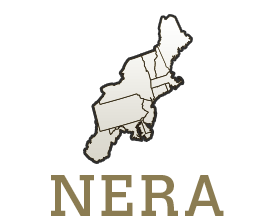
NE1442: Poultry Production Systems and Well-being: Sustainability for Tomorrow
(Multistate Research Project)
Status: Inactive/Terminating
NE1442: Poultry Production Systems and Well-being: Sustainability for Tomorrow
Duration: 10/01/2014 to 09/30/2019
Administrative Advisor(s):
NIFA Reps:
Non-Technical Summary
Statement of Issues and Justification
Over the past several decades improved poultry production systems have contributed to significantly enhanced performance traits (egg production, growth rates, meat yields, livability, feed conversion) and have provided economic, nutritious, and safe food choices. However, the poultry industry is also increasingly being challenged to address consumer and general public concerns about animal welfare and environmental issues. Additionally, legitimate changes to production systems, such as novel housing systems, are being developed with little knowledge as to their appropriateness and impacts positively and negatively on bird welfare, productivity, food safety, and economic sustainability. Tensions between efficient production (safe, affordable food) and perceptions of consumers will certainly continue and intensify in the near term. Therefore it is imperative that the basis for future production practices be based on sound science that includes a strong emphasis on bird physiology and behavioral indicators of well-being in all types of production systems, as well as considerations of the social and environmental sustainability of these practices.
The U.S. Poultry & Egg Association and Egg Industry Center, primary organizations that represents the poultry industry, have identified the development of energy/resource efficient production systems for poultry as a research need. This includes consideration of animal welfare, nutrition, ventilation, lighting, air quality, environmental footprint, and food safety/security. A related research need identified by these organizations is the environmental, welfare, and economic effects of the movement toward alternative production systems. [http:// http://www.uspoultry.org/research/] [http://www.eggindustrycenter.org]
Poultry production systems are continuing to change to address both welfare and environmental issues. However, the efficiency of energy/resource use must be optimized to ensure the sustainability of the poultry industry; otherwise poultry products will be compromised from a security, quality, and affordability perspective.
If the poultry industry does not remain sustainable it will be impossible for them to remain competitive in the global market, which will result in more poultry production occurring outside the U.S. This may lead to a decrease in poultry production within the U.S. and an increase in importation of poultry products.
Historically, this has been a successful interdisciplinary multistate research project involving nutritionists, environmental physiologists, neuroscientists, ethologists, engineers, operations researchers, extension specialists and economists. The broad scope of interests allows for research to be conducted in relation to a range of numerous aspects of poultry production. These collaborators have the facilities (both lab and commercial scale) and equipment necessary to continue work in the proposed areas.
Objectives:
Collaborators at the experimental stations in AR, CA, CT, GA, GA-ARS, IL, IA, IN-ARS, MD, MI, MN, MS-ARS, NE, NC, PA, TX, WI, Bern Switzerland, will work on research related to the following objectives.
1. Energy/resource efficiency
This will include shared efforts on feed and fuel energy sources for poultry and facilities by geographical region; facility design, equipment efficiency, management, and modeling energy use in poultry systems.
2. Evaluating commercial poultry production systems
This will include joint efforts on the characterization of the performance of conventional, alternative, and organic poultry production systems relative to air and water quality, nutrient management, acoustic environment, and animal health and welfare.
3. Establishing parameters influenced by the production system and strains utilized within the poultry industry
This collaborative research will encompass the areas of poultry nutrition, physiology, behavior, well-being, food safety and quality, and economic evaluation of poultry production systems.
Some of the critical and unique instruments and facilities that will be shared/used collaboratively are spectra radiometers (CT) and audiology analysis systems (CT, NE), hypo/hyperbaric chambers (TX), emission chambers (PA, IA, MI), portable air emission monitoring trailers (IA, IL, MI), multi-channel telemetric body temperature sensing system with ingestible sensors (IA), infrared thermal imager for quantification of surface temperature distribution (CA, IA, GA, MI), multi-station individual bird feeding units (IA, MI, NC), environmental chambers (IL, MS, CA, PA), and four large-scale indirect animal calorimeters/emission chambers that allow for simulation of commercial production settings (IA). We also have state-of-the-art poultry production facilities (IL, CT, GA, MN, IA, MI, MS, NC, PA, Bern Switzerland) and energy monitoring equipment (MS-ARS) available. The project includes advanced food safety and egg quality laboratories (GA-ARS, NC) and a pilot shell egg processing plant (NC, PA). In addition, there is a network of established relationships with poultry producers that allows collection of large-scale data in a commercial setting (CA, MI, MS, NC, IA, PA). These are highly specialized pieces of equipment and/or facilities already in place that would be prohibitive to reproduce at other universities. Collaborative use through this project maximizes both efficiency of use and research productivity. This project now adds the use of biotechnology and avian genomics to use mRNA expression to develop new molecular biomarkers that can be used to assess nutrient status (WI). In addition, this project has the distinct advantage of poultry behavioral expertise (CA, IN, GA, MI, NC) that is lacking in other poultry regional projects. We also have expertise from colleagues abroad (Switzerland, Spain, Canada, etc.) who can offer insights and practical experience with changes in poultry production systems (with their outcomes, expected and otherwise) that have occurred in their own countries and that U.S. producers and special interest groups are considering.
The complexity of the questions and problems that will be addressed by this project could not be accomplished at any single station, thus requiring a multi-state approach to evaluate the tradeoffs that might exist in the alternative production systems. The level and diversity of expertise that this project brings together cannot be found at any single institution. Additionally, the equipment and facilities available for use in this research would also not be found in any single location. The decrease in funding sources requires collaborative efforts from individuals in various disciplines to assess the questions being asked. This multi-expertise/multistate effort will eliminate duplication of effort and conserve resources.
Successful completion of the endeavors outlined in this proposal will lead to 1) increased knowledge of basic physiological and behavioral processes in poultry; 2) identification of meaningful relationships between environmental factors and their associated production and economic ramifications; 3) enhanced management-decision making and action taking initiatives; and 4) enhanced understanding of poultry feed/fuel energy parameters and ramification for sustainable and profitable production. With this information, housing environments can be optimized by defining environmental conditions (aerial, thermal, spectral, spatial) and management practices (nutritional, behavioral) that will result in production systems, which promote bird welfare, performance, food safety and security, environmental soundness, and ultimately sustainable development of the U.S. poultry industry.
Related, Current and Previous Work
A CRIS search on poultry environment or production system did not identify any active multi-state project other than the existing NE-1042.
Contributions of NE-1042 investigators.
NE-1042 was founded in 1978 to facilitate research in the area of poultry production recognizing collaboration and communication amongst the research institutions would advance poultry management strategies. NE-1042 has grown from 11 universities in 1999 to 22 universities and USDA-ARS labs for the 2009 renewal and expanding to 25 universities, USDA-ARS labs, and additional international partners for this 2014 rewrite. An annual meeting is held each October to discuss research results and plan collaborative projects for the coming year. The NE-1042 philosophy is to help implement sustainable practices in poultry production for the industry from a multi-state collaborative approach recognizing more advances can be made than working as a single investigator initiated grant. The complexities of the animal-environment interactions overlaid with animal welfare and consumer perceptions require a multi-discipline, collaborative approach to identifying ways to best achieve the producer and societal goals.
Examples of sustained endeavors by NE-1042 participants include:
1. History of publication in the scientific literature.
Over the last 5 project years (2009-2013), this regional poultry research group has published 412 abstracts, 119 peer-reviewed journal articles, 10 popular-press articles, 9 peer-reviewed extension reports, and 55 proceedings at national and international meetings.
