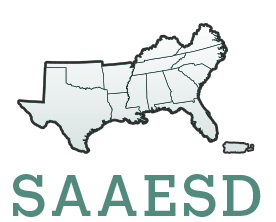
S1032: Animal Production Systems: Synthesis of Methods to Determine Triple Bottom Line Sustainability from Findings of Reductionist Research
(Multistate Research Project)
Status: Inactive/Terminating
S1032: Animal Production Systems: Synthesis of Methods to Determine Triple Bottom Line Sustainability from Findings of Reductionist Research
Duration: 10/01/2013 to 09/30/2018
Administrative Advisor(s):
NIFA Reps:
Non-Technical Summary
Statement of Issues and Justification
As a result of population growth and increases in caloric intake associated with increasing income to expend on food (FAO, 2008), food demand is expected to increase by 70% by year 2050. The demand for animal protein is expected to outpace the growth in total food consumption. However, at the same time, the quantity and quality of available land, fresh water, and energy resources are declining. Furthermore, consumers increasingly want to know how their food is produced, and as they learn, their evolving product preferences create demand for different production practices with respect to (for example) food safety, nutrition, animal welfare, and environmental protection. Balancing accelerating global demand for animal protein with finite production resources, vulnerable environments and ecosystems, economic viability of allied industries and surrounding communities, and social acceptance of food-production practices requires an approach unlike what has been used in the past. Results from reductionist research that addressed individual or isolated components of livestock- and poultry-production systems must now be integrated in ways that reflect the complexity of the systems as a whole.
A systematic and holistic approach to livestock production is the only possible means of continuously meeting global food needs while 1) protecting natural resources such that soil health, water quality and quantity, species diversity, air quality, and climate homeostasis are sustained 2) producing animal products in a manner that is socially acceptable to consumers, and 3) ensuring continued financial solvency of farm operations. The challenges faced by the livestock industry are complex and intertwined. Therefore, approaches to addressing the Triple Bottom Line (TBL) of sustainability that is, sustainability as expressed in the ensemble of planet (environmental), people (social), and profit (economic) subsystems (Elkington, 1997) must be comprehensive and reveal the broader, system-wide impacts of decisions.
At our 2001 annual meeting in San Antonio, co-hosted by the National Center for Manure and Animal Waste Management, we began to recognize that the tremendous, technical progress our members had made over the past decades in reducing waste streams and making more efficient, beneficial use of manure and wastewater from animal-confinement facilities was reaching, or perhaps had already reached, a pivotal phase. Up to then, the primary function of our multistate research committee (then known as S-1000) had been to report to one another on that component-focused, reductionist research, the tacit assumption being that efficiency improvements in various processes were leading the livestock and poultry industries toward ever-increasing sustainability. Around that time, however, we were beginning to observe various forms of evidence that our efficiency-centered view of livestock and poultry systems was not well suited to explaining a number of perverse effects we were seeing from efficiency gains in other industries. We saw irrigation efficiencies approaching 100% in the Great Plains, but aquifer depletion was accelerating. Clearly, efficiency gains in discrete processes, as impressive as they were, have not consistently led us to greater sustainability. In some cases, in fact, increased efficiency was simply allowing finite resources to be accessed more easily by a broader range of newly deployed processes (highly efficient in their own rights) that had not been involved in these systems before or extending the useful life of other resources. The result in those cases was greater usage of finite resources, not lesser usage; and with the specter of Peak Oil and related geophysical limitations emerging in the popular awareness; such a result could not reasonably be considered a contribution to greater sustainability.
In retrospect, we should have predicted that efficiency gains might lead to such perverse outcomes in some cases, especially in the agricultural systems that give focus to our work. But in order to predict such outcomes, we would have had to be listening closely to our colleagues in macroeconomics, ecology, the social sciences, and system dynamics. From macroeconomists, we might have learned that humans respond dynamically and self-interestedly to changes in their economic surroundings. From the ecologists, we might have learned that biological systems can be driven by external subsidies (e. g., fossil energy) to levels of productivity that lead to ecosystem collapse. From the system dynamicists, we might have learned that feedback, delays, and other structural attributes of a system often have as much or more to say about the systems evolution as the individual processes themselves. Throw in the specialized, culturally contingent dynamics associated with human agency, the social scientists might have warned us, and we should be prepared for indeterminate, perverse outcomes in even the simplest, most isolated communities.
Trans-disciplinary and non-traditional research teams are needed due to the technical complexity of these challenges and the growing social aspects of livestock production. Therefore, integration of our efforts among our traditional participants (continuation of previous work on chemical, physical and biological changes occurring in animal facilities from feeding animals through manure generation, treatment, storage and ultimately utilization) and an expansion of participants to include additional colleagues is a key aspect of this proposal to better synthesize scientific findings and aid in policy development. Individually, we will continue to conduct fundamental and applied research that aligns with our specific expertise, similar to what was done previously in S-275, S-1000 and S-1032. Under this proposed project, however, we will contribute our individual findings to the larger project team to integrate findings beyond what any of us could do independently.
