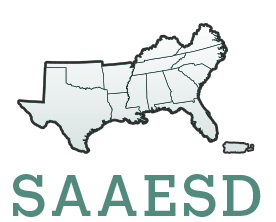
S1048: Assessment of the Carbon Sequestration Potential of Common Agricultural Systems on Benchmark Soils Across the Southern Region Climate Gradie
(Multistate Research Project)
Status: Inactive/Terminating
S1048: Assessment of the Carbon Sequestration Potential of Common Agricultural Systems on Benchmark Soils Across the Southern Region Climate Gradie
Duration: 07/01/2011 to 09/30/2016
Administrative Advisor(s):
NIFA Reps:
Non-Technical Summary
Statement of Issues and Justification
Problem Statement
With the recent advent of the Chicago Climate Exchange (CCX, 2006), soil carbon (C) credits, which can be accrued by the agricultural community through the use of conservation-tillage and grassland restoration practices, can be bought and sold in a stock-exchange-like market (Schneider and McCarl, 2003) to off-set carbon dioxide (CO2) emissions to the atmosphere from large companies and utilities. The agricultural community has a competitive advantage in the carbon economy because agricultural soils, if properly managed, can sequester appreciable amounts of atmospheric C as soil organic carbon (SOC). Conversion from conventional- to conservation-tillage practices provides a management mechanism for increasing soil carbon stocks. Marketing C credits can represent an added economic incentive to producers to continue using or convert to more environmentally friendly and sustainable agricultural management practices. Additionally, perennial biomass energy crops, many of which are grasses and may be a future focus of US producers, also have the potential to sequester SOC, adding an economic dimension to dedicated energy crops (Lemus and Lal, 2005; Liebig et al., 2005; Omonode and Vyn, 2006; Tillman and Lehman 2006). For the carbon economy to be politically-, legislatively-, and economically-viable, science-based methods for monitoring and verifying SOC changes must be accurate, sensitive, and practical. However, soil C sequestration rates have been developed for C credit accrual in upper-mid-western states, but not for southern states.
In a study conducted throughout Canada, VandenBygaart et al. (2003) documented that C sequestration potential was generally greater for soils with a low SOC pool. Similarly, southern soils (i.e., Alfisols and Ultisols), particularly those of the Mississippi River Delta region and other areas throughout the southern US, may have a greater potential for C sequestration than upper-mid-western soils (i.e., Alfisols and Mollisols) due to the generally lower soil organic matter (OM)/SOC contents. However, southern US soils may also respond differently to imposed management practices than Canadian soils. Considering the economic component to soil C sequestration, if C credits for the southern US are set based on the soil C sequestration potential of upper-mid-western soils, producers will likely not be fully economically compensated for their conservation practices if soil C sequestration potentials are indeed greater in the southern US than in the upper mid-west.
In addition to initial SOC level, soil C sequestration depends on soil texture (Ihori et al., 1995; Percival et al., 2000; Brye and Kucharik, 2003), landuse or agricultural management system (West and Post, 2002) and time (i.e., consistent duration of current landuse or agricultural management system; Potter et al., 1999; Brye et al., 2002a; Brye and Kucharik, 2003; Tolbert et al., 2002; Post et al., 2004). Therefore, it is essential to develop appropriate soil C sequestration potentials for southern soils, across varying soil textures and cropping systems, so that agricultural producers can maximize economic benefits for employing more-sustainable, conservation-tillage practices and contribute to decreasing the rising atmospheric CO2 concentrations.
Furthermore, momentum is currently increasing to find alternative sources of fuel. Perennial crops that produce a large amount of above-ground biomass are being targeted as one potential bio-fuel source that could be dedicated for energy production by combustion. Though it has been shown that perennial bio-fuel crops, particularly the grasses, will increase soil C storage (Tolbert et al., 2002), little to no data exists demonstrating the soil C sequestration potential associated with perennial bio-fuel crops in the soils of the southern US.
Justification
The scientific community knows several important things about the Earths atmosphere and how it has changed in the last century or so: 1) atmospheric CO2 concentrations, as well as concentrations of other greenhouse gases, where greenhouse gases are those [i.e., CO2, methane (CH4), ozone (O3), nitrous oxides (NOx), and water (H2O)] that tend to absorb radiant heat energy and trap it within the Earths atmosphere before that heat energy escapes to space, are increasing; 2) as a result of increased greenhouse gas concentrations, the entire Earth has experienced a warming trend of between 0.5 and 1.7 oF in the last 100 years (USEPA, 2006); 3) human activity, particularly the burning of fossil fuels among other mechanisms, is changing the composition of the Earths atmosphere and exacerbating the greenhouse effect and global warming (IPCC, 2001; USEPA, 2006). Other significant mechanisms affecting the composition of Earths atmosphere include land-use change and agriculture.
Some serious ramifications exist if increasing greenhouse gas concentrations and global warming continue. A potential positive impact might be increased photosynthetic rates and productivity from some plant species in the presence of an atmosphere with a greater CO2 concentration. However, the potential negative consequences are far more numerous and include the partial melting of ice caps, flooding of coastal lands, major disruptions of weather patterns, which some may argue are occurring already, lower agricultural yields, species extinctions, and a proliferation/expansion of disease vectors, among others. Though some is already known about what is happening and what might happen if trends continue at current rates, many questions remain regarding the specific effects of land-use change and other human activities (e.g., agriculture) on the Earths climate that will require much further scientific exploration (USEPA, 2006).
Although not as extensive as the burning of fossil fuels, agricultures role with increasing greenhouse gas concentrations is significant given the amount of arable land present in some states and throughout the contiguous US. Animal agriculture is responsible for CO2 and CH4 emissions, while cultivated row-crop agriculture as a specific land use is responsible for CO2 (i.e., soil respiration), CH4 [i.e., methanogenesis from anaerobic soil conditions generally associated with rice (Oryza sativa L.) production], and NOx (i.e., denitrification from water-logged or prolonged saturated soil conditions) emissions. Although CH4 is ~ 30 times more effective than CO2 at trapping heat energy in the atmosphere, CO2 emissions to the atmosphere from soil respiration are much more widespread and temporally consistent than agriculturally related CH4 emissions.
Soil respiration is the combination of root and microorganism respiration, both of which are affected by soil moisture and temperature conditions. In the context of row-crop agriculture, cultivation (i.e., tillage), as a common residue and soil management practice, loosens and aerates the soil, at least temporarily, and tends to stimulate the oxidation of soil OM, hence increasing soil respiration (Doran, 1980; Fortin et al., 1996; Reicosky 1997; Curtin et al., 2000). This general relationship between tillage and soil surface CO2 flux (i.e., soil respiration) has also been demonstrated for silt-loam Alfisols in the Mississippi River Delta region of eastern Arkansas, where soil surface CO2 fluxes over a 2-year period were 38 % greater under conventional tillage (CT) than under no-tillage (NT) in a wheat (Triticum aestivum L.)-soybean [Glycine max (L.) Merrill] double-cropped production system (Brye et al., 2006a). Therefore, it is certainly possible to reduce CO2 emissions to the atmosphere and increase C in the soil simultaneously within row-crop agriculture simply by altering residue and general soil management strategies.
