif, Y. M. 1994. Rapid differentiation of infectious bursal disease virus serotypes by polymerase chain reaction. J. Vet. Diagn. Invest. 6:100-02.
Linares, J.A., A.A. Bickford, G.L. Cooper, B.R. Charlton, and P.R. Woolcock. 1994. An outbreak of ILT in California broilers. Avian Dis. 38:188-92.
Liu M, Wood JM, Ellis T, Krauss S, Seiler P, Johnson C, Hoffmann E, Humberd J, Hulse D, Zhang Y, Webster RG and Perez DR (2003). Preparation of a standardized, efficacious agricultural H5N3 vaccine by reverse genetics. Virol. 314: 580-90.
Lombardo, E., Maraver, A., Caston, J. R., Rivera, J., Fernandez-Arias, A., Serrano, A., Carrascosa, J. L., and Rodriguez, J. F. 1999. VP1, the putative RNA-dependent RNA polymerase of infectious bursal disease virus, forms a complex with the capsid protein VP3, leading to efficient encapsidation into virus-like particles. J. Virol. 73:6973-83.
Luschow D., Werner O., Mettenleiter T.C. and Fuchs W. 2001. Protection of chickens from lethal avian influenza A virus infection by live-virus vaccination with infectious laryngotracheitis virus recombinants expressing the hemagglutinin (H5) gene. Vaccine 19: 4249-59.
Malone, O., G., E. Benson, B. Alphin, and N. Tablante. 2006. Carcass disposal options and experiences for emergency disease depopulation. Proc. Natl. Mtg. Poultry Health and Process. Ocean City, MD. pp. 27-31.
Mandy F.F., Nakamura T., Bergeron M., Sekiguchi K. 2001. Overview and application of suspension array technology. Clin Lab Med. 21:713-29.
Mardani, K., A.H. Noormohammadi, P. Hooper, J. Ignjatovic, and G.F. Browning. 2008. Infectious bronchitis viruses with a novel genomic organization. J. Virol. 82: 2013-24.
Mariella, R., Jr. 2002. MEMS for bioassays. Biomed. Microdevices. 4:77-87.
McFerran, J. B., McFerran, M. S., McKillop, E. R., Conner, T. J., McCracken, R. M., Collins, D. S., and Allan, G. M. 1980. Isolation and serological studies with infectious bursal disease virus from fowl, turkeys, and ducks: demonstration of a second serotype. Avian Path. 9:384-92.
Montgomery, R.D., D.L. Magee, J.A. Watson, S.A. Hubbard, F.D. Wilson, T.S. Cummings, G.L. Luna, W.R. Maslin, C.R. Sadler, and D.L. Thornton. 2007. An episode of infectious laryngotracheitis affecting Mississippi broiler-breeders and broilers in 2002-2003. Mississippi Agric. and Forestry Expt. Sta. Bull. 1160: 1-39.
Mundt, E., and V. N Vakharia. 1996. Synthetic transcripts of double-stranded birnavirus genomes are infectious. Proc. Natl. Acad. Sci. USA 93:11131-36.
Mundt, E., Beyer, J., and Muller, H. 1995. Identification of a novel viral protein in infectious bursal disease virus-infected cells. J. Gen. Virol. 76:437-43.
Mundt, E., de Haas, N., and van Loon, A. A. 2003. Development of a vaccine for immunization against classical as well as variant strains of infectious bursal disease virus using reverse genetics. Vaccine 21:4616-24.
Neumann G., Watanabe T., Ito H., Watanabe S., Goto H., Gao P., Hughes M., Perez D.R., Donis R., Hoffmann E, Hobom G. and Kawaoka Y. 1999. Generation of influenza A viruses entirely from cloned cDNAs. Proc. Natl. Acad. Sci. USA 96: 9345-50.
Nolan, L. K. 2003. Colibacillosis. In: Diseases of Poultry, 11th ed. Y. M. Saif et al. Iowa State University Press, Ames Iowa. pp.149-50.
O. C. Ou, J. J. Giambrone, and K. Macklin. 2008. Detection of Infectious laryngotracheitis virus of the darkling beetle and its larval stage the lesser meal worm by real time PCR. Southern Conf. Avian Dis. Atlanta, GA.
Oldoni, I., and M. García. 2007. Characterization of infectious laryngotracheitis virus isolates from the United States by polymerase chain reaction and restriction fragment length polymorphism of multiple genome regions. Avian Path. 36:167-76.
Panda, A., Elankumaran, S., Krishnamurthy, S., Huang, Z., and Samal, S.K. 2004. Loss of N-linked glycosylation from the Hemagglutinin-Neuraminidase protein alters virulence of Newcastle Disease Virus. J. Virol. 78:4965-75.
Pasick, J. 2004. Application of DIVA vaccines and their companion diagnostic tests to foreign animal disease eradication. Anim. Health Res. Rev. 5:257-62.