Figure 1 (attached) is a causal-loop diagram (CLD) in which animal-protein production systems are embedded within the conceptual environments of triple-bottom-line (TBL) sustainability. Traditionally, our membership has conducted its research within a small area within the CLD. However, changes/decisions within any given area create intended and unintended changes throughout the CLD. These changes are often difficult to identify unless one is aware of and looking for broader impacts. The relative density of interactions in the environmental and economic sustainability sections of the CLD, together with the relative scarcity of interactions in the social sustainability section, reflects the need of our Multistate Research Committee to establish constructive, long-term collaboration with researchers in the social sciences and related disciplines.
Through the efforts described below we propose to evaluate more comprehensively how animal protein production practices impact all facets of the CLD by providing a more integrated view of the system. The long-term goal of the project team is to identify strategies to optimize animal protein production by balancing environmental, social, and economic drivers and effects. The overall objective of this proposal is to construct and develop an increasingly quantitative framework that conveys the system-wide impacts of decisions and the tradeoffs that result from scenarios under consideration. These tradeoffs will include environmental, social, and economic impacts.
Related, Current and Previous Work
Zering et al (2012) summarized the state of water and land use associated with U.S. animal agriculture. According to those authors, who also summarize other work, we are approaching carrying capacity of the planet with our use of land and water. Representing experts in poultry, beef, dairy, and swine production they highlight emerging regulatory, non-regulatory, management, and research approaches &:
(1) a focus on policy transitions to prevent economic damage to producers and consumers; (2) environmental management programs, including goal setting, monitoring, and reporting; (3) the life cycle approach, which provides a comprehensive analysis of effects and a basis for efficient minimization of these effects; and (4) the systems approach, which considers the interdependent effects of livestock production decisions on air emissions, water quality, public health, the economy, and other issues.
Past research, including research conducted as part of the previous multistate research project (S-1032), has demonstrated the local impacts of various production practices and mitigation strategies. These efforts have gone a long way to identify technological and management approaches to support improvements in TBL sustainability of animal protein production. By design, research conducted to date has focused within an area of the CLD and not focused in a systematic way that considers most or all consequences of implementation of alternative technological and management approaches. Some animal protein production practices are expensive and would eventually increase the cost of food (e.g., municipal-type waste treatment systems for animal manure); other practices trade improvements in specific parameters (i.e. animal welfare, improved soil quality, or lower input requirements) for reduced overall agricultural productivity. The cumulative effect of any combination of alternative production practices must be studied holistically in terms of food supply and demand, economic impact on producers and consumers, social impacts such as jobs and quality of life, as well as reduced environmental impact and resource depletion. At present, the system tools needed for such holistic evaluation are unavailable or inaccessible to the researchers exploring mitigation and sustainable options. Intensive production of animal protein results in loss of ecosystem services such as nutrient mitigation by local waterways, biodiversity, water purification, soil formation, flood mitigation, carbon sequestration and others (Costanza et al. 1997; Robinson and Sutherland 2002). The opportunity thus exists for researchers, policy makers and producers to find ways to increase production while limiting harmful effects of that production on ecosystems and society (Rockstrom, J., et al. 2009). A primary objective of this new project is to evaluate effectiveness of new techniques of production, management or resource recovery (area(s) within the CLD). Yet, the broader impacts on biodiversity, ecosystem services and overall food production (the entire CLD) are complex and must be accomplished to adequately evaluate the TBL. The latter areas of focus are particularly time consuming, expensive, and require collaboration with scientists accomplished in different skill sets. Literature from several fields offers insights and methodology that can be applied to the interaction of agricultural production, ecosystem services, and social acceptance of both the products of production and the systems used to accomplish that production. Those techniques include multiple network analysis (Snyder and Kick, 1979), ecological network analysis (Fiscus, 2009) (Fath et al., 2007), concepts from economic and ecological resiliency (Goerner et al., 2009; Ulanowicz et al., 2009), system dynamics (Sterman, 2000), game theory (Gibbons, 1992), and structural equation modeling (Schumacker and Lomax, 2010).
A CRIS database search revealed eight regional projects tangentially related to the proposed replacement project: SCC081, Sustainable Small Ruminant Production in SE US, whose objectives are more closely related to rearing of small ruminant systems (sheep and goats); W 1012; Improving Ruminant Use of Forages in Sustainable Production Systems for the Western US whose objectives focus on the use of interaction between the animal and forage systems; WERA1014 Intensive Pasture Management for Sustainable Livestock Production in the Western US, with objectives focused on the management of pastures; NC 2042 and NC 1042Management Systems to Improve the Economic and Environmental Sustainability of Dairy Enterprises (Rev. NC-1119), NC 1195 Enhancing nitrogen utilization in corn based cropping systems to increase yield, improve profitability and minimize environmental impacts (NC1032/218), NCCC 208 and 308 Nutrition and Management of Feedlot Cattle to Optimize Performance, Carcass Value and Environmental Compatibility (NCT192), NE 1024 Whole Farm Dairy and Beef Systems for Environmental Quality, NE 132, Environmental and Economic Impacts of Nutrient Management on Dairy Forage Systems and W 503, Economic, Environmental, Genetic, and Nutritional Aspects of Grass-Fed Beef. These projects are largely focused either on specific regions, species, or environmental media and therefore would be largely complementary and do not represent duplication to the proposed project. One of the strengths of the terminating project, S-1032, for which this proposed project is a replacement is that it has established a track record of a multi-disciplinary, integrated project.