2. Success with joint proposals demonstrates the capacity of NE-1042 to coordinate ideas, resources, and execute multi-state research projects.
The multistate research group has secured over $22 million in grants to conduct research with the NE-1042 project during the last project cycle (5-year) period.
3. Collaborative research previously conducted and currently being worked on by these research stations is listed below.
Current Work: Literature Review
A. Measuring and Assessing Elements of the Physical Environment
Ventilation. Many environmental factors affect the growth of broilers and turkeys in a commercial setting. The poultry house ventilation system interfaces with heating and cooling systems in the control of barn temperature, humidity, and air exchange that ultimately affect air and litter quality and thermal environment. These factors can either positively or negatively affect growth rate, uniformity, feed consumption, and feed conversion of birds during the brooding and growing phases.
The proposed MRP intends to study air quality, ventilation rates, and heat stress avoidance. Proper carbon dioxide (CO2) balance is important to maintain within the poultry house (Xin et al., 2009). It has been documented that turkey poults exposed to levels of over 25 to 30 ppm CO for just a few hours may have a higher risk of spontaneous turkey cardiomyopathy (STC) (round heart disease) (Frame et al., 2010). In addition to the negative effect of increased CO, increased levels of CO2 is also of concern when conventional brooders are used during cold weather conditions. Thus, based on this research finding, increased CO2 levels can have deleterious effects on turkey health, particularly during the brooding phase.
The effects of air quality and heat stress on laying hen performance has also been studied extensively (Green and Xin, 2009a,b; Green et al., 2009; Hayes et al., 2013a,b,c; Zhao et al., 2013a,b). Reilly et al. (1991) found water-cooled perches were effective in improving broiler performance in heat stress conditions. A similar approach to alleviate heat stress in laying hen systems that include perches warrants further investigation. Surface wetting using overhead sprinklers to cool broiler chickens have gained popularity in the commercial broiler production in the southern United States. Surface wetting reduces heat stress by resembling artificial sweat, reducing surface temperature and preventing the rise of core body temperature (Tao and Xin, 2003). Sprinkler cooling in the floor-raised production house can substantially reduce cooling water usage (Liang et al., 2013) and may improve animal well-being. However, the heat and mass transfer properties of a chicken surface with various amount of feather coverage are unknown.
Lighting. Energy efficient lighting is important for reducing electrical energy inputs and maximizing outputs of a poultry enterprise. New technology, in the area of LED lighting, is being quickly adopted, in market turkeys, without much research. The same is true with laying hens in alternative housing systems. Producers need unbiased information on the proper types of lighting devices to select. Field research information is also needed regarding the impact of LED lights on the performance of the birds. Use of LED, as compared with other lighting options such as fluorescent lights, represents a major upfront investment.
Poultry behavior is modified depending on light wavelength, intensity and photoperiod (Marchewka et al., 2013). Specific wavelengths can influence activity and feather pecking incidence with red wavelengths promoting activity while blue light reduces activity (Sultanaa, et. al. 2013). Olanrewaju et al. (2006) noted that growth performance also varied with wavelength. LED lighting provides the capability of developing a lighting system that may improve welfare of turkeys reared in confinement.
Bedding Systems and Litter Characteristics. Bedding systems have traditionally been used in meat poultry production (broilers, turkeys) and now for chicken layers in non-cage systems. Bedding type and condition can impact flock performance along with foot and leg condition in all types of poultry.
As bedding costs increase or traditional bedding sources become less available (wood shavings), alternatives are being examined that may also have other attributes in terms of contributions to the production system. Studies in PA have demonstrated the application of willow, poplar, and miscanthus as alternative bedding materials that can be grown on the farm and utilized as vegetative buffers (Hulet et al., 2010; Hile et al., 2012; Patterson et al., 2012). Litter from these birds has also been demonstrated as alternative fuel sources for brooding birds (Patterson et al., 2010; Bardella et al. 2011).
Litter source and condition impacts bird performance and has health and welfare considerations such as footpad dermatitis (Wu and Hocking, 2011). Litter condition exerts an effect on the gaseous and particulate emissions; and bacterial populations. Litter condition is complex, influenced not only by the excretions of the bird but also by external environmental factors (ventilation, temperature, dew point, etc.) (Tasistro et al., 2004). Likewise, there are feed and nutritional factors that affect litter moisture such as by-product inclusion and electrolytes which indicated inclusion of ingredients containing sulfur or chloride resulted in increased litter moisture but did not greatly impact footpad or gait score (Farahat et al., 2013).
Characterization of bedding including new sources and its condition within housing systems has varied considerably and includes measures of foot pad scoring, histology of foot pad, leg problems, particle size, pH, temperature, and moisture (Garcês et al., 2013). A more standard approach to litter assessment should be developed and needs to be correlated with bird condition and well-being.
Housing. Alternative hen housing for egg production is a topic that continues to receive growing interest and attention among U.S. egg producers and those connected to or affected by the egg industry. This development mainly stems from the intention/demand for improving animal welfare, other aspects of production food safety/security, environmental impact, economic efficiency, and ergonomics of the caretakers must also be taken into consideration to ensure a sustainable future of egg supply and the industry. Alternative hen housing not only effects production performance (Karsten et al., 2010) but also the microbiology status of the eggs produced (Jones and Anderson, 2013; Jones et al., 2011, 2012). Work in PA has verified previous studies demonstrating that pasture reared hens require significant amounts of commercial feed (at least 90% of the intake of sister hens raised in cages) to sustain body weight, egg production, and egg size.
Hens maintained in alternative housing systems have a higher rate of keel fractures (Freire et al., 2003; Wilkins et al., 2004; Nicol et al., 2006). Keel fractures have been shown in experimental settings to relate to individual`s birds latency to transition between varying heights to access a food reward suggesting the fractures are a source of pain, particularly as the latency was diminished with administration of an analgesic (Nasr et al., 2012). Evidence was also found that birds with fractures had reduced egg production; however, the trials were conducted in small groups of birds (less than 20) (Nasr et al., 2013). An ex vivo system to replicate collisions that cause fractures and quantify the necessary forces involved has been developed to allow experimental replication of field fractures (Toscano et al., 2013). These results imply effects of keel fractures on the laying hen welfare and productivity, but need to be replicated within a commercial setting.
B. Dietary Manipulations
Feed ingredients. Energy and protein remain the most expensive components of feeding programs and most influence bird performance (growth and utilization) and economics. Concentrated energy sources are being diverted elsewhere and lower energy diets are being fed along with exogenous enzymes to maintain energy utilization. Utilization of byproducts such as corn-derived distillers grains with solubles (DDGS) and alternative grains and protein sources (Burley and Patterson, 2013; Burley et al., 2013a) is becoming more common compared to the traditional corn-soy diet in the Midwest. DDGS has been successfully used in molt diets for laying hens as an example (Mejia et al., 2010, 2011).
Production of DDGS remains strong in the U.S. but recent processing developments have changed the composition of the product by removing the oil to levels less than 7% of the dried product leading to the question of an appropriate energy value for the product. Despite the reduction in fat content, fat content alone is not predictive of metabolizable energy. Prediction equations based on chemical composition and in vivo measures that could be related to NIR would increase confidence in utilization of the product. Several prediction equations have been developed by various researchers for various poultry types (Cozannet et al., 2010; Rochell et al., 2011; Jie et al., 2013; Zhang et al., 2013) except for turkeys and even fewer of these have been tested in feeding trials to confirm their applicability.