The build-up or accrual of C from the atmosphere can occur in soil, biomass (i.e., forests), and oceans and is known in general as carbon sequestration. Since soil is estimated to contain nearly double the amount of OC contained in the atmosphere (Schlesinger, 2000), it is believed that soil can act as a significant C sink because of soils known responsiveness to modification (i.e., various residue and general soil management strategies; Baker et al., 2007). Lal et al. (2003) projected soil C sequestration rates of 24 to 40 Mt C yr-1 if widespread adoption of conservation-tillage practices, generally defined as any tillage practice that leaves enough crop residue to cover 30 % of the soil surface, occurred throughout the US. In a global analysis based on 67 long-term experiments, West and Post (2002) reported that 57 (" 14) g C m-2 yr-1 could be sequestered in the soil by conversion from CT to NT. Similarly, Post and Kwon (2000) reported a mean global C sequestration rate of 33.2 g C m-2 yr-1 upon conversion from agriculture to grasslands. In contrast, VandenBygaart et al. (2003) also reported SOC increases following conversion to no-tillage, but only for western Canada, while similar land-use conversion in eastern Canada had no effect on SOC accretion. Due to the variable spatial results reported in the literature, a comprehensive survey throughout the southern US climate gradient is warranted.
As an ecosystem function, soil C sequestration generally does not continuously increase. Soil C sequestration rates have been shown to decrease over time (Silver et al., 2000). West and Post (2002) estimated that peak C sequestration rates would be achieved within 5 to 10 years upon conversion from CT to NT and that a new equilibrium soil C concentration would be achieved within 15 to 20 years with little soil C increase thereafter. Soil C sequestration potential has also been linked to general soil taxonomy and the size of the SOC pool. VandenBygaart et al. (2003) demonstrated that soil C sequestration potential decreased as the SOC pool increased. This may be particularly significant throughout much of the southern US which has rather low levels of SOC due to a long history of cultivation. Brye et al. (2006b) documented a 2-fold greater increase in SOC content under NT than CT over a 2-year period in a wheat-soybean double-cropped production system in east-central Arkansas, which translated into sequestration rates of 84.5 and 162 g SOC m-2 yr-1 in the top 10 cm of soil. In contrast, an undisturbed native tallgrass prairie on a silt-loam Alfisol in east-central Arkansas sequestered 45.7 g SOC m-2 yr-1 in the top 10 cm over a 14-yr period from 1987 to 2001 (Brye et al., 2004b). These few assessments of soil C sequestration in eastern Arkansas support the conclusions of VandenBygaart et al. (2003) and demonstrate that soil C sequestration potential is greater for soils with a low SOC pool, which characterizes many of the soils located throughout much of the southern US. In addition, in a 2008 survey of soils in the Suwannee River watershed in GA, SOC values for the top 15 cm ranged from 0.39 to 2.52% C with an average of 0.79% for both conventionally-tilled and no-tillage managed farms. Assuming that the low-SOC soils could be increased to the high-SOC values as a result of conservation tillage, a maximum potential SOC increase of 550% (or 5354 g m-2) could be realized. A more conservative estimate using the average SOC value would suggest a potential increase of 104% (or 1012 g m-2). Thus, southern US soils may have a greater potential for soil C sequestration than upper-mid-western soils due to the generally lower soil OM/OC contents. Soil C sequestration will also likely be greatly impacted by the current bio-fuel/bio-energy initiative.
A steady land-use change in the agricultural sector from traditional food/fiber crops toward dedicated bio-energy crops is foreseeable. Whereas the US energy security remains a major concern, other important issues such as soil C sequestration and climate mitigation add new immediacy to conversion of traditional crops to bio-fuel crops (Walsh et al., 2003). Politicians and economists advocate capitalizing on emission and sequestration of greenhouse gases in the form of a C-trading market (Freibauer et al., 2004). Because bio-energy crops will enhance the potential of SOC accumulation (McCarl and Schneider, 2001; Tilman et al., 2006), the projected C credit, estimated from $5 to more than $20 per metric ton (Causarano et al., 2006), will contribute a new dimension to the economic viability of bio-energy crops and farm incomes. The problem is that there is little scientific knowledge regarding the potential expected change in SOC when a soil is converted from a row crop or grazing pasture to a perennial bio-fuel crop. The information that is available focuses on native grasses [i.e., switchgrass (Panicum virgatum L.] and some perennial bio-fuel stocks (i.e., Miscanthus). To confound the lack of information, SOC sequestration data for the southern US are minimal, and few of the many SOC sequestration studies in the literature address the variable impact of climate and soil properties on SOC sequestration potential.
Though many soil responses to a particular treatment are easily quantifiable within a relatively short period of time, to be accurately assessable, the ecosystem function of soil C sequestration requires more than just one or two growing seasons. Inter-annual variability of soil C is often larger than the actual increases (or decreases) in soil C from year to year. Thus, accurate assessments of soil C sequestration will require more than 1 or 2 years between samplings.
Broader Project Impact
This proposal outlines a multi-state, regional project that is designed to improve our understanding and knowledge regarding the complex relationships among climates, soils, land uses, and C. The specific objective is to assess the soil carbon sequestration potential of common agricultural and natural ecosystems of varying ages on benchmark soils across the southern region climate gradient. This objective can be achieved relatively easily in the context of current research activities being conducted by all participating individuals despite not all individuals presently working on soil C sequestration or the bio-fuel issue. This project will generate essential, scientifically based field data to support accurate projections of soil C sequestration potentials across the climate gradient of the southern US. The project will build on published research by the regional soil physics group (S-124, S-185, S-225, and S-257) characterizing the physical properties of southern benchmark soils (Dane et al., 1983; Nofziger et al., 1983; Quisenberry et al., 1987; Romkens et al., 1986; Romkens et al., 1985; Bruce et al., 1983; Cassel, 1985; Scott, 1999). This project will also represent the first multi-state regional project of its kind throughout the southern US integrating research from Texas to Florida. Aside from the USDA-ARS GRACEnet Project led by Dr. Ron Follett, the only other similar multi-state regional project, NC1017 entitled Carbon Sequestration and Distribution in Soils of Eroded Landscapes , is in the north-central US including participating states of IL, IN, IA, KS, MN, MO, ND, OH, SD, and WI and the USDA-ARS National Soil Tilth Laboratory.
The Southern Association of Agricultural Experiment Station Directors has a current list of 33 Priorities for Multistate Research Activities. The proposed Regional Project will address aspects of eight (8) of these priority areas including: 1) Multiple uses of agricultural lands, 2) Environmentally benign agricultural operations, 3) Nutrient management in agricultural systems, 4) Air, soil, and water resources conservation and enhancement, 5) Natural resource and ecosystem management, 6) Environmental policy and regulations, 7) Integrated and sustainable agricultural production systems, and 8) Bioenergy and alternative fuels from agricultural products.
Related, Current and Previous Work
The southern regional soil physics research committee was formed some time in the late 1970's. The original goal of the group was to serve as a scientific meeting for soil physicists in the south. Historically, the southern states did not have many soil physicists. Dr. Russell Bruce, with USDA-ARS at Watkinsville, GA was one of the primary founders of the group.
The group has had four formal, southern regional projects, S-124, S-185, S-225, and S-257, from the late 1970's through 1999. Dr. George J. Kriz was the administrative advisor through these four regional projects.
The first project, S-124 entitled Movement and retention of water and solutes in selected Southern Region field soils, received funding as a field-scale regional project with a common design, but was narrow in scope in that it quantified hydraulic properties for major soil series in the south-eastern US. Emphasis was placed on collecting data and evaluating its geographical variability. Resulting soil hydraulic property data were published in eight Regional Research Bulletins and these data formed the framework for an international database of soil hydraulic properties developed by personnel at the US Salinity Laboratory.