Figure 2 represents an overview of the project scope in logic model format. See attached.
Objectives
-
Engage collaborators from the needed broad range of disciplines, institutions, and stakeholder groups to catalyze conceptual and quantitative synthesis, collaboration, and data sharing
-
Facilitate organization, synthesis, and integration of component-based research findings and supporting data and
-
Discover (or reveal), substantiate, and interpret the broader impacts of component-level modifications to animal-production systems.
Methods
OBJECTIVE 1. Engage collaborators from the needed broad range of disciplines, institutions, and stakeholder groups to catalyze conceptual and quantitative synthesis, collaboration, and data sharing. The overarching goal of objective 1 is to expand the scope of expertise represented by the participants and facilitate interaction among the network of participants resulting in creative dialogue and attainment of the project mission. Often the cost of travel and the time required for travel prohibits meaningful interaction. The rationale for this objective is that in order for meaningful collaboration to occur, a mechanism for low-barrier interaction among scientists with very different backgrounds, cultures, and constraints must be established. The underlying hypothesis is that wicked problems (ill-informed, where the information is confusing, many clients and decision makers have conflicting values, and ramifications in the whole system are thoroughly confusing) (Churchman, 1967), such as the accelerating demand for global protein production under the constraint of finite natural resources, necessitates trans-disciplinary thought processes and collaborations. Task 1.i. Provide opportunities for regular interaction among project participants and exposure to new ideas and thought provocateurs through a no-cost webinar series. (State participation: MI, CA, TX) We will establish a Virtual Dialog Network (VDN) that assembles membership quarterly throughout the project period and exposes project participants to work conducted by the membership as well as ideas and work from scientists that are not part of the formal membership as a way of establishing collaborative connections and catalyzing creative thought. The intended outcome of these meetings is a better understanding of how each others work might fit together to promote professional development and participant/collaborator recruitment. Information transfer from these meetings may serve to fill knowledge gaps within individual members programs and catalyze formation of additional research topics. Each virtual meeting will have a specific focus and topic leader/facilitator. Topics and leaders will be determined at the onset of each project year. Peer interaction will identify presenters from outside the project team that can address critical knowledge areas by (a) introducing new concepts, analytical methods, and thought processes, (b) identifying relevant applications of those concepts, methods, and processes, and (c) equipping participants to use those new competencies to overcome barriers to problem resolution. Additionally, the annual face-to-face meeting time will be set aside to plan the upcoming years quarterly VDN and identify potential collaborative opportunities. The chair-elect of the committee will have leadership responsibility for the VDN. Task 1.ii. Develop and implement an annual meeting format to foster substantive collaboration. (State participation: MI, CA, TX) Annual face-to-face meeting time will be utilized to further the progress on project deliverables. The intended outcome of the annual face-to-face meeting is progressive identification of the interactions between accelerated animal protein production and TBL metrics. The meeting focus will be dedicated to a structured analysis and discussion of the dynamic nodes necessary to properly define and describe the system and subsystems involved. Invited presentations that address remaining gaps in understanding the complex dynamics of sustainable animal protein production will comprise a portion of the annual meeting time. The annual business meeting time will entail discussion about a strategic location for the next meeting that will further the project mission (meet in conjunction with a related meeting; identify a location that facilitates interaction with scientists of specific skill sets; or provide some other means of catalyst for project success) and selection of new project officers. Evaluation of success. Measures of success include the number of new concepts introduced each year as well as the scholarly products that incorporate those new concepts developed by project participant, project participants groups, thought provocateurs engaged during the project period (e. g., proposals, working papers, range of data types and volume of data in databases), and familiarity of project participants with those new concepts. Each metric will be tracked annually through annual participant reports and reported in the annual project report. OBJECTIVE 2. Facilitate organization, synthesis, and integration of component-based research findings and supporting data. The overarching goal for objective 2 is to develop trans-disciplinary research teams that are highly collaborative but also heterogeneous in that different team members may work together at different times. The underlying hypothesis is that the transient nature of the collaborations will result in some teams working in parallel on a component or relational aspect of animal protein production while other teams work sequentially (i.e. one teams product being another teams tool or starting point). Individuals will assemble to form necessary teams and participation into these teams will ebb and flow, as needed, in order to make best use of the expertise needed for specific, discrete tasks. The rationale for this objective is that only through a collaborative network that facilitates the sharing of data, inside and outside of the project participant network, will progress towards resolving wicked problems be achieved. Project leadership supports the synthesis and sharing of component-based work and supporting data via a publicly accessible database. Task 2.i. Design, and find a host for, a publicly accessible database for sharing peer-reviewed, published project data that facilitates integration of system components. (State participation: MI, CA, TX) Sharing ideas and data is at the heart of collaborative research and development and this project team will develop a database design to support this collaboration. The project team will select parameters and appropriate units for various animal production components or unit operations such that other team participants can subsequently use or add to those results. Identifying the parameters and units will be a primary objective of the first annual meeting. The database will be publically accessible and searchable; records