Peiris M., Yuen K.Y., Leung C.W., Chan K.H., Ip P.L., Lai R.W., Orr W.K. and Shortridge K.F. 1999. Human infection with influenza H9N2. Lancet 354:916-17.
Pereda A.J., Uhart M., Perez A.A., Zaccagnini M.E., La Sala L., Decarre J., Goijman A., Solari L., Suarez R., Craig M.I., Vagnozzi A., Rimondi A., König G., Terrera M.V., Kaloghlian A., Song H., Sorrell E.M., Perez D.R.. Avian influenza virus isolated in wild waterfowl in Argentina: Evidence of a potentially unique phylogenetic lineage in South America. 2008. Virol. Sep 1;378:363-70. Epub 2008 Jul 15. PMID: 18632129 [PubMed - in process]
Peters, M. A., Lin, T. L., and Wu, C. C. 2005. Infectious bursal disease virus recovery from Vero cells transfected with RNA transcripts is enhanced by expression of the structural proteins in trans. Arch. Virol. 150: 2183-2194,
Peters, M. A., Lin, T. L., and Wu, C. C. 2005. Real-time PCR differentiation and quantitation of infectious bursal disease virus strains. J. Virol. Meth. 127: 87-95.
Peters, M., Lin, T. L., and Wu, C. C. 2004. Infectious bursal disease virus polyprotein expression arrests growth and mitogenic stimulation of B lymphocytes. Arch. Virol. 149:2413-26
Quan P.L., Palacios G., Jabado O.J., Conlan S., Hirschberg D.L., Pozo F., Jack P.J., Cisterna D., Renwick N., Hui J., Drysdale A., Amos-Ritchie R., Baumeister E., Savy V., Lager K.M., Richt J.A., Boyle D.B., García-Sastre A., Casas I., Perez-Breña P., Briese T., Lipkin W.I. 2007. Detection of respiratory viruses and subtype identification of influenza A viruses by GreeneChipResp oligonucleotide microarray. J. Clin. Microbiol. 45:2359-64.
Ricks C.A., Avakian A., Bryan T., Gildersleeve R., Haddad E., Ilich R., King S., Murray L., Phelps P., Poston R., Whitfill C. and Williams C. 1999. In ovo vaccination technology. Adv. Vet Med. 41:495-515.
Ritter, G.D. 2000. Clinical perspective of laryngotracheitis. Proc. 35th Natl. Mtg. Poultry Health Process. Ocean City, MD, pp. 59-60.
Ritter, G.D. 2007. LT: Biosecurity and control programs. Proc. 2007 Delmarva Breeder Hatchery Grow-out Conf., Salisbury, MD.
Robertson, G.M. and J.R. Egerton. 1981. Replication of infectious laryngotracheitis virus in chickens following vaccination. Aust. Vet. J. 57:119-23.
Rout, S. and Samal, S.K. 2008. The large polymerase (L) protein is associated with virulence of Newcastle disease virus. J. Virol. 82:7828-36.
Ruby, T., Whittaker, C., Withers, D. R., Chelbi-Alix., M. K., Morin, V., Oudin, A., Young, J. R., and Zoorob, R. 2006. Transcriptional profiling reveals a possible role for the timing of the inflammatory response in determining susceptibility to a viral infection. J. Virol. 80:9207-16.
Schlesinger S. and Dubensky T.W. 1999. Alphavirus vectors for gene expression and vaccines. Curr. Opinion in Biotech. 10:434-439.
Schultz-Cherry S, Dybing JK, Davis NL, Williamson C, Suarez DL, Johnston R and Perdue ML (2000). Influenza virus (A/HK/156/97) hemagglutinin expressed by an alphavirus replicon system protects chickens against lethal infection with Hong Kong-origin H5N1 viruses. Virol. 278:55-59.
Skyberg, J. A., T. J. Johnson, and L. K. Nolan. 2008. Mutational and transcriptional analyses of an avian pathogenic Escherichia coli ColV plasmid. BMC Microbiol. 29;8:24.
Sijben, J. W. C., Klasing, K. C., Schrama, J. W., Parmentier, H. K., van der Poel, J. J., Savelkoul, H. F. J., and Kaiser, P. 2003. Early in vivo cytokine genes expression in chickens after challenge with Salmonella typhimurium lipopolysaccharide and modulation by dietary n3 polyunsaturated fatty acids. Developmental Comp. Immunol. 27:611-619.
Snyder, D. B., Lana, D. P., Savage, P. K., Yancey, F. S., Mengel, S. A., and Marquardt, W. W. 1988. Differentiation of infectious bursal disease viruses directly from infected tissues with neutralizing monoclonal antibodies: evidence of a major antigenic shift in recent field isolates. Avian Dis. 32:535-539.