The second project, S-185 entitled Spatial and temporal variability of soil characteristics and material fluxes in field soils", expanded the soil hydraulic property database and emphasized the description and prediction of the observed variability using geostatistical procedures, such as variogram analysis, auto-correlation analysis, kriging, sliding polynomials, spectral analysis, and bootstrapping.
The third project, S-225 entitled "Variability of soil properties and its effect or water quality and soil management", focused on the effect of the soil properties and their variability on practical problems. This project stressed the effects of soil properties and their associated short-, medium-, and long-range variability on water movement and solute transport. The S-225 project also evaluated the interrelationships between soil variability and soil management systems.
The S-257 project, entitled Classifying soils for solute transport as affected by soil properties and landscape position, was the last formal regional project. The group then changed to an Information Exchange Group (IEG). The book "Water and Chemical Transport in Soils of the Southeastern United States", H. Don Scott, Editor, was written by members of the group between 1997 and 1998 and published in December 1999 as Special Report 197 of the Arkansas Agricultural Experiment Station.
The group historically, even continuing from 1999 through 2008, has met each year at the home location of the current secretary. The meeting format included detailed presentations by each scientist on recently completed or on-going studies. There was considerable discussion among the scientists during each presentation. When possible, the meeting would include a field trip to an on-going research site. The host scientist has always had the participants to his or her home one evening for dinner to promote camaraderie among the group members and guests (i.e., undergraduate or graduate students and post-docs of the group members).
Need for a New Direction
The previous four regional projects that spanned nearly three decades were generally centered around characterizing soil physical and hydraulic properties at various positions in a landscape to assess spatial variability and their influence on solute transport. Throughout the 1970's through 1990's, many models had been or were being developed that relied on databases of measured soil physical and hydraulic properties across a wide range of soils to make reasonably accurate predictions about the fate and transport of a variety of potential environmental contaminants. However, the research needs and environmental issues that existed 10 to 30 years ago, are not the same needs and issues that exist presently.
Mitigation of rising atmospheric CO2 concentrations and identifying alternative energy sources are two of the major environmental, political, and social issues present today. Agriculture and agricultural activities are central to the potential success that we might have at addressing these two global issues. Specifically, the soil C sequestration potential will directly impact the magnitude of change that can be expected or realized when addressing these two issues. In addition, both issues have a significant economic component to them with individual agricultural producers potentially benefiting financially through employing environmentally sound land management practices. Carbon will likely be increasingly viewed as a marketable commodity as interest and direct involvement in C-credit trading increases. For these reasons, researchers throughout the southern region feel that a new direction to unified research efforts is necessary.
Soil OM and C are related to a wide range of other soil properties and processes, thus soil OM and C are important factors to be considered in most research activities soil physicists undertake. Since OM is the native source of soil nutrients, SOM and C are important for maintaining the soils natural fertility, which in turn affects soil physical properties and biological activity for sustainable crop production (Follet et al., 2001). Soil organic matter helps to minimize compaction, reduce soil erosion (Rhoton, 2000), and improves soil workability, water-use efficiency, resistance to surface crusting, and nutrient cycling and availability for adequate plant growth (Weil and Magdoff, 2004). Soils with low levels of SOM generally require greater levels of inorganic fertilizers, irrigation water, pesticides and machinery inputs than soils with adequate SOM to maintain the same crop yield (Magdoff and van Es, 2000). Crop response to mineral inputs is generally increased in soils with greater SOM due to the positive effects of SOM on soil biological and physicochemical properties (Wander, 2004). The quality of water flowing from catchments depends on the sorptive capacity of the soil, which depends on SOM (Oades, 1995). Soil OM and C are also related to other soil physical properties such as soil structure and aggregation (Undersander and Reiger, 1985; Six et al., 2004); soil hydraulic properties such as soil moisture, water-holding capacity, infiltration capacity, and hydraulic conductivity (Undersander and Reiger, 1985; Rawls et al., 2003); soil chemical properties such as cation-exchange capacity (Fontana et al., 2008); and soil biological properties such as soil respiration (Brye et al., 2006a), microorganisms (Six et al., 2004), and biological diversity (Collins et al., 1992). Therefore, SOM and C clearly affect or are related to a vast array of other soil properties and processes that many soil physicists may be currently researching. The present timeliness of the bio-energy and rising greenhouse gas issues provides the necessary opportunity to expand research activities into new, but related, arenas that can have immediate and direct positive impacts on these major environmental issues.
Current Work
Selected research is already being conducted across the southern climate/soil gradient in Texas, Arkansas, Tennessee, and Georgia investigating the soil C sequestration potential of various conservation-tillage practices and perennial bio-fuel crops.
In Texas, a current study is being conducted by Dr. Cristine Morgan to evaluate the soil C sequestration potential of Arundo donax L., a perennial grass that is a candidate as a cellulosic bio-fuel source. Two of the project objectives include comparing C sequestration potential of bermudagrass (Cynodon dactylon), which has a soil C sequestration value associated with it at the CCX, to Arundo donax and quantifying the spatial variability of SOC in a floodplain to understand how landscape position affects soil C estimates. An 80-ha field that has both Arundo and bermudagrass established for over 60 years has been soil sampled for total and inorganic soil C. In all, 125 soil cores, 78 under Arundo and 47 under bermudagrass, were collected to a depth of 60 cm. For each 10-cm depth increment of each core, bulk density, total C (Nelson and Sommers 1996), and inorganic carbon (Sherrod et al., 2002) are being measured. Soil OC is determined by subtracting inorganic C from total C and using the measured bulk density to convert SOC to a mass-equivalent basis (Mg ha-1). Preliminary results show that Arundo has greater SOC in the 0- to 10-cm (P < 0.01) and 10- to 20-cm (P < 0.05) depth intervals than bermudagrass, while SOC is similar in the 40- to 50-cm depth interval. The average Arundo SOC in the 0- to 10-cm depth is 16.9 Mg ha-1 and that for bermudagrass is 11.6 Mg ha-1. The study is continuing to investigate spatial variability of SOC under the two grasses as well. New Arundo plots have also been recently established in a different floodplain on a more clayey soils and under a different rainfall regime.
In Arkansas, recent and on-going research by Dr. Kristofor R. Brye has centered around assessing native soil quality and the effects of agricultural practices on soil quality with emphasis on soil C sequestration potential. Before research could begin on evaluating the effects of agricultural practices on soil quality in specific cropping systems, the present level or status of soil quality in representative, undisturbed ecosystems had to be characterized and understood. Therefore, native prairies scattered throughout the Mississippi River Delta region of eastern Arkansas and the Ozark Highlands of northwest Arkansas were initially targeted for soil quality assessments along with adjacent areas that had been disturbed by either mechanized agriculture or pasture management practices, such as animal grazing and forage removal. Initially (i.e., prior to 2003), soil quality assessments in the top 10 cm were conducted on three sites in the Ozarks and eight sites in the Delta, representing 10 and > 36 soil-mapping-unit/land-use combinations, respectively. The long-term goal was to assess how native soil quality changes over time and how other soil disturbances affect soil quality over time, specifically management practices affect the soils ability to sequester carbon. Recently (i.e., after Fall 2007), the same initial sites were re-assessed for changes that may have taken place in the previous 5-6 year period since initial assessment. Additional sites have also been added to the Ozarks regions and all new and re-sampled sites have been sampled from the 10-20 cm depth interval as well to investigate deeper-profile changes that may be occurring. One important research result thus far has been that it appears that soil C sequestration in Arkansas soils and under the present climate regime may be greater than once thought.