will be downloadable in usable electronic form. At present, members of the project team are discussing the possibility of collaborating with National Center for Ecological Analysis and Synthesis (NCEAS) for hosting the database, either as a part of or in parallel with the Knowledge Network for Biocomplexity (KNB; http://knb.ecoinformatics.org) as they are currently equipped for the data-sharing enterprise. It is expected that this database will be accessible through a project web site. We expect that this database will become a valuable resource for collaborative efforts. Peer-reviewed data from project participants will populate the database voluntarily, precisely because of the potential for future involvement in such projects. Quality control and publication rights will be managed by the individual project participants by contributing peer-reviewed data only after appropriate publication. The trend towards multi-disciplinary research by federal agencies has driven collaborative proposals for several years. Multistate research committee S-1032 has already served as a vehicle for submitting two large proposals to NSF in 2012, and because dedicated, external funding is so critical to the success of what is proposed herein future proposals will build on the constructive peer reviews that each proposal received. Task 2.ii. Design and find a host for a database accessible to project participants describing ongoing projects and data currently being collected. Another tool to enhance collaboration among participants will be a database, accessible internally to participants, that describes specific interests, special capabilities, and current projects of each participant. The types of information expected to be included here are specific research topics each participant is interested in, equipment, measurement systems, or other resources that would be useful in specific circumstances; and ongoing projects, including current data collection (parameters, functional units). This information will make it easier to find the expertise and interest needed for specific projects and will become valuable for identification of potential collaborators on competitive, multi-disciplinary research proposals. This password-protected database will be hosted on the same server that supports the project website. Project participants will update their information annually, or more frequently if appropriate. Evaluation of value and use of databases. With input from committee membership and as a consequence of the annual station reports, the project chair will track the range of data types and the number of entries in the databases as a means of assessment and include the results in the annual report. OBJECTIVE 3. Discover, substantiate, and interpret the broader impacts of component-level modifications to animal-production systems. (State participation: MI, CA, TX) The legacy value of S-1032 and its predecessors has been to complete reductionist research without formal attention to system structure and the role of human agency. To address this, we have started, through S-1000, to develop an appreciation for (a) the variety of under-represented (or unrepresented) research disciplines having specific relevance to livestock and poultry production systems and (b) the variety of analytic and synthetic tools that those disciplines make available to us to enrich our understanding of sustainability and what it means for the systems that interest us. Over the past five years, especially, we have been introduced to life-cycle analysis (LCA), thermodynamic measures like emergy and exergy, ecological footprint analysis, network and information theory, game theory, and various modeling perspectives, all of which seem likely to give quantitative meaning to the term sustainability as it applies to modern livestock and poultry production. But we are at a pivotal time when additional members in those under-represented disciplines must be engaged and given a formal stake in S-1032 to help us formalize our mental models, devise meaningful and measurable conceptions of sustainable livestock and poultry production, select and apply the appropriate quantitative, system-level modeling tools, and evaluate the system-level implications of information gained from our ongoing, reductionist research. Objective 3, the description of which is necessarily technical (and may be tedious for some readers), advances our overall understanding of animal production systems while providing means to evaluate alternative management practices more thoroughly and identify incremental progress toward TBL sustainability. The National Academy of Sciences (NAS, 2010) stated that an agricultural system may be considered sustainable if and only if it: a) satisfies human needs for food, feed, fiber, and biofuels; b) enhances environmental quality and the natural-resource base on which it depends; c) sustains the economic viability of agricultural communities; and d) enhances the quality of life for farmers, farm workers, and society as a whole. Figure 1, presented earlier, illustrates the mutual dependence of those four cardinal attributes of a sustainable agricultural system, represented as state variables (e.g. economic or environmental sustainability, demand for animal protein), through a wide variety of interconnected processes, feedback pathways, and intermediate variables. All of these variables, taken together, represent the complex, highly integrated, social, economic, and environmental system within which animal production occurs. Figure 1 is available as an attachment. Because feedbacks, event cascades, mutual dependences, and delayed effects are so pervasive throughout in ways that are strongly dynamic and nonlinear changes at the level of individual processes (i.e. within a confined a section of the CLD) may impact the system in ways that are not reliably predicted by the kind of linear conceptualizations that underlie our typical, reductionist research projects resulting in both intended and unintended consequences. In short, component-level modifications of the animal-production system are not expected to result in easily predictable outcomes or trajectories for the system as a whole. Just as increasing the fuel efficiency of 19th-century British technologies accelerated coal consumption (Jevons, 1865), we should expect that management or technology changes at the level of individual animal-production processes may generate strange, counter intuitive, and perhaps even perverse behaviors in other processes or in the system as a whole. We propose, therefore, to develop, quantify, and validate a dynamic, simulation-modeling framework, along the conceptual lines of Figure 1, which will help researchers and collaborating stakeholders to project the system-level impacts of management or technology changes at the process level (with a segmented section of the CLD). Task 3.i. Progressively refine a modeling framework, from the