Volatility in the price of conventional feed ingredients such as corn and soybean meal for poultry feed and increased biofuel production has and will make different alternative feed sources available. Algae and corn biofuel production may provide cost effective and nutritionally valuable by-products (e.g. algae meal and distillers dried grains with solubles (DDGS)) to the poultry industry. Previous studies have shown that carbohydrases and phytase increased AME (Apparent Metabolizable Energy) and growth performance of the broiler diet with high DDGS (Adeola et al., 2010). It is critical to determine proper inclusions of these by-products into poultry diets and their efficacy when one or more exogenous enzymes are included in the diet.
Feed processing. Feed and feed manufacture represents 60-70% of the investment required to produce poultry. Poultry diets in the U.S. are predominately steam conditioned and pelleted, which entails substantial cost; however, costs may be justified if pellet quality is maintained from the feed mill to the feed pan. Recent interest in the poultry industry has been directed towards determining how current genotypes of broilers respond to pellet quality. When poor quality pellets are transported and conveyed through extensive augured systems of modern broiler and turkey barns, nutrient/ingredient segregation is inevitable and detriments to health and performance are probable. Feed samples have been obtained from four commercial poultry integrators located in regions of the Eastern U.S. and then analyzed for percent pellets, particle size, and specific nutrient compositions that may be affected by segregation. These data have demonstrated that nutrient segregation occurs (Wamsley, 2013, personal communication).
Provision of nutrients. Alternatives to synthetic dietary methionine are needed in the production of organic poultry. Recent reduction in the allowed amounts of synthetic methionine (2, 2, and 3lbs/ton) challenge nutritionists to provide for the growth and production requirements of layers, broilers, and turkeys. Higher protein diets cannot meet the needs of these birds, and when used generate unfavorable environmental and health issues (wet litter, greater ammonia emissions, etc.) for the birds (Burley et al., 2013b).
The current 1994 NRC selenium (Se) requirement is 0.2 µg Se/g for all stages from starter to finishing diets, and for breeding and laying hen diets (NRC, 1994). The minimum Se requirement for male turkey poults is 0.3 µg Se/g three times higher than requirements found in rodents based on liver and gizzard Gpx4 and Gpx1 activities (Sunde and Hadley, 2010). Work has been done to clone and sequence 24 selenoproteins so far (Sunde et al., 2012), as well as the selenocysteine tRNA which was not found in contigs present in shotgun sequencing of the turkey genome (Dalloul et al., 2010). These sequences are being used to develop molecular biomarkers to characterize Se regulation in the turkey. In addition, these studies may help to better define the value of super nutritional Se supplementation (excess Se beyond that needed to meet requirements), which adds expense to animal diets and can contribute to excess excretion of this element in animal waste (Davis et al., 1988).
C. Food Safety and Housing System
In preparation for the EU hen housing transition, researchers explored various aspects of the law in member countries and resulting egg microbiology (De Reu et al., 2005, 2006; Mallet et al., 2006; De Reu et al., 2008; Schwaiger et al., 2008; De Reu et al., 2009; Huneau-Salaün et al., 2009), often presenting conflicting results. Unfortunately, due to the dynamic nature of microbial growth and the differences in husbandry practices and laying hen genetics between the EU and U.S., much of the egg microbial information from the EU cannot be directly applied to U.S. egg production systems (Holt et al., 2011).
Food safety is not the only concern for the U.S. egg industry in reference to microbiological concerns. Environmental and egg microbiology impact not only consumer safety, but also the ability of the U.S. egg industry to meet the requirements of the federal law governing egg production and transportation (FDA, 2009). Salmonella Enteritidis (SE) is a leading foodborne pathogen in the U.S. with many outbreaks in humans traced back to shell eggs. As a result, the implementation of effective strategies for reducing SE in commercial layer flocks has become a critical public health and economic objective. The current FDA Egg Safety Rule and Egg Quality Assurance Programs are based on critical control points and best management practices developed from studies of large flocks (>50,000 hens) conducted in the 1990s, indicating there are opportunities to improve pre-harvest programs to reduce SE contamination (Patterson et. al., 2013). Surveys of the microbial diversity of a variety of commercial egg production systems in Europe have been conducted (De Reu et al., 2006; Schwaiger et al., 2008; De Reu et al., 2009; Huneau-Salaün et al., 2009), but very few controlled commercial-style studies have occurred. In the U.S., researchers have begun to examine the effects of commercial scale alternative egg production systems on environmental and egg microbiology (Jones et al., 2011, 2012). Additionally, Jones and Anderson (2013) have shown that laying hen strain can impact the microbiology of the egg and production environment in various housing systems.
Objectives
-
Energy/resource efficiency. This will include collaborative efforts on feed and fuel energy sources for poultry and facilities by geographical region, facility design, equipment efficiency, management, and modeling energy use in poultry systems.
-
Evaluating commercial poultry production systems. This will include collaborative efforts on the characterization of the performance of conventional, alternative, and organic poultry production systems relative to air and water quality, nutrient management, acoustic environment, and animal health and welfare.
-
Establishing parameters influenced by the production system and strains utilized within the poultry industry. This collaborative research will encompass the areas of poultry nutrition, physiology, behavior, well-being, food safety and quality, and economic evaluation of poultry production systems.
Methods
Objective 1. Energy/resource efficiency. (MS, IA, CT, AR) Studies will establish methods to reduce energy usage and improve resource efficiency in poultry production. This objective will be achieved through studies evaluating: 1) building design and operation, and 2) management practices. The expected results will provide insight into building design and operation to maximize resource efficiency while maintaining a high level of animal welfare allowing for a sustainable poultry enterprise. 1a. Building design including lighting technologies, thermal and non-thermal parameters, and energy consumption. Evaluation of lighting devices (CFL, LED, SSL) will be tested under commercial poultry housing conditions for reliability, light output, energy savings, bird performance, and suitability for various production systems. In addition, laboratory scale tests will be conducted to determine the interactive effects of differing lighting programs (photoperiod and illuminance) and lighting devices on bird performance and physiological response. Energy use in different poultry housing systems for laying hens and broilers will be monitored over extended period (covering different production seasons and stages) and analyzed. Partitioning of energy consumption into classes of equipment or operations will be determined, e.g., ventilation (fans), lighting, feeding systems, and fuel for supplemental heating (if equipped). The energy use will be expressed in the amount of electricity (kWh) or fuel (liter or gallon) per dozen or kg of eggs produced or per kg of live meat birds marketed. Practical means to reduce electricity or fuel usage, reduce energy transfer through the building envelope using heat flux sensors and assessment of radiant heater efficiency will be explored through lab experiments and field verification. 1b. Evaluation of management practices including litter sources, lighting and ventilation programs. Research will be conducted on litter sources for broilers and turkeys as a sustainable cycle, i.e., utilize vegetative buffers (grasses, willow, etc.) as litter sources, generate energy from litter for heating houses, and determine nutritive potential for feeding ash residue. Additionally, drinker systems to improve litter moisture and maintain turkey growth will be evaluated. A range of lighting programs and temperatures will be assessed to determine their impact on heat and moisture production in modern broiler genetics. The thermal parameters and heat flux rates through the chicken surface will be measured in a custom-made wind-tunnel apparatus to allow examination of heat transfer coefficient and water vapor evaporation. The relationship between air velocity, ambient temperature, relative humidity and heat transfer coefficients will be developed. In addition, energy usage for different ammonia control strategies will be assessed. Objective 2. Evaluating commercial poultry production systems (IL, NC, MI, MN, IA, CA) Studies will characterize the performance of conventional, alternative, and organic poultry production systems. The objective will be achieved through examining: 1) nutrient management and impacts on air and water quality, 2) housing environment, 3) food quality and safety, and 4) animal health and welfare. 