Additional recent and on-going research in Arkansas has focused on investigating the long-term impacts of alternative wheat (Triticum aestivum L.) residue management practices [i.e., conventional- (CT) and no-tillage (NT), burning and no burning, and low and high wheat-residue levels achieved with differential N fertilization) on soil quality and soybean [Glycine max (L.) Merr.] production. A long-term field study on a common silt-loam soil in the Mississippi River Delta region of eastern Arkansas was initiated in Fall 2001 with all combinations of the above-mentioned treatments. Treatments were arranged in a strip-split plot experimental design and replicated six times. Time trends for the first six years of this long-term rotation study indicate that soil organic matter (SOM) is increasing over time in all treatment combinations. Furthermore, total soil C is increasing at a greater rate in the no burn and high-residue-level than in the burn and low-residue-level treatments. These results support the contention that implementation of the appropriate residue management practices has the potential to improve soil quality and maintain long-term productivity of silt-loam soils in the Mississippi River Delta region of the mid-southern United States and likely elsewhere.
In Tennessee, a multi-disciplinary group, including scientists from soil science (Drs. Jaehoon Lee and Don Tyler), economics (Drs. Burt English and Chris Clark), and plant science (Drs. Neal Stewart and Neal Rhodes), is investigating effects of bio-crop production on soil and water quality. One of the objectives of the project is to evaluate the impacts of land-use change on properties of the local ecosystem, such as SOC, nutrients, soil structure, the soil microbial community, and soil moisture availability. Beginning in 2004, the University of Tennessee Switchgrass Project established 700 acres of switchgrass at various UT Research and Education Centers. The current study has two main scientific questions: What is the direct impact on the soil, and what are the subsequent, implied impacts on the environment? Examples of direct effects on the soil are SOC, carbon emission/sequestration, nutrient availability (i.e., will additional fertilizers be required), regulation of soil temperature, changes to soil structure (implied impacts on infiltration capacity and therefore erosion), changes in microbial habitats (what kinds of organism dwell in this environment), and soil moisture budget. Some of these propagate new questions regarding the environmental impacts of switching to a monoculture with such high production levels. Government studies as well as published studies state SOC increases with switchgrass and is one of its major benefits. However, long-term data are not plentiful and previous studies found results of a 10+ year study to be variable. Even if it were to increase C, degradation of some compounds is C-type specific (e.g., hydrophobic acids, hydrophilic C, colloidal C etc.). The collected field data are being used with watershed and crop models to estimate the effects of land-use change on water and soil quality.
In Georgia, soil C sequestration research is also being conducted at the USDA/ARS Southeast Watershed Research Laboratory at Tifton. A number of cooperative research studies are on-going under the leadership of Dr. Timothy C. Strickland. Studies combine repeated measurement of total soil C and N with microbial biomass C and N analyses. All studies address the effects of conservation tillage and/or water utilization in the Coastal Plain of Georgia. Current projects include: 1) the effects of cover crops [i.e., sunn hemp (Crotalaria juncea L.) and crimson clover (Trifolium incarnatum)] in conservation tillage on C and N sequestration and cycling and soil physical properties in sandy coastal plain soils [Dr. Robert Hubbard (USDA-ARS-Tifton) and Dr. Sharad Phatak (UGA-Tifton)], where preliminary results indicate that sunn hemp grown under conservation-tillage practices can increase soil C at a rate of 107 g m-2 yr-1; 2) the effects of composted municipal sludge in conservation tillage to increase soil C levels (Drs. Hubbard and Phatak). This study also includes effects of these systems plus C from the sludge on soil physical properties (Dr. Hubbard); 3) the effects of several different winter covers [rye (Secale cereale), rye + winter pea (Pisum sativum L.), faba bean (Vicia faba L.), and fallow] and winter cover planting dates on soil moisture availability, thermal stress to crops, and C and N cycling in conventional-tillage and strip-tillage systems [Drs. Dana Sullivan, Clint Truman, Robert Hubbard, and Brian Scully (USDA-ARS-Tifton) and Drs. Gary Hawkins, Scott Tubbs, John Beasley, and Dewey Lee (UGA-Tifton)]; 4) the regional variation of soil C and N, winter cover crop biomass, and residue coverage as detected by remote sensing platforms [Dr. Sullivan, Dr. Mark Masters (Albany State Univ.), and Drs. Guy Serbin, Dean Hively, and Greg McCarty (USDA-ARS-Beltsville, MD)]; 5) a comparison of conservation versus conventional tillage effects on C and N cycling and accretion, sediment erosion potential, erosive carbon losses in runoff, and associated pesticide degradation and facilitated transport [Drs. Truman and Hubbard, David Bosch, and Thomas Potter (USDA-ARS-Tifton) and Dr. Dorcas Franklin (USDA-ARS-Watkinsville, GA)]; 6) the effects of conservation tillage and variable irrigation control on C and N accretion, soil moisture, and crop response [Dr. Sullivan and Dr. Marshall Lamb (USDA-ARS-Dawson, GA); 7) the effect of poultry litter amendments on the production of napier grass (Pennisetum purpureum L. Schum.) as a bio-fuel feedstock and associated accretion of soil C and N and microbial C and N cycling [Drs. Robert Hubbard, Bill Anderson (USDA-ARS-Tifton) and Dr. Ravindra Malik (Albany State University)]; and 8) the effects of conservation tillage in a dry-land cropping system on within-field associations between soil C and N accretion and availability, soil moisture profile, winter cover biomass production, and summer crop yield [Drs. Clint Truman, Dana Sullivan, Robert Hubbard, Bill Anderson, Dawn Olsen, Brian Scully, Jeff Wilson (USDA-ARS-Tifton), Russell Nuti (USDA-ARS-Dawson, GA), Gary Hawkins (UGA-Tifton)].
A similar multi-state regional project to the one being proposed for the southern region is NC1017, entitled Carbon Sequestration and Distribution in Soils of Eroded Landscapes . However, NC1017 focuses on soil responses in the north-central region of the US which differs climatically, ecologically, and edaphically from the southern region. Observed soil responses in the north-central would not necessarily be similar to observed responses in the southern region. Therefore, the southern region needs to be studied separately.
Objectives
-
The soil C sequestration potential of common cropping systems and land uses, including bio-fuel crops, on benchmark soils throughout the southern US are largely unknown. Similarly, the impact of climate regime, which can have a varying effect across the southern states, on soil C sequestration potential is largely unknown. Therefore, to address these present knowledge gaps and unify present research activities in the southern and south-eastern US into a common goal, the overall objective of this regional research project is to assess the soil carbon sequestration potential of common agricultural and natural ecosystems of varying ages on benchmark soils across the southern region climate gradient. Though the main study objective will be on direct assessment of soil carbon sequestration potential, several related sub-objectives will also be explored, namely i) evaluate the effects of land use, crop rotation, tillage practice, soil texture, and ecosystem age/rotation duration on soil carbon concentration, content, and sequestration and related soil physical and chemical properties, ii) quantify and understand the physical and chemical processes that relate to and control soil carbon sequestration, and iii) investigate spatial variability issues associated with soil carbon content and sequestration.