conceptual to the quantitative, that describes the relationships between (a) increasing demand for animal protein produced in the United States and (b) the social, economic, and environmental subsystems that sustain animal-protein production for the long term (NAS, 2010, p. 25, Box 1-6; p. 24, figure 1-1). In its initial form, our conceptual modeling framework is a CLD that consists of (a) variables - nodes or sections within the CLD - of uneven specificity and quantitative meaning and (b) causal linkages - arrows and polarities - of uneven clarity and scientific justification. Over the five-year duration of the project, participants will progressively refine the CLD by modifying, replacing, removing, adding, and documenting variable nodes and causality arrows, replacing polarities with mathematical relationships when appropriate. A simple exposition of Task 3.i. might start with a simple CLD (e.g. figure 3), an arbitrarily selected subset of the diagram in figure 1. Figure 3 attached. In this case, we have (a) four operational nodes or variables (blue) and (b) a single state-variable node (red) that represents, for example, a sustainability or system-performance index computed from several of the values of the operational variables. Developing the process-level understanding by which ad hoc judgments of polarities are transformed into mechanistic descriptions of causality is the ongoing, reductionist task in which S-1032s membership has been historically, productively, and meritoriously engaged. For the purposes of this 5-year proposal, we assume that such work will continue. In fact, for our project to be successful, it must continue. In figure 3, the four operational sections of the system (nodes) might be low-level variables whose interactions, represented by arrows, are more or less mechanical; that is, the interactions may be represented by the kinds of mathematical relationships that are the foundation of any members research program, both historical and current (e.g. air emission mitigation strategies, environmental nutrition, manure treatment processes, soil properties). Some of the causal interactions are unidirectional (e. g., nodes 1 and 3), meaning that changes in the originating node provide a cause for the changes in the terminal node measured as the effect. Some of the interactions, however, are bidirectional, or nonlinear (e. g., nodes 2 and 4), in that one process reflects a partial causality by which changes in node 2 elicits behavior in node 4, and a different process reflects another partial causality by which changes in node 4 elicits behavior in node 2. Further, non-linearity may arise indirectly (e. g., the loop involving nodes 3, 4, and SV) through intermediate nodes. Thus the subsystem consists of both unidirectional and mutual relationships that must be moved from the conceptual/qualitative to the concrete/quantitative in order that the subsystem, as a whole, be usefully operationalized. The ability to describe and quantify these relationships requires the basic reductionist research findings that members produce in their primary research programs. The principal value-adding thrust of this proposal, however, goes beyond the reductionist task and views the committees future membership as interpreters, distillers, integrators, and synthesizers of that research, which involves not only (a) moving specific ideas of causality from the conceptual to the concrete but also (b) progressively developing and refining the conceptual model as our understanding of and appreciation for causality matures in breadth and depth within the broader system domain. This synthetic task, then, involves a more deliberate and strategic engagement with individuals providing a needed and complimentary skill set in our current membership (e. g., soil and crops sciences, statistics, systems analysis, integrated modeling) and a new roster of collaborators in more synthesis-oriented disciplines such as ecosystem science, network analysis, and the social sciences, disciplines in which those collaborators will be able to help us integrate the subsystems and associated TBL considerations into our modeling framework. Activities for Task 3.i. will originate from the committees final, annual meeting as S-1032, scheduled for August 13-15 2013 in Washington, D. C. For that meeting, we have used the funds from our 2011 ESCOP national award to engage the services of Systems Thinking Collaborative to help us (a) refine or replace our sustainability CLD for the next five-year cycle, (b) specify the new analytic and synthetic expertise that will help us accomplish Objective 3 tasks, (c) identify structural and strategic means of improving committee operations, and (d) develop recruiting, retention, and reproduction strategies to sustain our committees vitality for the longer term. Task 3.ii. Learn and adapt analytic and synthetic approaches from new bodies of knowledge to integrate process-based research findings into the system framework developed in Task 3.i. For example, Nnetwork theory and graph theory provides a set of tools by which we can might record, document, and measure (see Task 3.iii) our progress in moving the conceptual ideas implied by figures 1 and 3 into the quantitative domain. Figure 4 is known as a connectivity matrix, which maps the causal relationships in figure 2 into a two-dimensional, square array that contains the same information. Figure 4 (see attached). Directional connectivity matrix C(I,j) representing the causal-loop diagram in figure 3. The row and column labels in that connectivity matrix, are the variable names associated with the five nodes in figure 3. Thus each cell C(i,j) contains information about the direct causality, if it exists, exerted by variable i on variable j. If there is no causality (no arrow) directly linking nodes i and j, then the cell contains a null or zero. If there is a causality from i to j, the cell contains either its polarity (if known) or, as our process-oriented research progresses, some quantitative relationship that describes the causality. There may be several different forms of a generalized connectivity matrix that map important information from our CLDs. For example, an adjacency matrix has a number of useful properties that can expose network structure even if the system itself cannot yet be numerically described and may well serve purposes in the early stages of our modeling framework, while we are mainly interested in exposing network structure and relationships. In an adjacency matric, the cell contents are either 0 (no directional causality from node i to node j) or 1 (a directional causality exists). As our ongoing, process-oriented research allows us to define more fully (i.e., mathematically) the causal mechanisms existing between adjacent nodes, a Jacobian matrix may be useful. In a Jacobian matrix cell (i,j) contains the partial derivative Vj/Vi, which represents the marginal sensitivity of system variable j