Solorzano A, Song H, Hickman D, Pérez DR. Pandemic influenza: preventing the emergence of novel strains and countermeasures to ameliorate its effects. 2007. Infect Disord Drug Targets. 7:304-17. Review. PMID: 18220962 [PubMed - indexed for MEDLINE]
Solorzano, A., R. J. Webby, K.M. Lager, B.H Janke, A. Garcia-Sastre, and J.A. Richt. 2005. Mutations in the NS1 Protein of Swine Influenza Virus Impair Anti-Interferon Activity and Confer Attenuation in Pigs. J. Virol. 79:7535-43.
Song H., Nieto G.R., Perez D.R. 2007. A new generation of modified live-attenuated avian influenza viruses using a two-strategy combination as potential vaccine candidates. J. Virol. Jun 27; [Epub ahead of print]
Song, H. R.G. Nieto, R.D. Perez. 2007. A New Generation of Modified Live- Attenuated avian influenza viruses Using a two-strategy combination as potential vaccine candidates. J. Virol. 81:9238-48
Song, M, T.K. Oh, H.J. Moon, D.W. Yoo, E.H. Lee, J.S. Lee, C.J. Kim, G.J Yoo, H. Kim & Y.K. Choi. 2008. Ecology of H3 avian influenza viruses in Korea and assessment of their pathogenic potentials. J. Gen. Virol. 89:949-57.
Sorrell E.M., Perez D.R. 2007. Adaptation of influenza A/Mallard/Potsdam/178-4/83 H2N2 virus in Japanese quail leads to infection and transmission in chickens. Avian Dis. 51(1 Suppl):264-8.
Sorrell E.M., Ramirez-Nieto G.C., Gomez-Osorio I.G., Perez D.R. 2007. Genesis of pandemic influenza. Cytogenetic Genome Res.117(1-4):394-402.
Spackman E., Senne D.A., Davison S., Suarez D.L. 2003. Sequence analysis of recent H7 avian influenza viruses associated with three different outbreaks in commercial poultry in the United States. J. Virol. 77: 13399-13402.
Spackman, E., D. A. Senne, T. J. Myers, L. L. Bulaga, L. P. Garber, M. L. Perdue, K. Lohman, L. T. Daum, and D. L. Suarez. 2002. Development of a real-time reverse transcriptase PCR assay for type A influenza virus and the avian H5 and H7 hemagglutinin subtypes. J. Clin. Microbiol. 40:3256-3260.
Stewart-Brown, B. 2006. Overview of ILT outbreaks in the United States. Proc. 41st Natl. Mtg. Poultry Health Proc. Ocean City, MD, pp. 78-80.
Subbarao K., Klimov A., Katz J. 1998. Characterization of an avian influenza A (H5N1) virus isolated from a child with a fatal respiratory illness. Science 279: 393-96.
Sun, H., Y. Wang, G. Tong, P. Zhang, D. Miao, H. Zhi, M. Wang, and M. Wang. 2008. Protection of chickens from Newcastle disease and infectious laryngotracheitis with a recombinant fowlpox virus co-expressing the F, HN genes of Newcastle disease virus and gB gene of infectious laryngotracheitis virus. Avian Dis. Digest, Vol. 3, No. 1, pp. 10.
Swayne D.E., Beck J.R., Garcia M., Stone H.D. 1999. Influence of virus strain and antigen mass on efficacy of H5 avian influenza inactivated vaccines. Avian Path. 28:245-56.
Swayne D.E., Senne D.A., Beard C.W. 1998. Avian influenza. In:D. E. Swayne (ed.), A Laboratory Manual for the Isolation and Identification of Avian Pathogens. Amer. Assn. Avian Pathologists, Kennett Square, PA pp. 150-155.
Swayne, D. E. and D. A. Halverson. 2003. Influenza. In: Diseases of Poultry, 11th ed. Y. M. Saif et al. Iowa State University Press, Ames, IA. pp.149-150.
Tang Y., Lee C.W., Zhang Y., Senne D.A., Dearth R., Byrum B., Perez D.R., Suarez D.L., Saif Y.M. 2005. Isolation and characterization of H3N2 influenza A virus from turkeys. Avian Dis. 49:207-13.
Taylor, J. W., J. Ott, F. Eckstein. 1985. The rapid generation of oligonucleotide-directed mutation at high frequency using phosphorothioate-modified DNA. Nucleic Acid Res: 13:8765-85.
Toro H, Tang DC, Suarez DL, Zhang J, Shi Z. 2008. Protection of chickens against avian influenza with non-replicating adenovirus-vectored vaccine. Vaccine. 26:2640-46.
Toro, H. and D. Suarez. 2007. 50th Ann. Mtg. Amer. Assn. Avian Pathologists Washington, DC.