Methods
Site Selection The strength of having a regional project address carbon sequestration in the Southeastern US is that a diverse geographical group can easily obtain quality data over an extensive geographical region. The weakness is that the project is unfunded and the task has infinite possibilities. The southern region is highly diverse in the combination of land uses, crop rotations, tillage practices, soil textures, and ecosystem ages/rotation durations that are present within each state. Therefore, to capture this regional diversity, sites will be selected to represent the major land use, crop rotation, tillage practice, soil texture, and ecosystem age/rotation duration combinations that characterize each state. Multiple participants from a particular state will coordinate with each other so as to provide some level of treatment combination replication, but also to provide a diversity of sites. The southern region soil physics group has a long history of productive regional projects that, in more recent projects, targeted certain Major Land Resource Areas (MLRAs) for soil physical, chemical, and hydraulic property characterization as related to solute transport. Based on this previous regional project work, which was described above and from which much data have been gathered, site selection for the present proposed regional project will be partly guided by past site selections with the goal of using previously collected data from past sites. Project participants will also be encouraged to investigate previous work in their state to possibly locate additional sites that have been previously sampled in other projects to use in this project. Any previous data on soil carbon from the various participating states will be used to help guide decisions about new site selections. An additional goal of the site selection process will be to include sites of similar treatment combination from as many states throughout the region as possible. Thus, where possible, participants will replicate field measurements in multiple counties within a MLRA. Table 1 summarizes an initial proposed list of MLRAs, ecosystems, crop rotation systems, tillage practices, and soil surface textures to be targeted for sampling within participating states. Table 1 also provides an indication of whether previous data/samples exist or first-time sampling will have to be conducted for the proposed target treatment combinations. To be noted from Table 1 is that there will be multiple replications of various treatment combinations across multiple states that will contribute to statistical power for data analyses. It will be important that a sufficient number of samples representing various treatment combinations be collected to allow the opportunity to detect SOC changes over a period of time. Based on the observed variability in a preliminary dataset from samples previously collected in Arkansas by the proposed methods (described below), the estimated average annual rate of SOC change in the top 20 cm over a 4-yr period that should be detectable ranges from 2.33 to 0.26 Mg/ha/yr in undisturbed grassland and from 1.28 to 0.14 Mg/ha/yr in agricultural cropland for sample numbers of 2 and 160, respectively (Table 2). Soil Sampling Protocol A 60-m line transect will be established at each new site selected for soil sampling. A soil core with an approximate diameter of 4.8 cm will be collected from the 0- to 10- and 10-20-cm depths with a slide hammer and core chamber, similar to that pictured in Figure 1, at various intervals along the 60-m transect to determine bulk density and other soil physical and chemical properties. The soil sampling chamber will be beveled to the outside to minimize compaction upon sampling and may be of variable diameter among project participants collecting soil samples. Each collaborator will choose an appropriate number of representative samples to collect considering their available resources and the hypothesized rate of SOC change at their site. Since time of year greatly affects SOC concentrations, all samples will be attempted to be collected following any crop harvest, but before field manipulations the following year. The sample for the 10- to 20-cm soil depth will be collected from immediately adjacent to the cavity left from collecting the 0- to 10-cm soil core (Figure 2). For sites which will not be cultivated, the holes created by removing the soil samples will be filled with sand. This will prevent possible livestock injury and serve as a visual indicator to prevent accidental re-sampling of the exact same location in the future. New soil cores will be air-dried, weighed, and subsequently crushed and sieved through a 2-mm mesh screen for soil physical and chemical analyses. A sub-sample of the air-dried soil will be oven-dried for soil moisture correction and will be used for additional soil physical and chemical analysis, except that all carbon analysis will be performed on air-dry samples. Samples will be stored until funding is secured to conduct the desired chemical analyses. A similar sample collection and sample processing approach has been used in numerous prior studies investigating landuse effects on soil properties in tallgrass prairies (Brye, 2003, 2006; Brye and Slaton, 2003; Brye and Pirani, 2005; Brye and West, 2005; Brye and Moreno, 2006; Brye et al., 2004a,b). Any soil re-sampling, either of new sites selected for this project or sites previously sampled from past research, will be conducted after a minimum of four years. For consistency and for the purpose of this project, soil sampling efforts will focus on the top 20 cm, the zone most that will be most influenced by varying management practices (Kucharik, 2007). However, to document possible deeper-soil accumulation of C, a sub-set of sampling sites along the climate gradient in Texas, Oklahoma, Arkansas, Mississippi, Alabama, Georgia, and North Carolina will be sampled to a depth of 60 cm. These additional deep-soil samples will be collected from the 20-40 and 40-60 cm depth intervals at a minimum of two of the sample locations (i.e., the beginning and end of the transect) along the established 60-m transect. To minimize potential compaction, if there is more than a 5% difference in the length of the collected deep core compared to the actual target length, then the sample will be discarded and re-collected. To prevent effects from soil processes associated with slope, e.g. more organic carbon accumulation in a toeslope compared to a backslope position, participants will sample from relatively level fields (< 5% slope) and document slope and overall landform from which the samples were collected. Also to be recorded, measured, or determined for each site will be Global Positioning System (GPS) coordinates for the beginning and end of the transect, the vegetation or crop present and, if possible, the per area grain or biomass yield produced, the duration in the present crop rotation, the regional 30-year mean annual air temperature and precipitation from the nearest NOAA weather station, and where possible the local soil temperature. Laboratory Analyses Sub-samples of oven-dry, sieved soil will be extracted with Mehlich-3 extractant solution (Tucker, 1992) in a 1:10 (w/v) soil-to-extractant solution ratio and analyzed for extractable soil nutrients (i.e., P, K, Ca, Mg, Na, S, Fe, Mn, Zn, and Cu) via inductively coupled argon-plasma spectrophotometry by the University of Arkansas Soil Testing Laboratory. Soil pH and EC will be determined with an electrode on a 1:2 (w/v) soil-to-water paste. Soil organic matter will be determined by weight-loss-on-ignition after 2 hrs at 105 oC and 2 hrs at 360 oC. Soil inorganic carbon (SIC) will be determined using pressure calcimeter method (Sherrod et al., 2002) in the laboratory of Dr. Cristine Morgan at Texas A&M University. Total soil C and N will be determined on all samples collected by high-temperature combustion through a single laboratory to be determined at a later date based on the availability of funding. Soil particle-size analysis will be conducted in project participants own laboratories using the 12-hr hydrometer method (Gee and Bauder, 1986). Data Manipulations Measured soil concentrations (i.e., mass-per-mass basis) will be converted to contents (i.e., mass-per-area basis) on a sample-by-sample basis using the 10-cm sampling interval and the measured bulk density. The soil C:N ratio will be calculated from the measured total C and N concentrations. Soil organic C will be calculated, if necessary, by difference from the direct measurements of TC and SIC. Carbon and N sequestration rates will be calculated based on the change in soil C or N content in a particular soil depth interval divided by the time (to the nearest day) between samplings. Data Analyses Multiple project participants have varying levels of expertise with traditional and spatial statistical data analyses that will be relied upon to analyze the data collected in this project. To support project participants expertise, a statistician will be consulted prior to soil sampling new sites and once data have been collected to ensure that the most-appropriate and valid statistical approaches and models are used to analyze the data and assist with interpretation of results. It is anticipated that, due to the likelihood of missing treatment combinations, multiple separate statistical analyses will need to be conducted to address specific questions and/or hypotheses using sub-sets of the entire collected data set. A variety of traditional and spatial statistical approaches will be used to analyze the data based on the specific questions and/or hypotheses to be addressed. Using a combination of previously and newly collected data to represent the initial soil sampling, data will be analyzed by analysis of variance (ANOVA) using SAS (SAS Institute Inc., Cary, NC), or a similar software package, to determine effects of weather (i.e., the combination of regional mean annual air temperature and precipitation and local soil temperature), soil texture, land use, and soil depth on soil C and N concentration and content, soil bulk density, and other measured soil properties. A separate ANOVA will be conducted for the agricultural ecosystems only to determine the effects of climate, soil texture, soil depth, crop rotation, rotation duration, and tillage on soil C and N concentration and content, soil bulk density, and other measured soil properties. Multiple regression techniques will be used to determine predictive models for soil C and N content. Data obtained from Iowa will be used as a very basic initial attempt to compare soil C and N contents and sequestration between upper-Midwest and southern conditions. Once data from the second soil sampling have been obtained, an ANOVA will be conducted to evaluate the effects of weather, land use, soil texture, and soil depth on soil C and N sequestration and the change in other measured soil properties over time. A separate ANOVA will be conducted for the agricultural ecosystems only to determine the effects of weather, soil texture, soil depth, crop rotation, rotation duration, and tillage on soil C and N sequestration and the change in other measured soil properties over time. Multiple regression techniques will again be used to determine relationships among measured variables to develop predictive models for soil C and N sequestration. Additional statistical analyses will be made in response to other questions and/or hypotheses that may arise at a later date once initial data analyses have been made. Actual experimental designs for each statistical analysis will be determined in consultation with a statistician once data have been collected and based on the desired evaluation to be made. Means will be separated by least significant difference at the 0.05 level. Soil C sequestration rates will be expressed within upper and lower limits of their associated variability. Additional spatial statistical techniques will be explored, if applicable, depending on the final number of sites sampled and their spatial distribution throughout the region.Measurement of Progress and Results
Outputs
- This project will generate essential, scientifically based field data to support accurate projections of and provide guidance for soil C sequestration potentials across the climate gradient of the southern US. Collected data and findings will be made available via the internet and accessible through the groups website, which will be created to facilitate dissemination of information generated in the proposed project. The group project will stimulate common understanding, shared research, and provide an educational platform among southern US academic institutions and government partners.