2a. Nutrient management and impacts on air and water quality. Field studies will be conducted that evaluate the operational characteristics of certain housing features. These features may include, but not limited to, a new ventilation system or strategy, a new manure-drying system or strategy, and a different lighting system. Portable monitoring instrumentation systems will be used in the field studies. Current collaborative, long-term studies concerning indoor air quality (i.e., concentrations of ammonia, carbon dioxide, and particulate matters) and air emissions of conventional cage, enriched colony and aviary housing systems, along with other components (animal welfare, egg quality and safety, food affordability, and worker ergonomics) of the project will continue during this phase of the multi-state project. One particular area is to explore practical ways to reduce ammonia and PM concentrations and emissions of the aviary houses through combination of laboratory tests and field verification. Field production data will be analyzed for alternative egg production systems under different U.S conditions. From the collected information life-cycle analysis will be performed to assess the environmental impact of alternative egg production systems. The Egg Industry Center has recently performed two LCA studies: one on the Midwest U.S. egg industry (Pellieter et al., 2013a), and the other on the advancement of U.S. egg industry in environmental footprint over the past 50 years (Pelletier et al., 2013b). 2b. Housing environment among the different poultry production systems. A thorough literature review will be conducted and methods assessing bedding and litter conditions will be compiled and reviewed. From these methods and others as developed, a new standard method of assessment will be developed and applied to characterizing litter in production systems with turkeys and other poultry. Turkeys will be reared under different environmental conditions, feeds and different bedding types and litter assessed under these different conditions. Measures of well-being will include bird performance, behavior, bone/foot measures, feather scores and gait scores along with carcass quality measures (foot pad score and breast lesion scores at processing), condemnation, and meat quality. Laying hen housing will be investigated with commercial strains of white and brown egg-type chickens. The North Carolina Layer Performance and Management Test (NCLP&MT) has been an ongoing extension project since 1958, examining the impact of commercial strains of laying hens and their selection on performance and feed utilization and for the past 30 years the production environment on performance and feed utilization. Research will be conducted in the NCLP&MT at the Poultry Research Unit of the Piedmont Research Station at Salisbury, NC and Laying Hen Research Facility at Michigan State University. Pullets will be reared in environmentally controlled housing with various combinations of nutritional or management programs that affect the productivity of the layers. Factors could include: light programs, dietary regimen, body weight program, molt techniques, husbandry practices, environment control programs, alternative production environments, and egg handling programs. Productivity will be defined by various measurements of body weight, skeletal structure, feed consumption, immune competence, physiological health, and egg production criteria. The welfare status will be defined by the behavioral profile and hormonal response of the birds. Egg quality will be defined by the USDA egg quality standards, physical shell and membrane measurements, and through microbiological testing of the shell and contents. The potential of poultry manure as an organic fuel source will be evaluated from commercial sources and based upon governmental standards for emissions. The general experimental design will be a factorial arrangement allowing for examination of multiple factors simultaneously. Additionally, the enriched colony cage for laying hens will have resources evaluated including nests, perches, foraging scratch areas, and the effects of the provision of those resources on hen health. Different housing designs and ventilation systems for alternative hen housing systems will be evaluated in commercial production facilities with regards to indoor environment (e.g., uniformity of air distribution) and hen production performance during different production seasons. 2c. Alternative production systems on food safety and quality. Scientists from USDA-ARS, Egg Safety and Quality Research Unit, Athens, GA will collaborate with faculty from North Carolina State University and Michigan State University to examine the food safety and product quality impacts of alternative hen housing systems. Through these collaborations, the microbiological diversity of the eggs, as well as the production environments will be determined. Total aerobic bacteria, Enterobacteriaceae, and yeast and mold populations are enumerated (counted). Total aerobic bacteria are indicators of the overall microbial levels present on and in eggs, as well as the production environment. Enterobacteriaceae is the group of organisms containing most of the human pathogens. Coliforms are also found within the group and are indicators of fecal contamination. Yeast and molds are important organisms to enumerate since they are spoilage organisms. Additionally, the presence of mold growth on or in an egg renders it inedible according to U.S. egg grading guidelines. When considering food safety, human pathogens are always of concern. During the collaborative research efforts assessing the impacts of alternative hen housing systems, the pathogens of concern are Salmonella spp., Campylobacter spp., and Listeria spp. The prevalence of the pathogens in the production environment, as well as on and within eggs, will be determined. The speciation of each pathogen will be determined to assess if certain species of the organisms more readily exist in the various housing systems. The impact of housing systems on egg quality will also be assessed during the collaborative projects. Egg quality analyses to be conducted through the collaborations include: micro crack detection (technology unique to USDA-ARS), shell dynamic stiffness, static compression shell strength, shape index, shell thickness, egg weight, Haugh unit/albumen height, yolk index, vitelline membrane strength and elasticity, apparent viscosity, color measurements, total solids determinations, percent ash, and egg functionality. Additionally, USDA-ARS and the Piedmont Research Station, NC has a pilot egg washing system, which allows for the experimental washing of shell eggs and subsequent analysis. 2d. Analyzing poultry health and welfare in different production systems. The refinement of on-farm welfare assessment/auditing measures, including measures of physical condition; behavior and fearfulness will be used to assess the differences in production systems for all types of poultry. The evaluation of the health and behavior of laying hens and broiler chickens in conventional and organic production systems will be investigated with factors affecting range use in systems with outdoor access. Broiler strains utilized for the live-market and alternative rearing systems will be evaluated for growth and efficiency while commercial stains of turkey toms will be evaluated for growth, feed conversion, and carcass yield. Objective 3. Establishing parameters influenced by the production system and strains utilized within the poultry industry. (CA, CT, GA, IA, IL, IN, MI, MN, MS, NC, NE, WI, Bern Switzerland) Research studies will be conducted to investigate the parameters influenced by the production system or genetic strain used. The objective will be achieved by evaluating the areas including: a) poultry nutrition, b) physiology, c) behavior and well-being, d) food safety and quality, and e) economic implications. Studies can overlap into several areas. 3a. Evaluating poultry nutrition in production systems and genetic strains. The proposed research will cover the breadth of poultry feeding programs by examining feed form, dietary ingredients, feed additives, and individual nutrients. This research will create replicated data to investigate the relationship between pellet quality, nutrient segregation, current meat bird genotypes and bird performance and health. Additionally, identification of bird strain and feed form effects on bird performance and nutrient utilization will be determined. Alternatives are needed to the use of antibiotic growth promoters in diets of broilers and turkeys. Prebiotic and probiotics additives will be evaluated for use in antibiotic free diets by assessing growth efficiency as well as ammonia emissions in broilers and turkeys. Byproduct ingredients (algae meal and reduced-oil dried distillers grains with solubles) will be assessed in poultry diets by inclusion of different dietary levels. General growth performance, meat yield, intestinal development, intestinal enzyme secretion, intestinal microflora, physiological reaction, and production cost will be determined. Metabolizable energy measures will be conducted as appropriate for poultry (TME, AME) and these ME values will be used to incorporate these ingredients in diets fed to turkeys maintained under different environmental (temperature) conditions. The energy value of alternative feed ingredients will be determined as well in laying hen rations. Nutritive organic sources of methionine will be studied for efficiency and feasibility for feeding layers, broilers, and turkeys. The skeletal problems and eggshell quality issues require a systems approach to evaluation of calcium, phosphorus, and vitamin D within the diet. The increased activity in alternative housing systems may impact the nutritional regulation of the skeletal, muscular and cartilaginous portions of the body. Calcium particle size will be evaluated throughout the laying hens life cycle. A better understanding of micro-mineral nutrition will help to refine the specific requirements within poultry diets. Poultry species have unique requirements compared to other animal models and development of specific biomarkers will help to refine minimal requirements and/or identify toxic levels such as being suggested for the model to study selenium nutrition. 