to changes in system variable i. This more advanced, operational tool is conducive to system simulations. For variable pairs that involve easily related quantities (e. g., feed composition and manure output, or commodity sales and sales income), our existing membership is well qualified to derive the Jacobian expressions. For variable pairs whose mutual relationships are less obvious - for example, a variable describing energy availability and a variable representing some sort of social currency or demographic gradient - we will need close collaboration with new partners to guide us (a) in devising the appropriate Jacobian terms and/or (b) in decomposing the relationships until the chain of causality is clearer and more compelling. Put another way. We will need new collaborations to help us describe the impact of one variable on another and/or help us to expand that part of the CLD to include additional nodes and until the cause and effect relationships are clearer. It is apparent that the specialized capabilities of both our legacy membership and our newly engaged collaborators will be needed to achieve Task 3.1. The whole-system, somewhat ad hoc CLD in Figure 1 contains many causal linkages between incommensurable variables, that is, variables that operate in completely different domains of reality and whose pairwise relationships are therefore problematic to describe in intuitive (to say nothing of quantitative!) terms. To enable long-term development of a full Jacobian connectivity matrix, we will likely need to decompose the whole-system CLD into subsystems whose causalities link commensurable variables. One way of viewing this decomposition is shown in Figure 5 (see attached). Finally, the connectivity matrix concept may also be useful for documenting the causal linkages in peer-reviewed research. In this application, the cells in the connectivity matrix contain string variables analogous to the records in a bibliographic database. As our mechanistic understanding of the relationships between adjacent nodes is progressively developed and refined by process-level research that is, as we fill the cells of the various forms of connectivity matrices we will document those refinements by populating a bibliographic form of the matrix with the applicable citations of peer-reviewed research. To summarize Task 3.ii., the primary synthesis work during the next five years will be the systematic refinement of our whole-system CLD, transforming it from a conceptual network of nodes and linkages into an increasingly organized, quantitative, and well documented network. The CLD will be mapped to a library of closely related connectivity matrices, commonly available to all participants that contain the polarities, mechanistic descriptions, and bibliographic documentation of the relationships between adjacent nodes. Task 3.iii. Build a dynamic, system-level, modular, simulation-modeling framework to project the concurrent flows of mass, energy, money, and various other fundamental currencies within our TBL-sustainability domain. This Task will necessarily involve intelligent trial-and-error with a variety of modeling environments, beginning with highly simplified platforms (e. g., Excel, Stella, Verisim) and progressing to more powerful, sophisticated platforms (e. g., Matlab/Simulink, GAMS) as our progress justifies it. This modeling framework, and the associated module libraries, will be designed to accommodate disparate model types across the scientific disciplines represented by our committee. For example, in the realm of animal sciences we will build or adapt, as appropriate functionally similar modules that embody National Research Council (NRC) and related algorithms to estimate feed intake, conversion, and excretion dynamics as modified by dietary, management, housing, and meteorological factors. We will also build/adapt engineering-based modules to simulate the performance of manure- and/or waste-management processes (e. g., anaerobic digesters, holding ponds, lagoons) and environmental-control systems (e. g., heating, ventilation, evaporative cooling). These, along with other modules from other disciplines from economics to sociology to soil and atmospheric sciences, will be built or adapted in accordance with an increasingly formalized, quantitatively unified framework that ensures the modules can be used together in arbitrarily large, complex simulation models. In addition, where sophisticated, modern modeling environments already exist (e. g., GAMS for economic optimization modeling, ArcGIS for spatial modeling), we will seek to design and build into our modeling framework or adapt, as appropriate tools become known to us a suite of tools by which our system models can call other modeling environments as subroutines. Deliverables from Task 3.iii. may be conveniently viewed as a growing, increasingly sophisticated and powerful library of sub-models of raw materials, feedstuff production, animal production, waste management, natural resources, market dynamics, and social dynamics that represent the quantitative relationships and networks in our governing, evolving CLD. Task 3.iv. Perform dynamic simulation of subsystems to evaluate effects of newly specified causal linkages, research findings, and policy options. As project participants contribute to the enrichment of the conceptual modeling framework with refined variable sets and mathematically specified causal relationships defined in their own reductionist, process-oriented research programs, we will be able to build, test, and evaluate functioning models of dynamic systems of increasing scale and sophistication. The robustness of this Task will depend on the progress made in Task 3.iii. Interested participants will collaborate to prepare proposals to funding institutions for dynamic simulation of subsystems, including e. g. NSFs Research Collaboration Network (RCN) and Sustainability Research Network (SRN) programs and USDAs National Needs Fellowships (NNF) program. At our annual meetings and during mid-year teleconferences, we will identify research gaps within our modeling framework, available funding sources to underwrite the work, and teams of collaborators to write responsive grant proposals. Evaluation. Track the richness and quality of the CLD (extent and depth to which the various CLD sections are described with respect to conceptual diversity, causal interconnectedness, documentation of causal linkages, quantification of causal relationships) and identify new mathematical relationships within the CLD.Measurement of Progress and Results
Outputs
- Improve professional development at our annual face-to-face and quarterly virtual meetings
- Establish a collaborative network from which members may select to work on research proposal development and submission to federal agencies to expand the network of expertise engaged in the discussion and framework development in order to tackle the complex problems.