- Collected data and findings will be made available via the internet and accessible through the groups website, which will be created to facilitate dissemination of information generated in the proposed project. The group project will stimulate common understanding, shared research, and provide an educational platform among southern US academic institutions and government partners.
Outcomes or Projected Impacts
- By documenting SOC accumulation under different climates, land uses, and soils, it is expected that the results of this project will provide producers, policy makers, and the CCX credible and comprehensive information on the environmental merit of adopting different land uses such as bio-energy crops and conservation-tillage practices in the southern US. It is also expected that the project will facilitate the establishment of a C-trading farm economy in the southern US by providing credible and realistic data on soil C sequestration potentials.
- Aside from the information and data to be generated, the activities associated with this project will promote teaching, training, and learning at both the graduate and undergraduate student levels. It is expected that the project cooperators will involve their undergraduate students in field activities, such as collecting soil samples, and in laboratory activities, such as processing samples and conducting analyses. Undergraduate students will also have the opportunity to compile data under mentorship of graduate students and/or the project collaborator. Once a year, these participating students would have the opportunity to present a summary of their results in a teleconference with the working group or in-person during regular annual meetings. This type of activity provides students with some experience in basic statistics and presentation skills. It also provides an opportunity for students to interact with a geographically diverse group of faculty, researchers, and other students across the states and institutions involved in this project.
- The experimental infrastructure to be created will enhance education, research, and collaboration between the southern Universities, federal agencies, such as the Natural Resource Conservation Services Soil Survey Division, which is very interested in compiling databases on benchmark soils and dynamic soil properties such as soil C, and/or the US Forest Service, and other state and local agencies involved in natural resource conservation and management. The USDAs Voluntary Reporting Carbon Management Tool (COMET-VR) is one such database of soil C sequestration, which uses data compiled from the Carbon Sequestration Rural Appraisal along with the Century model (Parton et al., 1988) to estimate annual C fluxes (USDA, 2007; Milne et al., 2008). However, one current limitation of the Century model is that little soil C sequestration data is available for areas where flood-irrigated rice is a main row-crop, such as in areas of Texas, California, Arkansas, Mississippi, and Louisiana (Milne et al., 2008). Thus, improving our understanding of the relationships among climates, soils, land uses, and C will contribute to increased awareness and protection of our natural resources and improve the quality of our air, soil, and water for future generations. It is also anticipated that this information will lead to increased on-farm profits for those producers who chose to use more-sustainable land management practices.
Milestones
(2009): Accumulate past data and data from a small sub-set of participants from initial soil sampling efforts to use to assess anticipated variability, which will also be used as the foundation for discussions at the annual meeting in May 2010 that may lead to possible refine of methods.(2010): Initial soil samples will be collected and processed for new proposed sites and site information will be complied for all sampled sites including sites previously sampled in past studies; samples will be stored until adequate funding is secured to conducted desired chemical analyses; conduct soil particle-size analyses; preliminary data analyses will be initiated; begin seeking extramural funding to support laboratory analyses; discuss preliminary results and project progress at annual meeting in May 2010, make refinements to procedures if necessary.
(2011): 2011 through 2014: Continue seeking extramural funding opportunities; continue data analyses; conduct laboratory analyses based on available funding; discuss results and project progress at annual meetings; initiated re-sampling of sites; begin processing and analyses of new samples.
(2015): Complete re-sampling of sites, sample processing, and chemical analyses; conduct final data analyses; discuss options for dissemination of information; begin preparing manuscripts for peer-review; complete sampling and data analysis portions of project by December 31st, 2015.
Projected Participation
View Appendix E: ParticipationOutreach Plan
Project participants will immediately be encouraged to seek funding from within their own state, institution, or agency to support travel and laboratory analyses for samples they collect. Project participants will also immediately begin searching for extramural funding opportunities for the whole group of states in the southern region and/or a sub-set of states in the region to support project activities, particularly laboratory chemical analyses. For example, the rice-producing states could target the Rice Foundation for funding to evaluate soil carbon storage and sequestration potential under various tillage practices for rice production. Similarly, soybean-producing states could target the United Soybean Board and cotton-producing states could target Cotton Incorporated for similar research. The whole regional project could also be used to seek funding through the Southern Regions Sustainable Agriculture Research & Education (SARE) funding opportunities.
Project participants will collaboratively discuss the research results on an annual basis and collectively determine how to refine the procedures, if necessary, particularly following the stage of investigating preliminary data. Project participants will also collectively determine how to present and publish/disseminate project results. Results of this project will likely be communicated to the scientific community via several mechanisms including refereed journal publications and oral and poster presentations made at annual meetings of the American Society of Agronomy, Soil Science Society of America, and perhaps the Soil and Water Conservation Society. Information gathered in this project will also be provided to collaborating agencies, such as the NRCS, and other local and state agencies and other interested groups who could benefit from knowledge obtained in this project. Project participants will also likely present results at local field days and/or workshops and collaborate with State Extension colleagues to prepare fact sheets for distribution to state clientele. Preparation of a project web page will facilitate information exchange among project participants and others interested in this project.
Organization/Governance
The Regional Technical Committee will follow the operational procedures listed on pages 19-22 and 34-36 of the CSRS "Manual for Cooperative Regional Research" revised and dated October 1992. The voting membership of the Regional Technical Committee includes one representative from each cooperating agricultural experiment station or institution appointed by the director and a representative of each cooperating USDA-ARS research unit or location. The administrative advisor and the CSRS representative are non-voting members. All voting members of the Technical Committee are eligible for office. The offices of the Regional Technical Committee include the Chair, the Vice-Chair and the Secretary. These officers will be elected annually at the Technical Committee meeting, and they may succeed themselves.