3b. Poultry physiology and production systems. Aspects of production systems interact with the physiology of the bird and subsequent performance and well-being. Feather pecking (FP) and cannibalism occur in all the current egg production systems including cage and free range, which is an eminent cause of mortality in untrimmed chickens. Beak trimming (BT) is a common practice to prevent FP and cannibalism. Serotonin and its receptors appear early during prenatal development. Researchers will test the hypothesis that serotonin treatment immediately before egg incubation will affect brain development in chickens and prevent FP and cannibalism. The goal of the study is to develop an animal-friendly method for preventing FP and cannibalism and eliminating BT. Another area of concern in laying hen production systems are bone fractures. Osteoporosis is widespread in todays commercial laying hens and contributes to approximately 20 to 35% of all mortalities during the egg production cycle of caged hens. Bone fractures during production are a huge welfare issue because of the chronic pain these hens may experience. The effect of pullet rearing on skeletal quality during egg laying has not been extensively studied. Perches will be examined for their impact on bone mineralization during rearing. The expected outcome of the project is that due to endocrine response to exercise, pullets using perches during the growing phase will have increased bone mineralization as compared to pullets not using perches and that further use of perches during the adult phase will have no additional benefits in improving bone integrity. Heat stress is a critical factor affecting hen health and production. With the conversion by the egg industry from conventional to enriched cages, perches in enriched cages could be modified to improve hen thermal comfort. Our long-term goal is to identify intervention methods to reduce heat stress in laying hens. Hens will be evaluated for skeletal and foot health, feather quality, egg production traits, feed efficiency, causes of mortalities, body temperature, behavior, and endocrine responses in systems with and without modified perches. High atmospheric levels of CO2 do affect broiler and turkey performance. Previously, research has found levels of 2,500 and 4,000 ppm affected turkey poult metabolism and activity. Thus, experiments will be conducted to test additional CO2 ranges to determine what level CO2 exposure broilers and turkeys tolerate and still perform to their maximum capacity. The effects of varying wavelength of light will be examined using male turkeys. They will be reared under different wavelengths of red, blue, and green and different intensities of light. Growth rate, feed intake, gait score, feather score and behavior will be evaluated. Studies will be performed during the brood and grow finish phase of turkey production. Similar studies will be conducted in broilers evaluating different lighting programs during incubation and post-hatch. 3c. Behavior and well-being influenced by production system and genetic strains. The proposed work seeks to establish whether the observed relationship between latency to transition and keel fractures observed by others can be identified in a commercial aviary using newly available technology that allows tracking of individual birds. Productivity will be monitored of individual birds using colored feed dye allowing egg productivity to be monitored and compared across birds with and without fractures. A tracking system in combination with developed accelerometers worn by the birds will be used to identify locations in the aviary where high energy collisions occur, specifically, those exceeding values using ex vivo testing to cause fracture (Toscano et al., 2013). Several experiments are proposed to examine keel damage and the relationship with bird productivity, mobility, and collisions as a causal factor. To quantify impacts of keel damage on productivity, birds will be palpated and then administered a dyed capsule to allow for identification of eggs from hens with keel damage or not. Assessments of mobility and collisions will be made using custom designed surveillance equipment and a tri-axial accelerometer designed to be mounted on the keel of the bird allowing for unobstructed and normal locomotion. A series of lab experiments will be conducted that examine the responses of pullets and layers of different breeds to various housing or production management factors. These influencing factors will include, but not limited to, bird stocking density, perch space and configuration in an enriched colony setting, cage/colony configuration, and lighting intensity and light type. RFID sensing and machine vision technologies will be employed to aid in the behavioral measurements. Euthanasia techniques (captive bolt non-penetrating, and gas inhalation) in poultry production systems will be evaluated for effectiveness and future improvements. Studies to evaluate what management factors affect the incidence of pendulous crops in turkeys will be studied. Pendulous crop incidence can affect bird health and increase contamination in the processing plant. Research to understand what factors affects incidence will be helpful in improving bird health. 3d. Food safety and quality differences in production systems and genetic strain influence. Plant derived antimicrobials such as trans-cinnamaldehyde, carvocrol, capryllic acid and eugenol will be administered to commercial laying hens and broiler chickens in their feed and the birds will then be tested for levels of salmonella enteritidis in the ovaries, liver, muscle, crop and ceca. 3e. Economic implications of different production systems. Where appropriate, production income and costs will be calculated and compared for research previously described. These analyses will assist producers when implementing the research knowledge generated from these studies. The methodological approach frequently used in economics to evaluate projects or policy proposals is referred to as benefit-cost analysis. The approach relies on measuring benefits and costs of different scientific or engineering projects or regulation policy proposals, and if the benefits are larger than the costs, or if the so-called benefit-cost ratio is greater than 1, the project or policy passes the test and is recommended for adoption or approval. The proposed methodology for conducting the benefit-cost analysis relies on the standard enterprise budgeting techniques (Boehlje and Eidman, 1984). A typical enterprise budget reflects two sides of the profitability equation: revenues and costs. The standard approach for estimating economic benefits (revenue side) is to look at the change in prices consumers would be willing to pay for the new or improved product or service. For example, the potential economic benefits associated with improved living conditions in confined animal production facilities would materialize if consumers’ willingness to pay for this additional attribute would increase as the result of consumers' perception or as the result of some measurable product quality improvement. In most of the work in this project we will focus primarily only on the cost side of the profit equation, whereas the imputed values for total revenue will be calculated based on the break-even price, which implicitly assumes the zero-profit condition, for details see Vukina et al. (2014). The cost estimation methodology typically involve three steps. In the first step, the baseline cost structure and the break-even price for the relevant commercial enterprise is established. In the second step, we analyze the impact of the new technologies or practices on the baseline cost structure. All aspects of the cost of production that could have an effect on the representative operation’s baseline costs will be quantified to obtain the new cost structure and the new break-even price. The comparison of the new technology or practice and old (baseline) break-even prices will be expressed as a percentage increase or decrease in the break-even price relative to the baseline. In the third and final step, we try to calibrate the obtained results to capture possible economies of scale associated with different commercial sizes of targeted operations. To the extent that the analyzed project could have wider market implications, we rely on the partial equilibrium framework.Measurement of Progress and Results
Outputs
- Data is published in peer review high impact journals and presented at major scientific venues including Poultry Science and the World Poultry Science conferences
- Data and results are translated for lay publication in extension bulletins, articles for popular press and other medium made accessible to stakeholders including industry and the public. Periodic briefings/presentations and feedback from stakeholders.
- Enhanced collaborative research and networking by engaging members in development of research proposals for submission to federal agencies to tackle the complex problems.