- Publish white paper(s) and journal articles to amalgamate and summarize group findings to describe various measurement metrics, data collection needs to accomplish analysis, etc.
- Development of curriculum for inclusion in college courses.
- Outreach activities: articles in popular press/trade association outlets and mini briefing documents
Outcomes or Projected Impacts
- Standard reporting measures and units (media, substances, units) to quantify impacts of animal production and effectiveness of management alternatives.
- Quantitative tools to evaluate pollution modifications (increases or decreases)
- Verified systems-level model(s) to be used to evaluate the ecological impacts of changes in the animal agriculture industry. These models and the research they will inspire will lead to reduced inputs to food animal production, greater reuse and recycling of manure resources and improved environmental quality.
- Greater visibility of the expertise within the group resulting in enhanced grantsmanship.
Milestones
(1):ior to project start of this new project (last meeting of current S-1032 project; August 2013) specific subcommittees will be formed and assigned to move forward the overall objective of establishing a framework describing the complex dynamics of sustaining agricultural production for the foreseeable future. The objective will be to formulate specific questions concerning the various social, economic, and environmental interactions necessary to drive the addition of researchers representing other disciplines necessary to properly formulate the relationships between the various system sections of the CLD. Subcommittees established at this point will interact regularly through the year to formulate conceptual models describing the various economic, environmental, and social interactions relevant for sustaining livestock and poultry production in an environment of global economic and political instability, potential climate change, and possible environmental stresses (i.e. interaction of the CLD sections). Progress from these subcommittees will be shared through the VDN. Critical areas where project members are not working will be identified and individuals who may assist with these deficiencies will be recruited to participate in the group and the VDN. Prior to the year one annual meeting, subcommittee models will be disseminated to the membership for consideration and formal adoption. This effort will take place largely through electronic communications (i.e. virtual meeting spaces)(2):e approach for Year 2 is to use the outputs from Year 1 to formulate a series of conceptual models, including consistent representation using CLDs, stocks and flows, and life cycle analysis (LCA), that identify specific researchable topics matching investigators existing research programs and tying to the broader questions of how the various aspects of the livestock and poultry production systems interact. At the annual meeting (end of year 1), these models will be adopted, although not finalized, as a framework for the multistate research committee to move forward. The annual meeting at the end of year one will serve as a forum to formalize the cooperative framework for evaluating factors affecting the sustainability of livestock and poultry production. Included will be workshop sessions on " the mechanisms of systems dynamics conceptualization and " considerations for writing successful joint proposals incorporating researchable systems dynamics topics.
(3):e annual meeting at the end of Year 2 will serve as a forum to formalize the cooperative framework for evaluating factors affecting the sustainability of livestock and poultry production (identification of how to proceed with putting numerical descriptors into the CLD). Included in the annual meeting will be workshop sessions on "the mechanisms of systems dynamics conceptualization, "life cycle analysis of the system and components, and" considerations on writing successful joint proposals incorporating researchable systems dynamics topics. Committee members will integrate findings into the identified systems framework and tools. This will be documented in the station reports that will begin to reflect the systems integration approach as investigators discuss their findings within the dynamics framework identified. An expectation is that additional research topics will be identified with new members added to address the evolving understanding of the various relationships. Year 3 activities will include continuation of the virtual dialogs already established with emphasis on integrating individual research results into the dynamics framework established during year one. Further emphasis will be on writing collaborative proposals to answer the questions being identified during the continuing dialog.