The duties of the Technical Committee are to coordinate work activities related to the project. The Chair, in accord with the Administrative Advisor, will notify the Technical Committee of the time and place of the meeting, will prepare the agenda and preside at meetings of the Technical Committee and Executive Committee. He or she is responsible for preparing the annual progress report and coordinating the preparation of regional reports. The Vice-Chair assists the Chair in all functions and the Secretary records the minutes and performs other duties assigned by the Technical Committee or Administrative Advisor. The Chair appoints subcommittees as needed. Annual meetings will be held by the Technical Committee, as authorized by the Administrative Advisor, for the purpose of conducting business related to the project.
Literature Cited
Baker, J.M., T.E. Ochsner, R.T. Venterea, and T.J. Griffis. 2007. Tillage and soil carbon sequestration - What do we really know? Agric. Ecosys. Environ. 118:1-5.
Bruce, R.R., J.H. Dane, V.L. Quisenberry, N.L. Powell, and A.W. Thomas. 1983. Physical characteristics of soils in the southern region - Cecil. South. Coop. Ser. Bull. 267.
Brye, K.R. 2003. Long-term effects of cultivation on particle size and water-retention characteristics determined using wetting curves. Soil Sci. 168:459-468.
Brye, K.R. 2006. Soil physiochemical changes following 12 years of annual burning in a humid-subtropical tallgrass prairie: a hypothesis. Acta Oecol. 30:407-413.
Brye, K.R., and A.L. Pirani. 2005. Native soil quality and the effects of tillage in the Grand Prairie region of eastern Arkansas. Am. Midl. Nat.154:28-41.
Brye, K.R., and C.J. Kucharik. 2003. Carbon and nitrogen sequestration in two prairie topochronosequences on contrasting soils in southern Wisconsin. Am. Midl. Nat. 149:90-103.
Brye, K.R., and C.P. West. 2005. Grassland management effects on soil quality in the Ozark Highlands. Soil Sci. 170:63-73.
Brye, K.R., and L. Moreno. 2006. Vegetation removal effects on soil quality in a native tallgrass prairie fragment in east-central Arkansas. Nat. Areas J. 26:94-100.
Brye, K.R., and N.A. Slaton. 2003. Carbon and nitrogen storage in a typic albaqualf as affected by assessment method. Comm. Soil Sci. Plant Anal. 34:1637-1655.
Brye, K.R., D.E. Longer, and E.E. Gbur. 2006a. Impact of tillage and reside burning on CO2 flux in a wheat-soybean production system. Soil Sci. Soc. Am. J. 70:1145-1154.
Brye, K.R., C.P. West, and E.E. Gbur. 2004a. Soil quality differences under native tallgrass prairie across a climosequence in Arkansas. Am. Midl. Nat. 152:214-230.
Brye, K.R., E.E. Gbur, and D.M. Miller. 2004b. Relationships among soil C and physiochemical properties of a typic albaqualf as affected by years under cultivation. Comm. Soil Sci. Plant Anal. 35:177-192.
Brye, K.R., M.L. Cordell, D.E. Longer, and E.E. Gbur. 2006b. Residue management practice effects on soil surface properties in a young wheat-soybean double-crop system. J. Sustain. Agric. 29:121-150.
Cassel, D.K. 1985. Physical characteristics of soils of the southern region - summary of in situ unsaturated hydraulic conductivity. South. Coop. Ser. Bull. 303. Regional Research Project S-124. North Carolina State University.
Causarano, H.J., A.J. Franzluebbers, D.W. Reeves, and J.N. Shaw. 2006. Soil organic carbon sequestration in cotton production system of the southeastern United States: a review. J. Environ. Qual. 35:1374-1383.
Chicago Climate Exchange (CCX). 2006. CCX Agricultural Carbon Emission Offsets [On-line]. Available at http://www.chicagoclimatex.com/news/publications/pdf/CCX_Soil_Offsets.pdf (verified 10 March 2007).
Collins, H.P., P.E. Rasmussen, and C.L. Douglas. 1992. Crop rotation and residue management effects on soil carbon and microbial dynamics. Soil Sci. Soc. Am. J. 56:783-788.
Curtin, D., H. Wang, F. Selles, B.G. McConkey, and C.A. Campbell. 2000. Tillage effects on carbon dioxide fluxes in continuous wheat and fallow-wheat rotations. Soil Sci. Soc. Am. J. 64:2080-2086.
Dane, J.H., D.K. Cassel, J.M. Davidson, W.L. Pollans, and V.L. Quisenberry. 1983. Physical characteristics of soils of the southern region - Troup and Lakeland Series. South. Coop. Ser. Bull. 262. Alabama Agricultural Experiment Station and Auburn University.
Doran, J.W. 1980. Soil microbial and biochemical changes associated with reduced tillage. Soil Sci. Soc. Am. J. 44:765-771.
Follet, R.F., D.C Reicosky, G. Belanger, D.A. Angers, J.D. Reeder, and G.E. Schuman. 2001. Carbon sequestration. Available at http://www.accessscience.com. DOI 10.1036/1097-8542.YB010280 (verified 4 Sept. 2008).
Fontana, A, M.G. Pereira, L.H. Cunha dos Anjos, and V.M. Benites. 2008. Distribution of Organic Carbon in the Humic Fractions of Diagnostic Horizons from Brazilian Soils. Comm. Soil Sci. Plant Anal. 39:951-971.
Fortin, M.C., P. Rochette, and E. Pattey. 1996. Soil carbon dioxide fluxes from conventional and no-tillage small-grain cropping systems. Soil Sci. Soc. Am. J. 60:1541-1547.
Freibauer, A., M.D.A. Rounsevell, P. Smith, and J. Verhagen. 2004. Carbon sequestration in the agricultural soils of Europe. Geoderma 122:1-23.
Gee, G.W., and J.W. Bauder. 1986. Particle size analysis. p. 383-411. In A. Klute (ed.) Methods of Soil Analysis. Part 1. 2nd ed. ASA and SSSA, Madison, WI.
Ihori, T., I.C. Burke, W.K. Lauenroth, and D.P. Coffin. 1995. Effects of cultivation and abandonment on soil organic matter in northeastern Colorado. Soil Sci. Soc. Am. J. 59: 1112-1119.
Intergovernmental Panel on Climate Change (IPCC). 2001. Climate Change 2001: The Scientific Basis. Contribution of Working Group I to the Third Assessment Report of the Intergovernmental Panel on Climate Change [Houghton, J.T., Y. Ding, D.J. Griggs, M. Noguer, P.J. van der Linden, X. Dai, K. Maskell, and C.A. Johnson (eds.)]. Cambridge University Press, Cambridge, United Kingdom and New York, NY, USA, 881pp.
Kucharik, C.J. 2007. Impact of prairie age and soil order on carbon and nitrogen sequestration. Soil Sci. Soc. Am. J. 71:430-441.
Lal. R., R.F. Follett, and J.M. Kimble. 2003. Achieving soil carbon sequestration in the United States: a challenge to policy makers. Soil Sci. 168:827-845.
Lemus, R., and R. Lal. 2005. Bioenergy crops and carbon sequestration. Crit. Rev. Plant Sci. 24:1-21.
Liebig, M.A., H.A. Johnson, J.D. Hanson, and A.B. Frank. 2005. Soil carbon under switchgrass stands and cultivated cropland. Biomass Bioenergy 28:347-354.