Tripathy, D.N. 1998. Infectious laryngotracheitis. In: D.E. Swayne, J.R. Glisson, M.W. Jackwood, J.E. Pearson, and W.M. Reed eds. A Laboratory Manual for the Isolation and Identification of Avian Pathogens, 4th ed. Amer. Assn. Avian Pathologists, Kennett Square, PA, pp. 111-15.
Vakharia, V.N., He, J., Ahamed, B., and Snyder, D. B. 1994. Molecular basis of antigenic variation in infectious bursal disease virus. Virus Res. 31:265-73.
Van den Berg, T. P., Eterradossi, N., Toquinm D., and Meulemans, G. Infectious bursal disease (Gumboro disease). 2000. Rev. Sci. Tech. 19:509-543.
Wan H., Perez D.R. Amino acid 226 in the hemagglutinin of H9N2 influenza viruses determines cell tropism and replication in human airway epithelial cells. 2007. J. Virol. 81:5181-91. Epub 2007 Mar 7.
Wander, W, and P. Dunne. 1981. Species and numbers of shorebirds on the Delaware Bay shore of New Jersey - Spring 1981. Occas. Paper No. 140. Records New Jersey Birds 7:59-64.
Wang L., Suarez D.L., Pantin-Jackwood M., Mibayashi M., García-Sastre A., Saif Y.M., Lee C.W. 2008. Characterization of influenza virus variants with different sizes of the non-structural (NS) genes and their potential as a live influenza vaccine in poultry. Vaccine. 26:3580-86.
Webby R.J., Woolcock P.R., Krauss S.L., Walker D.B., Chin P.S., Shortridge K.F., Webster R.G. 2003. Multiple genotypes of nonpathogenic H6N2 influenza viruses isolated from chickens in California. Avian Dis. 47(3 Suppl):905-10.
Webster R.G., W.J. Bean, O.T. Gorman, T.M. Chambers and Y. Kawaoka. 1992. Evolution and ecology of influenza A viruses. Microbiol. Rev. 56:152-79.
Winterfield, R. W., S. B. Hitchner, and G. S. Appleton. 1964. Immunological characteristics of a variant of infectious bronchitis virus isolated from chickens. Avian Dis. 8: 40-47.
Wu, C. C, and Lin, T. L. 1992. Detection of infectious bursal disease virus in digested paraffin-embedded histologic sections by polymerase chain reaction. J. Vet. Diagn. Invest. 4:452-55.
Wu, C. C., Lin, T. L., and Akin, A. 1997. Quantitative competitive polymerase chain reaction for detection and quantitation of infectious bursal disease virus RNA and cDNA. J. Virol. Meth. 66:29-38.
Wu, C. C., Lin, T. L., Zhang, H. G., Davis, V. S., and Boyle, J. A. 1992. Molecular detection of infectious bursal disease virus by polymerase chain reaction. Avian Dis. 36:221-26.
Wu, C. C., Rubinelli, P., and Lin, T. L. 2007. Invited Minireview: Molecular detection and differentiation of infectious bursal disease virus. Avian Dis. 51:515-26.
Wu, H., Y. Williams, K. S. Gunn, N. K. Singh, Locy, R. D., and J. J. Giambrone. 2004. Yeast derived sigma C protein-induced immunity against Avian Reoviruses. Avian Dis. 49:281-84.
Yan, Y. and Samal, S.K. 2007. Role of intergenic sequences in Newcastle disease virus RNA transcription and pathogenesis. J. Virol. 82:1323-31.
Yao, K., Goodwin, M. A., and Vakharia, V. N. 1998. Generation of a mutant infectious bursal disease virus that does not cause bursal lesions. J. Virol. 72:2647-54.
Yassine H.M., Al-Natour M.Q., Lee C.W., Saif Y.M. 2007. Interspecies and intraspecies transmission of triple reassortant H3N2 influenza A viruses. Virology J. 4:129.
Yassine H.M., Lee C.W., Suarez D.L., Saif Y.M. 2008. Genetic and Antigenic Relatedness of H3 Subtype Influenza A Viruses Isolated from Avian and Mammalian Species. Vaccine. 26:966-977.
Yu, Q., Estevez, C. 2006. Sequence analysis of the complete genome of avian metapneumovirus Subgroup C Colorado strain: development of a reverse genetics system for this virus. In: Proc. Inter. Symp. Avian Corona and Pneumoviruses and Complicating Pathogens, Rauischholzhausen, Germany. pp. 6-15.
Ziegler, A. F., B. S. Ladman, P. A. Dunn, A. Schneider, S. Davison, P. G. Miller, H. Lu, D. Weinstock, M. Salem, R. J. Eckroade, and J. Gelb, Jr. 2002. Nephropathogenic infectious bronchitis in Pennsylvania chickens 1997-2000. Avian Dis. 46:847-858.