(4):e objective will be to move beyond conceptual systems models to descriptive numerical interactions (i.e. populating the connectivity matrix, Figure 3). Progress made will b
Projected Participation
View Appendix E: ParticipationOutreach Plan
Education Outreach (the extension of the information gained in this project to the greater scientific and lay communities) will be conducted throughout the project period. Some outreach will be passive (the user of the information finds or stumbles on it through web searching. Other outreach will be active through planned symposium, presentations, participation in webinars, etc. As an example, the quarterly virtual interactions (VDN; Objective 1, Task I) will be available for participation by individuals outside the project team. Members of the project team will be tasked with writing up presentations. Summation of information delivered/exchanged will be maintained on the group website under a heading of current beliefs/findings section. Members of the group will selectively identify information to share with interested clientele groups within their states to inform individuals/groups of the research activities, findings, and value of science. These outreach mechanisms may serve as informal recruitment events to improve the strength and validity of network elements. Results of research findings will be presented at various international and national professional societies, published in appropriate journals, technical proceedings, and annual reports. Progress with Objectives 2 (database development and accessibility) and 3 (modeling framework) lend themselves to establishment of online education materials or courses harnessing the amplified value of individuals of diverse backgrounds focused in one joint area of research to address critical questions for societal needs. Some outreach activities will be specifically targeted at other professionals (academic or private industry) working in areas similar to the work of the group or having cross-over effects (professional society meeting presentations or short courses). These activities will increase awareness and provide information for skill-building within these groups. Outreach programs will enhance further participation and potential collaboration with the Research Group. Outreach to individuals in stakeholder groups will improve the information exchange necessary to complete Objective 3. We believe business, industry, government, and the education community must work together as partners to improve the educational opportunities for all students in our region. One of the proposed objectives for this project is focused on establishing a communication system for researchers and stakeholders and enhancing abilities to share data. Advertising the availability of the online database website created as part of our work may result in self-identification of individuals who would add value to the group. Outreach accomplishments as both group and individual levels will be shared during annual project meetings.
Organization/Governance
Chair: The chair of the committee is responsible for organizing the meeting agenda, conducting the meeting, and assuring that task assignments are completed. The chair is elected for a one year term. Chairs are eligible for re-election. Chair-elect: The chair-elect normally succeeds the chair, and is expected to support the chair by carrying out duties assigned by the chair. The chair-elect serves as the chair in the absence of the elected chair. The chair-elect is elected for one year. The chair-elect is eligible for re-election. The chair-elect is responsible for management of the virtual dialog network (VDN) series. Secretary: The secretary is responsible for the distribution of documents prior to the meeting. The secretary is also responsible for keeping records on decisions made at meetings (a.k.a. keeping the minutes), maintaining an updated roster of participants (as a list server), and assisting in the preparation of the accomplishments report (i.e., the SAES-422). The secretary normally succeeds the chair-elect. Secretaries are eligible for re-election. Members: In addition to carrying out the agreed research collaboration, research coordination, information exchange, or advisory activities, project members are responsible for reporting progress, contributing to the ongoing progress of the activity, and communicating their accomplishments to the committee's members and their respective employing institutions.
Literature Cited
Churchman, C. W. 1967. Free for All. Management Science 14(4):B141-B146.
Costanza, R., R. D'Arge, R. De Groot, S. Farber, M. Grasso, B. Hannon, K. Limburg, S. Naeem, R. O'Neill, J. Paruelo, R. Raskin, P. Sutton and M. van den Belt. 1997. The value of the world's ecosystem services and natural capital. Nature 387253-260.
Elkington, J. 1997. Cannibals with forks: the triple bottom line of 21st century business. Capstone, Oxford, 402 pp.
FAO. 2008. The State of Food and Agriculture 2008. Rome.
Fath, B. D., U. M. Scharler, R. E. Ulanowicz and B. Hannon. 2007. Ecological network analysis: network construction. Ecological Modelling 208(1): 49-49-55.
Fiscus , D. A. 2009. Comparative network analysis toward characterization of systemic organization for human-environmental sustainability. Ecological Modelling 220(22): 3123-3123-3132.
Goerner, S. J., B. Lietaer and R. E. Ulanowicz. 2009. Quantifying economic sustainability: Implications for free-enterprise theory, policy and practice. Ecological Economics 69(1): 76-81.
Gibbons, R. 1992. Game Theory for Applied Economists. Princeton, NJ: Princeton University Press. 267 pp.
Jevons, W. S. 1865. The Coal Question: An Inquiry Concerning the Progress of the Nation, and the Probable Exhaustion of Our Coal-Mines. London: Macmillan & Co. 383 pp.
Robinson, R. A. and W. J. Sutherland. 2002. Post-war changes in arable farming and biodiversity in Great Britain. Journal of Applied Ecology 39(1): 157-176.
Rockstrom, J. 2009. A safe operating space for humanity. Nature 461(7263):472-475.
Gibbons, R. 1992. Game Theory for Applied Economists. Princeton, NJ: Princeton University Press. 267 pp.
Schumacker, R. E., and R. G. Lomax. 2010. A Beginners Guide to Structural Equation Modeling (3rd ed.). New York: Routledge. 510 pp.
Snyder, D. and E. L. Kick. 1979. Structural Position in the World System and Economic Growth, 1955-1970: A Multiple-Network Analysis of Transnational Interactions. American Journal of Sociology 84(5): pp. 1096-1126.
Sterman, J. D. 2000. Business Dynamics: Systems Thinking and Modeling for a Complex World. New York: McGraw-Hill, Inc. 1008 pp.
Ulanowicz, R. E., S. J. Goerner, B. Lietaer and R. Gomez. 2009. Quantifying sustainability: Resilience, efficiency and the return of information theory. Ecological Complexity 6(1): 27-36.
Zering, K.D., T. J. Centner, D. Meyer, G. L. Newton, J. M. Sweeten, and S. Woodruff. 2012. Water and Land Issues Associated with Animal Agriculture: A U.S. Perspective. Council for Agricultural Science and Technology, Issue Paper 50.