- Successful extramural competitive funding obtained from industry and government programs will target specific collaborative research goals outlined in the project and to support graduate student and post-doctoral trainees
- Resource and energy efficiencies will improve producer economic situations
- Output 6 Data generated from nutrition trials will be utilized by producers for feed formulations; Output 7 Producers will have access to data related to poultry well-being to supplement decisions related to management practices; Output 8 Human capital from the graduate student and post-doctoral training programs supported by this project and entering the professional workforce; Output 9 Industry adoption of recommendations emanating from the recommendation of research findings for use in commercial production and allied industries; Output 10 Annual progress reports and published material are made available to the public and stakeholders through various media including a website developed for the project
Outcomes or Projected Impacts
- Improved productivity (feed conversion, weight gain, dozen of eggs produced)
- Reductions in <i>Salmonella</i> spp. shedding as a result of feeds or management practices
- Adoption of new technologies reducing energy consumption in poultry facilities
- Standards will be developed for assessing and comparing litter substrates in poultry housing that allow producers to make sound decisions that improve animal and human well-being
- Management recommendations on alternative housing systems for laying hens that will assist producers in making sound business decisions on which systems are most suitable to their operations; and identify best practices to be adopted to allow these systems to function to full potential
- Outcome/Impact 6 Recommendations for LED lighting for turkeys based on well-being and performance that not only reduce energy use footprint but improve turkey performance and captures operation efficiencies
Milestones
(2014): <li>Complete analysis of the implications of organic paddock rotation following cattle on productivity and egg quality</li> <li>Paddock density influence on forage cover</li> <li>Quantify energy partitioning in commercial broiler houses (1-3 flocks)</li> <li>Quantify effective heating areas for commercial radiant brooders</li> <li>Develop an efficiency model for the improvement of attic inlet design</li> <li>Complete research on factors influencing incidence of pendulous crop in turkeys</li> <li>Complete sequencing of turkey selenoprotein transcriptome and development of qRTPCR primers; conduct male turkey poult study</li> <li>Conduct studies to determine the effects of atmospheric CO2 on broiler and turkey production performance during the brooding phase</li> <li>Complete trial 1 of broiler heat and moisture production for modern genetics</li> <li>Comparing the impact of three forage substrates on egg microbiology in an aviary production system</li> <li>Development of a reliable, rugged, and reasonable apparatus to monitor bird motion within non-caged (group housed, barn-reared) housing systems and while ranging (in extensive systems)</li> <li>Publication of alternative production system on prevalence of susceptible and antibiotic-resistant <i>Salmonella</i> on conventional farms that transitioned to organic practices</li>(2015): <li>Influence of density on laying hens of divergent strains in the enriched environmental housing systems</li> <li>Conduct research on the effectiveness of non-penetrating captive bolt instruments on rendering immediate unconsciousness and death for poultry</li> <li>Quantify energy partitioning in commercial broiler houses (4-6 flocks)</li> <li>Complete analysis of male turkey poult selenium study; conduct female turkey poult study</li> <li>Complete trials 2-3 of broiler heat and moisture production for modern genetics</li> <li>Recommendations will be made to the broiler and turkey industry as to acceptable levels of atmospheric CO2 during the brooding phase based on 2014 studies</li> <li>Complete study comparing the impact of five housing systems on environmental and egg microbiology utilizing a single strain of laying hen</li> <li>Thorough understanding of bird movement within non-caged (group housed, barn-reared) housing systems in relation to resources and management practices</li>
(2016): <li>Quantify energy partitioning in commercial broiler houses (7-9 flocks)</li> <li>Finalize standard methods to compare commercial infrared brooders</li> <li>Complete analysis of female turkey poult selenium study; initiate use of selenium molecular biomarker approach for assessment of selenium status/requirements in commercial flocks</li> <li>Complete trial 4 of broiler heat and moisture production for modern genetics</li> <li>Develop digital models to determine convective heat transfer coefficients</li>
(2017): <li>Summarize energy partitioning studies</li> <li>Conduct turkey studies on efficiency of new low moisture nipple drinkers on performance and growth</li> <li>Complete analysis of non-penetrating captive bolt studies on animal well-being of poultry</li> <li>Complete analysis of selenium molecular biomarkers in commercial flocks; expand these studies to other commercial flocks</li> <li>Complete single bird simulations to test heat transfer coefficients</li>
(2018): <li>Continue analysis of selenium molecular biomarkers in commercial flocks; expand use of biotechnology/bioinformatics approaches to development of super nutritional and toxic nutrient biomarkers in poultry</li> <li>Genetic, management, and nutritional effects on incidence of pendulous crop will be reported</li> <li>Complete group bird simulations to test heat transfer coefficients</li> <li>Comprehensive understanding of changes of how bird movement within various housing system affects and relates to bird welfare and behavior</li>
(19):li>Conduct initial study using biomarkers for super nutriti
Projected Participation
View Appendix E: ParticipationOutreach Plan
Study findings will be disseminated in a timely manner to the academic communities, industry stakeholders, and the general public through press releases, web publications, extension reports, presentations at professional conferences and industry educational workshops (e.g., Annual Industry Issues Forum organized by the Egg Industry Center, www.eggindustrycenter.org), extension publications (e.g., Animal Industry Report produced at ISU, NCLP&MT Reports at http://poultry.ces.ncsu.edu/layer-performance/), graduate student theses, and peer-reviewed journal articles. While some of the outreach will be passive (user identifies information through web searching or other means), other outreach will be active (planned "events"). The "events" will tend to be planned by the Extension specialists participating in the project including seminars, field days, and workshops at which results of the research technologies will be discussed/disseminated to poultry producers. On-farm demonstrations will be conducted when feasible to allow farmers to see firsthand the results of the research in conjunction with established producer education programs. Members of the group will identify relevant information to share with interested clientele groups within their states or regions. Engagement of the poultry industry stakeholders (United Egg Producers, National Chicken Council, National Turkey Federation, US Poultry and Egg, etc.) will allow for input on relevance of research being conducted and provide feedback on strategic ways to actively share information as well as provide ideas on future research topics. We believe a partnership between industry, government, and academia must exist to improve poultry production systems and well-being.
Organization/Governance
The Technical Committee is responsible for the planning and supervision of the Multi-State Research Project. The membership of this committee shall consist of an Administrative Advisor, a technical representative of each participating agency or experiment station, and representative of the USDA Cooperative States Research Service. Each participating agency or experiment station is entitled to one vote. The Technical Committee shall be responsible for review and acceptance of contributing projects, preparation of reviews, modification of the regional project proposal, and preparation of an annual report. Annual written reports will be prepared by each technical committee member and distributed at the annual meeting. Annual reports will be complied and distributed to Technical Committee members and Agricultural Experiment Station Directors. The Technical Committee will meet yearly and conduct an election for the office of Junior Executive. The position should alternate between Poultry Scientists and Agricultural Engineers. The person elected to serve as Junior Executive will rotate through the remaining offices of Senior Executive and Secretary and will serve as Chair in the fourth year. All voting members of the Technical Committee are eligible for office. The Chair prepares the meeting agenda and presides at meetings. The Chair is responsible for preparation of the annual report. The Secretary records minutes and assists the Chair. The Senior and Junior executives help with policy decisions and nominations. The Technical Committee functions as a unit with sub-committees formed as necessary. i.e., preparing nominations for elections.
Literature Cited
Adeola, O., J. A. Jendza, L. L. Southern, S. Powell, and A. Owusu-Asiedu. 2010. Contribution of exogenous dietary carbohydrases to the metabolizable energy value of corn distillers grains for broiler chickens. Poult. Sci. 89:1947-1954.
Bardella, A. J., P. H. Patterson, M. Hulet, and T. L. Cravener. 2011. Alternative fuel for brooding turkey poults: Bird and environmental impacts. Poult. Sci. 90(E-Suppl. 1):54.