Magdoff, F., and H. van Es. 2000. Building Soil for Better Crops. 2nd Edition. Handbook Series 4. Sustainable Agriculture Network, USA. 230pp.
McCarl, B.A., and U.A. Schneider. 2001. Greenhouse gas mitigation in U.S. agriculture and forestry. Science 294:2481-2482.
Milne, E., S. Williams, K. Brye, M. Easter, K. Killian, and K. Paustian. 2008. Simulating soil organic carbon in a rice-soybean-wheat-soybean chronosequence in Prairie County, Arkansas using the Century model. Elect. J. Integr. Biosci. In Press
Nelson, D.W., and L.E. Sommers. 1996. Total carbon, organic carbon, and organic matter. In D.L. Sparks (ed.) Methods of Soil Analysis. Part 3 - Chemical Methods. ASA and SSSA, Madison, WI.
Nofziger, D.L., J.L. Williams, A.G. Hornsby, and A.L. Wood. 1983. Physical characteristics of soils of the southern region - Bethany, Konawa, and Tipton Series. South. Coop. Ser. Bull. 265. Oklahoma State University.
Oades, J.M. 1995. Recent advances in organomineral interactions: Implications for C cycling and soil structure. p. 119-133. In P.M. Huang, J. Bertherlin, J.M. Bollag, W.G. McGrill, and A.L. Page (eds.) Environmental impact of soil Component Interactions. Vol 1: Natural and Anthropogenic Organics. Lewis Publishers USA.
Omonode, R.A., and T.J. Vyn. 2006. Vertical distribution of soil organic carbon and nitrogen under warm-season native grasses relative to croplands in west-central Indiana, USA Agric. Ecosys. Environ. 117:159-170.
Percival, H.J., R.L. Parfitt, and N.A. Scott. 2000. Factors controlling soil carbon levels in New Zealand grasslands: Is clay content important? Soil Sci. Soc. Am. J. 64:1623-1630.
Parton, W.J., J.W.B. Stewart, and C.V. Cole. 1988. Dynamics of C, N, P and S in grassland soils: a model. Biogeochem. 5:109-131.
Post, W.M., and K.C. Kwon. 2000. Soil carbon sequestration and land-use change: processes and potential. Global Change Biol. 6:317-327.
Post, W.M., R.C. Izaurralde, J.D. Jastrow, B.A. McCarl, J.E. Amonette, V.L. Bailey, P.M. Jardine, T.O. West, and J. Zhou. 2004. Enhancement of carbon sequestration in US soils. Biosci. 54:895-908.
Potter, K.N., H.A. Torbert, H.B. Johnson, and C.R. Tischler. 1999. Carbon storage after long-term grass establishment on degraded soils. Soil Sci. 164:718-725.
Quisenberry, V.L., D.K. Cassel, J.H. Dane, and J.C. Parker. 1987. Physical characteristics of soils of the southern region - Norfolk, Dothan, Wagram, and Goldsboro Series. South. Coop. Ser. Bull. 263. South Carolina Agricultural Experiment Station and Clemson University.
Rawls, W.J., Y.A. Pachepsky, J.C. Ritchie, T.M. Sobecki, and H. Bloodworth. 2003. Effect of soil organic carbon on soil water retention. Geoderma 116:61-76.
Reicosky, D.C. 1997. Tillage-induced CO2 emissions from soil. Nut. Cycl. Agroecosys. 49:273-285.
Rhoton, F.E. 2000. Influence of time on soil response to no till practices. Soil Sci. Soc. Am. J. 64:700-709.
Romkens, M.J.M., H.M. Selim, H.D. Scott, R.E. Phillips, and F.D. Whisler. 1986. Physical characteristics of soils in the southern region - Captina, Gigger, Grenada, Loring, Olivier, and Sharkey Series. Reg. Bull. 264. Mississippi Agricultural and Forestry Experiment Station, Mississippi State University, and University of Mississippi.
Romkens, M.J.M., H.M. Selim, R.E. Phillips, and F.D. Whisler. 1985. Physical characteristics of soils in the southern region - Vicksburg, Memphis, Maury Series. South. Coop. Ser. Bull. 266. Regional Research Project S-124.
Schlesinger, W.H. 2000. Soil respiration and the global carbon cycle. Biogeochem. 48:7-20.
Schneider, U.A., and B.A. McCarl. 2003. Economic potential of biomass based fuels for greenhouse gas emission mitigation. Environ. Res. Econ. 24:291-312.
Scott, H.D. 1999. Water and chemical transport in soils of the southeastern United States. Special Report 197. Arkansas Agricultural Experiment Station. University of Arkansas.
Sherrod, L.A., G. Dunn, G.A. Peterson, and R.L. Kolberg. 2002. Inorganic carbon analysis by modified pressure-calcimeter method. Soil Sci. Soc. Am. J. 66:299-305.
Silver, W.L., R. Osterlag, and A.E. Lugo. 2000. The potential for carbon sequestration through reforestation of abandoned tropical agricultural and pasture lands. Restor. Ecol. 8:394-407.
Six, J., H. Bossuyt, S. Degryze, and K. Denef. 2004. A history of research on the link between (micro)aggregates, soil biota, and soil organic matter dynamics. Soil Tillage Res. 79:7-31.
Tilman, D., J. Hill, and C. Lehman. 2006. Carbon-negative biofuels from low-input high-diversity grassland biomass. Science 314:1598-1600.
Tolbert, V.R., D.E. Todd, L.K. Mann, C.M. Jawdy, D.A. Mays, R. Malik, W. Bandaranayake, A. Houston, D. Tyler, and D.E. Pettry. 2002. Changes in soil quality and below-ground carbon storage with conversion of traditional agricultural crop lands to bioenergy crop production. Environ. Poll. 116:S97-S106.
Undersander, D., and C. Reiger. 1985. Effect of wheat residue management on continuous production of irrigated winter wheat. Agron. J. 77:508-511.
United States Department of Agriculture (USDA). 2007. The Voluntary Reporting of Greenhouse Gases-CarbOn Management Evaluation Tool (COMET-VR). [online]. Available at http://www.cometvr.colostate.edu/ (verified 22 Nov. 2007).
United States Environmental Protection Agency (USEPA). 2006. Climate Change - Science: State of Knowledge [On-line]. Available at http://www.epa.gov/climatechange/science/stateofknowledge.html (verified 3 March 2007).
VandenBygaart, A.J., E.G. Gregorich, and D.A. Angers. 2003. Influence of agricultural management of soil organic carbon: a compendium and assessment of Canadian studies. Can. J. Soil Sci. 83:363-380.
Walsh, M.E., D.L.T. Ugarte, H. Shapouri, and S.P. Slinsky. 2003. Bioenergy crop production in the United States. Environ. Res. Econ. 24:313-333.
Wander, M. 2004. Soil organic matter fractions and their relevance to soil function. p. 67-102. In F. Magdoff, and R.R. Weil (eds.) Soil Organic Matter in Sustainable Agriculture. CRC Press, USA.
Weil, R.R. and F. Magdoff. 2004. Significance of soil organic matter to soil quality and health. p. 1-44. In F. Magdoff and R.R. Weil (eds.) Soil Organic Matter in Sustainable Agriculture. CRC Press, USA.
West, T.O., and W.M. Post. 2002. Soil organic carbon sequestration rates by tillage and crop rotation: a global analysis. Soil Sci. Soc. Am. J. 66:1930-1946.