Boehlje, M. D., and V. R. Eidman. 1984. Farm Management. John Wiley and Sons, New York.
Burley, H. K., and P. H. Patterson. 2013. Brazil nut meal and inedible egg as alternatives to synthetic methionine in organic laying hen diets. Poult. Sci. 92(E-Suppl. 1):210.
Burley, H. K., R. M. Hulet, and P. H. Patterson. 2013a. Alternative ingredients to synthetic methionine for organic broiler diets. Poult. Sci. 92(E-Suppl. 1):209.
Burley, H. K., P. H. Patterson, and M. A. Elliot. 2013b. Effect of a reduced crude protein, amino acid-balanced diet on hen performance, production costs, and ammonia emissions in a commercial laying hen flock. J. Appl. Poult. Res. 22:217-228.
Cozannet, P., M. Lessire, C. Gady, J. P. Metayer, Y. Primot, F. Skiba, and J. Noblet. 2010. Energy value of wheat dried distillers grains with solubles in roosters, broilers, layers, and turkeys. Poult. Sci. 89:2230-2241.
Dalloul, R. A., J. A. Long, A. V. Zimin, L. Aslam, K. Beal, L. A. Blomberg, P. Bouffard, D. W. Burt, and O. Crasta. 2010. l. Multi-platform next-generation sequencing of the domestic turkey (Meleagris gallopavo): genome assembly and analysis. PLoS Biol. 8:e1000475.
Davis, E. A., K. J. Maier, and A. W. Knight. 1988. The biological consequences of selenium in aquatic ecosystems. California Agriculture 18-29.
De Reu, K., K. Grijspeerdt, M. Heyndrickx, M. Uyttendaele, J. Debevere, and L. Herman. 2006. Bacterial shell contamination in the egg collection chains of different housing systems for laying hens. Br. Poult. Sci. 47:163-172.
De Reu, K., K. Grijspeerdt, M. Heyndrickx, J. Zoons, K. De Baere, M. Uyttendaele, J. Debevere, and L. Herman. 2005. Bacterial eggshell contamination in conventional cages, furnished cages and aviary housing systems for laying hens. Br. Poult. Sci. 46:149-155.
De Reu, K., W. Messens, M. Heyndrickx, T. B. Rodenburg, M. Uyttendaele, and L. Herman. 2008. Bacterial contamination of table eggs and the influence of housing systems. Worlds Poult. Sci. 64:5-19.
De Reu, K., T. B. Rodenburg, K. Grijspeerdt, W. Messens, M. Heyndrickx, F. A. M. Tuyttens, B. Sonck, J. Zoons, and L. Herman. 2009. Bacteriological contamination, dirt, and cracks of eggshells in furnished cages and noncage systems for laying hens: An international on-farm comparison. Poult. Sci. 88:2442-2448.
Farahat, M. H., W. M. Abdel-Razik, E. I. Hassanein, and S. L. Noll. 2013. Effect of phytase supplementation to diets varying in chloride level on performance, litter moisture, foot pad score, and gait score of growing turkeys. Poult. Sci. 92:1837-1847.
FDA. 2009. Prevention of Salmonella Enteritidis in Shell Eggs During Production, Storage, and Transportation; Final Rule. http://edocket.access.gpo.gov/2009/pdf/E9-16119.pdf. Accessed August 30, 2012.
Frame, D. D., R. E. Buckner, and G. L. Anderson. 2010. Causes and control of spontaneous cardiomyopathy or roundheart disease in Utah turkeys. Fact sheet. Utah State University Cooperative Extension.
Freire, R., L. J. Wilkins, F. Short, and C. J. Nicol. 2003. Behavior and welfare of individual laying hens in a non-cage system. Br. Poult. Sci. 44:22-29.
Garcês, A., S. M. S. Afonso, A. Chilundo, and C. T. S. Jairoce. 2013. Evaluation of different litter materials for broiler production in a hot and humid environment: 1. Litter characteristics and quality. J. Appl. Poult. Res. 22:168-176.
Green, A. R., and H. Xin. 2009a. Effects of stocking density and group size on heat and moisture production of laying hens under thermoneutral and heat challenging conditions. Transactions of the ASABE 52:2027-2032.
Green, A. R., and H. Xin. 2009b. Effects of stocking density and group size on thermoregulatory responses of laying hens under heat challenging conditions. Transactions of the ASABE 52:2033-2038.
Green, A. R., I. Wesley, D. W. Trampel, and H. Xin. 2009. Air quality and hen health status in three types of commercial laying hen houses. J. Appl. Poult. Res. 18:605-621.
Hayes, M. D., H. Xin, H. Li, T. A. Shepherd, and J. P. Stinn. 2013a. Electricity and fuel usage of aviary layer houses in the Midwestern USA. Applied Engineering in Agriculture (accepted).
Hayes, M. D., H. Xin, H. Li, T. A. Shepherd, Y. Zhao, and J. P. Stinn. 2013b. Ammonia, greenhouse gas, and particulate matter concentrations and emissions of aviary layer houses in the Midwestern USA. Transactions of the ASABE 56:1921-1932.
Hayes, M. D., H. Xin, H. Li, T. A. Shepherd, Y. Zhao, and J. P. Stinn. 2013c. Heat and moisture production of Hy-Line brown hens in aviary houses in the Midwestern United States. Transactions of the ASABE 56:753-761.
Hile, M. L., E. F. Wheeler, and P. H. Patterson. 2012. Ammonia emissions from chopped willow vs. pine shavings as bedding for broiler chickens. (Presentation for the 9th International Livestock Environment Symposium sponsored by ASABE, Valencia, Spain.
Holt, P. S., R. H. Davies, J. Dewulf, R. K. Gast, J. K. Huwe, D. R. Jones, D. Waltman, and K. R. Willian. 2011. The impact of different housing systems on egg safety and quality. Poult. Sci. 90:251-262.
Hulet, R. M., P. H. Patterson, T. L. Cravener, and T. A. Volk. 2010. Alternative bedding for broilers: From vegetative buffers to fuel. Poultry 89(E-Suppl 1):42.
Huneau-Salaün, A. M. Chomaly, S. Le Bouquin, F. Lalande, I. Petetin, S. Rouxel, V. Michel, P. Fravalo, and N. Rose. 2009. Risk factors for Salmonella enterica subsp. enterica contamination in 519 French laying hen flocks at the end of the laying period. Prev. Vet. Med. 89:51-58.
Jie, Y. Z., J. Y. Zhang, L. H. Zhao, Q. G. Ma, and J. Cheng. 2013. The correlationship between the metabolizable energy content, chemical composition and color score in different sources of corn DDGS. Journal of Animal Science and Biotechnology 4:25.
Jones, D. R., and K. E. Anderson. 2013. Housing system and laying hen strain impacts on egg microbiology. Poult. Sci. 92:2221-2225.
Jones, D. R., K. E. Anderson, and J. Y. Guard. 2012. Prevalence of coliforms, Salmonella, Listeria, and Campylobacter associated with eggs and the environment of conventional cage and free-range egg production. Poult. Sci. 91:1195-1202.
Jones, D. R., K. E. Anderson, and M. T. Musgrove. 2011. Comparison of environmental and egg microbiology associated with conventional and free-range laying hen management. Poult. Sci. 90:2063-2068.
Karsten, H. D., P. H. Patterson, C. Stout, and G. Crews. 2010. Vitamins A and E, and fatty acid composition of the eggs of pastured and caged hens. J. Renew. Ag. & Food Sys. 25:45-54.
Liang, Y., G. T. Tabler, T. A. Costello, I. L. Berry, S. E. Watkins, and Y. V. Thaxton. 2013. Cooling broiler chickens by surface wetting: indoor thermal environment, water usage and bird performance. Applied Engineering in Agriculture (accepted).
Mallet, S., V. Guesdon, A. M. H. Ahmed, and Y. Nys. 2006. Comparison of eggshell hygiene in two housing systems: Standard and furnished cages. Br. Poult. Sci. 47:30-35.
Marchewka, J., T. T. N. Watanabe, V. Ferrante, and I. Estevez. 2013. Review of the social and environmental factors affecting the behavior and welfare of turkeys (Meleagris gallopavo). Poult. Sci. 92:1467-1473.
Mejia, L., E. T. Meyer, D. L. Studer, P. L. Utterback, C. W. Utterback, C. M. Parsons, and K. W. Koelkebeck. 2011. Evaluation of limit feeding varying levels of distillers dried grains with solubles in non-feed-withdrawal molt programs for laying hens. Poult. Sci. 90:321-327.
Mejia, L., E. T. Meyer, P. L. Utterback, C. W. Utterback, C. M. Parsons, and K. W. Koelkebeck. 2010. Evaluation of limit feeding corn and distillers dried grains with solubles in non-feed withdrawal molt programs for laying hens. Poult. Sci. 89:386-392.
Nasr, M. A. F., W. J. Brown, G. Capler, B. Hothersall, J. C. Murrell, and C. J. Nicol. 2013. Positive affective state induced opioidanalgesia in laying hens with bone fractures. Appl. Anim. Behav. Sci. 147:127-131.
Nasr, M. A. F., J. Murrell, L. J. Wilkens, and C. J. Nicol. 2012. The effect of keel fractures on egg-production parameters, mobility and behavior in individual laying hens. Anim. Welf. 21:127-135.
National Research Council. 1994. Nutrient Requirements of Poultry., 9 ed., Washington D.C., National Academy Press.
Nicol, C. J., S. N. Brown, E. Glen, S. J. Pope, F. J. Short, P. D. Warriss, P. H. Zimmerman, and L. J. Wilkins. 2006. Effects of stocking density, flock size and management on the welfare of laying hens in single-tier aviaries. Br. Poult. Sci. 47:135-146.
Olanrewaju, H. A., J. P. Thaxton, W. A. Dozier, J. Purswell, W. B. Roush, and S. L. Branton. 2006. A review of lighting programs for broiler production. Int. J. Poult. Sci. 5:301-308.
Patterson, P. H., R. M. Hulet, and D. E. Buffington. 2010. Turkey litter as an alternative fuel demonstration vs. propane on a Pennsylvania turkey farm. Proc. National Poultry Waste Management Symposium. Auburn University, Auburn, Al.
Patterson, P. H., R. M. Hulet, T. Cravener, M. Hile, and E. Wheeler. 2012. A comparison of pine shavings vs. chopped willow as bedding for rearing broiler chickens. Poult. Sci. 91(E-Suppl 1):203.
Patterson, P. H., K. Venkitanarayanan, and S. Kariyawasam. 2013. Introduction: Reducing Salmonella enteritidis contamination of shell eggs. J. Appl. Poult. Res. 22:(submitted).
Pelletier, N., M. Ibarburu, and H. Xin. 2013a. A carbon footprint analysis of egg production and processing supply chains in the Midwestern United States. J. Cleaner Production. 54:108-114.
Pelletier, N., M. Ibarburu, and H. Xin. 2013b. Comparative assessment of the environmental footprint of the U.S. egg industry in 1960 and 2010. Poult. Sci. 92:(accepted).
Reilly, W. M., K. W. Koelkebeck, and P. C. Harrison. 1991. Performance evaluation of heat-stressed commercial broilers provided water-cooled floor perches. Poult. Sci. 70:1699-1703.
Rochell, S. J., B. J. Kerr, and W. A. Dozier III. 2011. Energy determination of corn co-products fed to broiler chicks from 15 to 24 days of age, and use of composition analysis to predict nitrogen-corrected apparent metabolizable energy. Poult. Sci. 90:1999-2007.
Schwaiger, K., E. M. V. Schmid, and J. Bauer. 2008. Comparative analysis of antibiotic resistance characteristics of gram-negative bacteria isolated from laying hens and eggs in conventional and organic keeping systems in Bavaria, Germany. Zoonoses Public Hlth. 55:331-341.
Sultanaa, S., R. Hassana, H. S. Choeb, and K. S. Ryua. 2013. The effect of monochromatic and mixed LED light colour on the behaviour and fear responses of broiler chicken. Avian Biology Research 6:207-214.
Sunde, R. A., and K. B. Hadley. 2010. Phospholipid hydroperoxide glutathione peroxidase (Gpx4) is highly regulated in male turkey poults and can be used to determine dietary selenium requirements. Exp. Biol. Med. 235:23-31.
Sunde, R. A., G. R. Sunde, C. M. Sunde, M. L. Sunde, and J. K. Evenson. 2012. Cloning, sequencing, and expression of a subset of selenoprotein transcripts in the turkey (Meleagris gallopavo). FASEB J. 26:241.
Tao, X., and H. Xin. 2003. Surface wetting and its optimization to cool broiler chickens. Transactions of the ASABE 46:483-490.
Tasistro, A. S., D. E. Kissel, and P. B. Bush. 2004. Spatial variability of broiler litter composition in a chicken house. J. Appl. Poult. Res. 13:29-43.
Toscano, M. J., L. J. Wilkins, G. Millburn, K. Thorpe, and J. F. Tarlton. 2013. Development of an ex vivo protocol to recreate the keel damage resulting from collisions in laying hens. PLoS ONE 8:e66215. doi:10.1371/journal.pone.0066215.
Vukina, T., K. Anderson, and M. K. Muth. 2014. Economic impacts of proposed changes in living conditions for laying hens under the National Organic Program. J. Appl. Poult. Res. 23:80-93.
Wilkins, L. J., S. N. Brown, P. H. Zimmerman, C. Leeb, and C. J. Nicol. 2004. Investigation of palpation as a method for determining the prevalence of keel and furculum damage in laying hens. Vet. Rec. 155:547-549.
Wu, K., and P. M. Hocking. 2011. Turkeys are equally susceptible to foot pad dermatitis from 1 to 10 weeks of age and foot pad scores were minimized when litter moisture was less than 30%. Poult. Sci. 90:1170-1178.
Xin, H., H. Li, R.T. Burns, R. S. Gates, D. G. Overhults, and J. W. Earnest. 2009. Use of CO2 concentration difference or CO2 balance to assess ventilation rate of broiler houses. Transactions of the ASABE 52:1353-1361.
Zhang, S. J., C. H. Zhu, J. Guo, Q. P. Tang, H. F. Li, and J. M. Zou. 2013. Metabolizable energy and fiber digestibility of uncommon feedstuffs for geese. Poult. Sci. 92:1812-1817.
Zhao, Y, H. Xin, T. A. Shepherd, M. D. Hayes, and J. P. Stinn. 2013a. Modeling ventilation, balance temperature and supplemental heat requirement in alternative versus conventional laying-hen housing systems. Biosystems Engineering 115:311-323.
Zhao, Y., H. Xin, T. A. Shepherd, M. D. Hayes, J. P. Stinn, and H. Li. 2013b. Thermal environment, ammonia concentrations and ammonia emissions of aviary houses with white laying hens. Transactions of the ASABE 56:1145-1156.